ABSTRACT
Pituitary adenomas have been historically managed on a multidisciplinary level with surgery, medical therapy, and radiotherapy to control symptoms secondary to mass-effects and hypersecretion of hormones. While transsphenoidal surgery represents the standard initial approach in the majority of cases, radiotherapy is a valuable and effective treatment option for recurrent adenomas, or lesions not amenable to surgery or medical therapy. Following radiotherapy, tumor growth control (over 90% in most series), plus the normalization of hormones, occurs in a large proportion of treated patients, independent of tumor subtype. Over the last decades, radiotherapy technological advances have allowed the reduction of dose to uninvolved brain while maintaining an effective therapeutic dose to the tumor. This has generated debate on the superiority of some radiotherapy techniques over others. The clinical efficacy of conventionally-fractionated treatment (25 to 30 fractions delivered over 5 to 6 weeks), in the form of 3D-conformal radiotherapy (CRT) or intensity-modulated radiotherapy (IMRT) and the more refined “stereotactic” – highly conformal - fractionated radiotherapy (SFRT), can be compared to that provided by “radio-surgical” (SRS) techniques of irradiation (where the tumor is treated with single high dose of radiation). Due to the lack of randomized control trials addressing this issue, the evidence provided in retrospective studies of different radiotherapy technologies is critically reviewed in this chapter.
INTRODUCTION
Pituitary adenomas are mostly benign tumors and comprise about 10% of all intracranial tumors [1, 2]. Radiotherapy has an important and long-established role as part of the multi-disciplinary management of both non-functioning and functioning adenomas. There has been a steady evolution in radiotherapy technologies since radiotherapy was first used to treat pituitary adenomas more than 100 years ago [3]. Despite decades of clinical experience, there remains a paucity of randomized clinical trials to enable a robust evidence-based approach to the optimal use of radiotherapy. This is to some extent compensated for by the large number of non-randomized largely retrospective case series which provide evidence on relevant clinical outcomes and toxicities associated with pituitary radiotherapy. Nevertheless, given the nature of the available data, there continue to be areas of controversy regarding the use of particular radiotherapy modalities. We review the available published data on modern radiotherapy techniques for the treatment of pituitary adenomas to provide a rational basis for the selection of radiotherapy technologies.
RATIONALE FOR PITUITARY RADIOTHERAPY
Traditional practice had been to use post-operative radiotherapy for all patients with a residual non-functioning pituitary adenoma after surgical resection, as it was considered that otherwise most would subsequently progress [4, 5]. With improvements in surgical techniques, and the development of magnetic resonance imaging (MRI), post-operative radiotherapy is no longer routinely used, even in the presence of residual tumor. The use of post-operative pituitary radiotherapy is now based on a risk assessment. In patients with non-functioning adenomas, radiotherapy is generally withheld until the time of progression, unless there are concerns of significant threat to function (vision) with tumor progression, or the histology raises concerns of earlier recurrence risk (e.g., atypical features, silent corticotroph adenoma). When radiotherapy is used for patients with progressive non-functioning adenomas, tumor control is achieved in over 90% of patients at 10 years, and in 85-92% at 20 years [5-13].
In patients with functioning adenomas, radiotherapy is used when surgery fails to achieve hormone normalization and/or when medical treatment is insufficient to control hormone secretion or is not considered appropriate, often due to toxicities. Hormone levels decline slowly following radiotherapy, consequently normalization may take from months to years to achieve. The time required to achieve hormone normalization is primarily related to the pre-treatment hormone levels. Nevertheless, despite this temporal delay, the majority of patients will eventually achieve normalization of excess pituitary hormone secretion following radiotherapy [14].
CURRENT TECHNIQUES OF PITUITARY RADIOTHERAPY
The principal aim of pituitary radiotherapy techniques has always been to deliver an effective treatment dose to the target tumor volume while at the same time minimizing the radiation dose delivered to surrounding normal tissues, thereby minimizing the risk of normal tissue damage. Improved radiotherapy treatment precision, with the use of the modern radiotherapy techniques described in this chapter, relies on the increased accuracy in tumor volume delineation achieved by using modern MRI imaging technology. Over the last twenty years there have been a number of developments in techniques for pituitary radiotherapy which have largely amounted to refinements of existing technologies. However, the overall success of modern high precision pituitary radiotherapy techniques is largely a function of the quality of a treatment center’s infrastructure and its expertise and accuracy in identifying the target tumor volume, rather than of the particular radiotherapy technique that is used to deliver treatment.
3D-Conformal RT
Until the last decade, the standard of care for pituitary radiotherapy was three-dimensional (3D) conformal radiotherapy (CRT). CRT uses pre-treatment computed tomography (CT) and MRI imaging for computerized 3D radiotherapy treatment planning. CRT treatment is planned and delivered using a non-invasive method of patient immobilization. The tumor is visualized on unenhanced magnetic resonance imaging co-registered with planning computed tomography (CT). The treatment target is delineated on the MRI scan (in the three orthogonal planes), while radiotherapy dosimetry is calculated using the CT scan data.
The treatment target comprises the visible residual tumor and also accounts for any pre-operative extension of disease whilst sparing the optic chiasm where possible after decompression. An isotropic margin of 5-10 mm is added to account for areas of uncertainty in volume delineation, the transsphenoidal surgical route and any set-up variation. The whole pre-operative extent of the tumor is not included within the treatment volume as debulking of large, and particularly cranially extending tumors, often leads to the return of normal anatomical structures to their pre-morbid positions with no residual tumor present. On the other hand, tumors are frequently not removed from the walls of the cavernous sinus, particularly if the sinus is involved, and so the lateral extent of the radiotherapy target does not tend to alter with surgery. The resulting volume outlined on the treatment planning system therefore encompasses both the visible tumor and also any regions of presumed residual tumor. Normal tissue structures adjacent to the pituitary, such as the optic chiasm and optic nerves, the brain stem and the hypothalamus, may also be outlined to aid in treatment planning, and also to enable the calculation and recording of normal tissue
dosimetry, although with conventional fractionated radiotherapy all the structures are treated to below the limits of radiation tolerance in terms of structural damage.
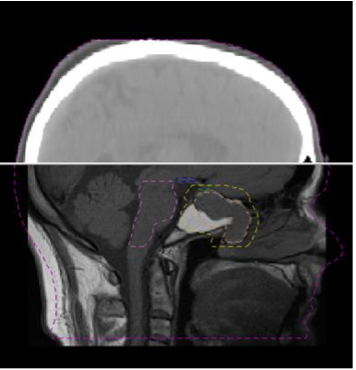
Figure 1. CT-MRI co-registration for planning purposes.
CT imaging for CRT planning is performed with the patient lying in the radiotherapy treatment position within the immobilization system and co-registered with the MRI (Figure 1) 3D computerized radiotherapy planning is followed by robust quality assurance (QA) procedures to ensure the accuracy of the whole process both before and during treatment. The planning system defines the number, shape, and orientation of radiation beams to achieve uniform dose coverage of the target volume with the lowest possible dose to the surrounding normal tissues. As the dose to the tumor is below the radiation tolerance dose of the surrounding normal tissue structures, no specific measures are generally needed, or taken, during treatment planning to avoid the optic apparatus, hypothalamus, and brain stem. In any case, for many patients requiring pituitary irradiation, some of these entire normal structures lie within, or in close proximity to, the target volume and cannot be avoided without compromising the efficacy of treatment.
Localized irradiation is achieved using treatment in multiple beams each shaped to conform to the shape of the tumor using a multileaf collimator (MLC). Traditionally, beam arrangements used for CRT consisted of three fixed beams (an antero-superior beam and two lateral beams) (Figure 2).
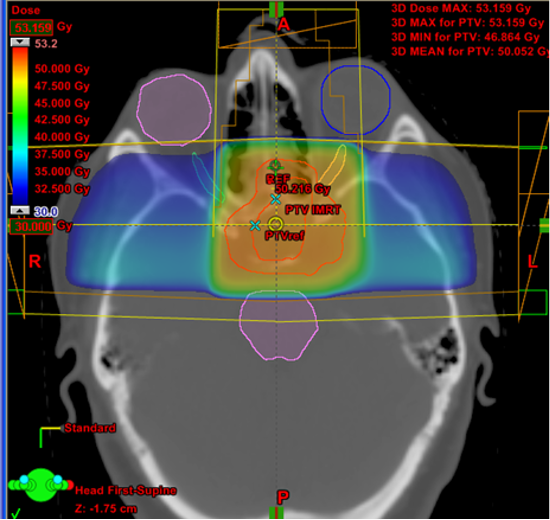
Figure 2. Example of beam arrangement and dose distribution in a traditional CRT plan (one antero-superior beam and two lateral beams).
Intensity-Modulated RT
Techniques for varying the radiation dose intensity across a beam, by moving MLC leaves into the beam path, are now standard and are collectively referred to as intensity modulated radiotherapy (IMRT). IMRT is a form of 3D CRT which can spare critical structures, especially within a concave PTV. Although IMRT offers no significant advantage in comparison with CRT for target volume dose coverage [
], its improved conformality can allow for reduced radiation dose delivery to adjacent normal tissues. This can be of particular use in tumor with suprasellar extension, where the dose delivered to the medial temporal lobes can reduced. The technique of arcing IMRT (described as VMAT or RapidArc) offers a fast way of delivering complex IMRT and is increasingly used as an alternative to fixed-field techniques (Figure 3).
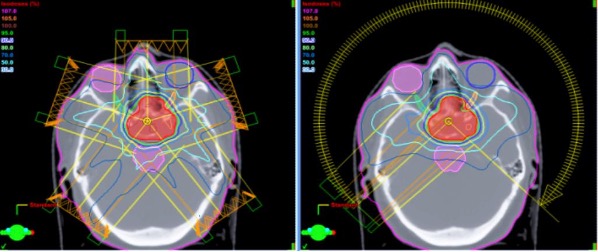
Figure 3. Example of beam arrangement and dose distribution in a static field IMRT plan (left) and in a VMAT plan (right) for the same patient. Note the better conformality of the high radiation dose region to the target volume in comparison with the CRT plan in Figure 2.
Patient immobilization and the imaging required for target volume definition are no different for IMRT treatment than for CRT as described above. Similarly, there are robust QA procedures to ensure the accuracy of IMRT treatment planning and delivery.
Stereotactic Radiotherapy Techniques
The term “stereotactic” is derived from long-established neurosurgical techniques, and denotes a method of determining the position of a lesion within the brain using an external 3D co-ordinate system based on a method of immobilization, usually an invasive neurosurgical stereotactic head frame [18-20]. Stereotactic radiotherapy originally referred to radiotherapy treatment delivered to an intracranial target lesion that was located by stereotactic means in a patient immobilized in a neurosurgical stereotactic head frame.
Stereotactic radiotherapy was first delivered with a multiheaded cobalt unit described as the gamma-knife (GK) which uses multiple cobalt-60 sources arranged in a hemispherical distribution with collimators to achieve a circumscribed spherical dose distribution of 4-18mm diameter [20]. Subsequent development of the GK has allowed larger non-spherical tumors to be treated by combining several radiation spheres using a multiple isocenter technique.
Due to the invasive nature of the GK stereotactic head frame (surgically fixed to the skull), GK radiation treatment is delivered as a single large dose during one combined treatment planning and delivery session. This single fraction stereotactic radiation technique was termed ‘radiosurgery’ [18]. The GK radiosurgical procedure aimed to create a non-invasive radiation-based analogue of an open neurosurgical ablation of an intra-cranial target lesion. It should be emphasized, however, that aside from the use of a surgically-fitted stereotactic frame, GK radiosurgery and open neurosurgery are quite distinct procedures, and GK radiosurgery is a radiotherapeutic rather than a surgicalintervention, particularly as the commonly used doses are not “ablative”.
Subsequently, linear accelerators (linacs) were adapted to deliver radiosurgery (single fraction radiation) using multiple arcs of rotation, achieving the same dose distribution as that delivered by the GK. With the introduction of non-invasive relocatable stereotactic head frames, which enabled stereotactic radiation to be given in a number of treatment sessions, stereotactic radiotherapy was delivered as fractionated treatment to conventional doses [21, 22]. Initially, specifically adapted linacs were required, but the precision of modern linacs is now such that they do not generally require modification for stereotactic radiotherapy. The improved patient immobilization, more accurate tumor target localization using cross-sectional image for treatment planning, and high precision radiation treatment delivery to the tumor target, enabled a reduction in the margins around the radiotherapy target volume (the gross tumor volume (GTV) to planning target volume (PTV) margin), therefore achieving greater sparing of surrounding normal tissues than can be obtained with standard CRT techniques.
The miniaturization of a 6MV linear accelerator has allowed for its mounting on a high precision industrial robotic arm, and this has been combined with real time kV imaging for target tracking during treatment to create a robotic frameless stereotactic radiotherapy machine that is commercially known as the Cyberknife (CK) [23]. The CK uses multiple narrow, low dose rate photon beams, which have to be summated, to create a dose distribution equivalent to that achieved with other techniques. The need to summate contributions from multiple narrow beams results in longer treatment times per fraction than with other techniques and requires that CK treatment be given as a single large fraction (SRS), or as a few large fractions delivered over the course of a week or so (hypofractionated stereotactic radiotherapy).
While the term stereotactic radiotherapy continues to be used, “stereotaxy” as initially used for neurosurgery and subsequently for target localization in radiotherapy is no longer necessary and not in routine use, as modern MR and CT imaging with on treatment image guidance allow for equivalent high-precision treatment delivery. The appropriate modern terminology for the best and most accurate techniques of treatment delivery should be high precision conformal radiotherapy. Nevertheless, the term stereotactic used in conjunction with fractionated treatment (see below), while largely outmoded, remains in use with no clear meaning other than presumably denoting accuracy. Stereotactic localization, however, largely remains the standard of practice with single fraction treatment (GK radiosurgery).
Radiotherapy Fractionation
TERMINOLOGY
The term ‘radiosurgery’ is used for radiation treatment that is given as a single large dose (a single fraction), and the term radiotherapy is used for treatment that is given as multiple, usually daily, small doses over a period of weeks (fractionated treatment). The fractionation of radiation treatment is a mechanism for protecting normal tissues, through recovery between fractions, and permits the delivery of higher total doses of radiation than can be given as single fractions [24].
Similarly, stereotactic radiotherapy to the pituitary can be given in multiple doses as fractionated stereotactic conformal radiotherapy (SCRT or fSRT), or as a single large dose when it is described as stereotactic radiosurgery (SRS). SCRT/fSRT is generally delivered using a linac. SRS has most frequently been delivered using a GK, but can also be delivered using a linac or a robotic arm mounted linac (CK). Treatment given in fewer large fractions is described as hypofractionated RT.
BIOLOGICAL RATIONALE
The use of single fraction SRS is based on a belief, prevalent in the literature, that there is greater clinical benefit from single fraction rather than fractionated irradiation for pituitary adenomas. This belief was based on radiobiological modelling which defines equivalent radiation doses and fractionation schemes through biologically derived parameters [24, 25], mainly from the radiobiology of malignant tumors and some normal tissues. Such models are not validated for single fraction treatments [26], and the corresponding biological parameters necessary to calculate equivalent radiation doses do not exist for benign tumors. Publications claiming theoretical benefit of single fraction radiosurgery over fractionated irradiation [25] are based on constants that are not derived from experimental data and may therefore be misleading.
The therapeutic effect of radiation on malignant tumors is thought to be due to tumor cell attrition, either as apoptosis, or reproductive cell death, secondary to radiation-induced DNA damage. As a consequence, the time taken for an irradiated tissue to manifest radiotherapy related effects is proportional to the rate of cell proliferation in the tissue. In tissues with rapidly proliferating cells (malignant tumors), radiation effects are expressed either during or immediately after a course of radiotherapy, while in a tissue with a slowly proliferating cell population, such as benign tumors, radiotherapy effects may take many months or years to manifest. It is assumed that the beneficial effects of radiation in pituitary adenomas conform to these same mechanistic principles with the radiation-induced depletion of pituitary adenoma tumor cells, and with the adenoma being considered a slowly proliferating tissue. As benign tumors are rarely grown in culture, the precise mechanism of the observed clinical benefit of irradiation is not elucidated and remains largely theoretical. The surrounding normal brain tissue is also considered to consist largely of slowly proliferating cell populations, although critical cell populations with faster turnover, such as blood vessels, are also present and are affected by radiation.
DOSE FRACTIONATION SCHEMES FOR PITUITARY ADENOMAS
Conventional CRT and fractionated SCRT are given to total dose of 45 to 50 Gy at 1.8 Gy per fractionation, once a day, five days per week. These treatment doses are below the tolerance of central nervous system neural tissue, and the risk of structural damage due to such treatment is <1% [27, 28]. While, theoretically, single large doses of radiation as used in SRS may result in a higher tumor cell kill than the equivalent total dose given over a small number of fractions, this is also true for the normal tissue cell population and leads to normal tissue toxicity which may not be acceptable if it affects critical regions such as optic chiasm [28].
As most pituitary adenomas requiring radiation treatment lie in close proximity to the optic apparatus, and to the cranial nerves in the cavernous sinus, SRS is suitable only for small lesions located away from critical structures, and the optic apparatus should not exceed single doses above 8Gy [28]. Fractionated SRT, using up to 5-fractions over a week course, is another feasible alternative fractionation scheme delivered by LINACs, Cyberknife or frameless radiosurgery.
For larger NFPA with chiasmatic involvement, hypofractionation can allow for safe delivery of enhanced biologically effective doses compared to conventional fractionation. The safety of this scheme has been recently reported in a cohort of NFPA, the majority with abutment or compression of optic chiasm, who had satisfactory local control compared to SRS with acceptable toxicity for visual preservation [29].
Linac Based SCRT/FSRT
For fractionated stereotactic radiotherapy, patients are immobilized in a non-invasive relocatable frame with a relocation accuracy of 1-2mm [21, 22], or a precisely fitting thermoplastic mask system with an accuracy of 2-3mm [16]. Sub-millimeter repositioning accuracy can now be achieved with thermoplastic mask immobilization by means of image guidance techniques which can determine and apply daily online setup corrections [30]. As for conventional CRT, the GTV is outlined on an MRI scan co-registered with a CT scan. The PTV margin used for SCRT is smaller than for conventional CRT, typically in the region of 3-5mm based on the overall accuracy of the treatment system, the principal determinant of which is the repositioning accuracy of the patient in the immobilization device [31] and the ability to correct it with on treatment imaging (image guidance). For such precision treatment, accurate localization of the tumor volume is of paramount importance in order to avoid treatment failure due to exclusion of a part of the tumor from the treatment volume.
SCRT employs a larger number of radiotherapy beams than conventional CRT (usually 4-6). Each beam is conformed to the shape of the PTV using a narrow leaf MLC (5mm width known as mini MLC, or 3mm width known as micro MLC). MLC leaves can be used to modulate the intensity of the radiation beam during its delivery as in intensity-modulated radiotherapy (IMRT). More recently, arc-based or rotational techniques (volumetric modulated arc therapy or VMAT) have been introduced in the clinical practice to overcome some of the limitation of IMRT (complex planning and QA process). The continuous rotation of the radiation source allows the patient to be treated from a full 360° beam angle in a shorter time interval. Fractionated SCRT (fSRT) combines the precision of stereotactic patient positioning and treatment delivery with standard radiotherapy fractionation, which preferentially spares normal tissue. Complete avoidance of surrounding normal tissue structures, such as the optic apparatus, is not generally practiced, as the dose fractionation schemes used are below the radiation tolerance doses of the CNS. Nonetheless arc techniques are used to minimize the dose bilaterally to the temporal lobes with the aim of reducing the impact of treatment on patients’ cognitive function. The fractionated SCRT technique is suitable for pituitary adenomas of all sizes, regardless of their relationship to adjacent critical normal tissue structures.
Linac Based SRS
Linac based SRS can be delivered using either a relocatable or an invasive neurosurgical stereotactic frame. Use of an invasive neurosurgical frame necessitates that the treatment planning and delivery procedures are carried out and completed within a single day. Computerized treatment planning defines the arrangement of the radiation beams, as in SCRT. SRS can be planned either as multiple arcs of rotation, simulating GK SRS treatment, and producing small spherical dose distributions, or as multiple fixed conformal fields. Multiple arc SRS using a linear accelerator, employing multiple isocenters, is a cumbersome and rarely used technique. The use of multiple fixed fields is generally confined to fractionated treatment, although it can also be used for single fraction SRS. Because of the potentially damaging effect of large single fraction radiation doses on normal tissue structures, SRS is only suitable for small pituitary adenomas that are at least 3-5mm away from the optic chiasm.
Several dosimetry studies have shown that linear accelerators could deliver the same SRS doses to pituitary tumors as GK, with comparable conformity indices and OAR doses. Linac SRS has the advantage of being available, efficient with a less beam-on time, so could be considered for radiosurgery of pituitary adenomas [32]..
Gamma Knife SRS
For GK SRS, patients are immobilized in an invasive neurosurgical stereotactic frame. A relocatable non-invasive stereotactic frame has become available, enabling the delivery of hypofractionated stereotactic radiotherapy treatment in addition to SRS, and experience with this system is increasing [33, 34]. GK SRS delivers a single high dose, in a spherical distribution, of 4-18mm diameter. Larger, non-spherical tumors, which represent the majority of pituitary adenomas, are treated by combining several such spherical dose volumes using a multiple isocenter technique. The appropriate number and distribution of isocenters is defined using a 3D computer planning system which also allows for selective plugging of some of the cobalt source positions to enable shaping of the high dose volume envelope. The use of multiple isocenters results in dose inhomogeneity within the target volume, with small areas of high radiation dose (hot spots) in the regions of overlap of the radiation dose spheres. This may lead to radiation damage if critical normal structures, such as cranial nerves, lie within these hot spots. GK SRS is given to doses of 12 - 35Gy to the tumor margin with doses to the optic chiasm and the other cranial nerves in the cavernous sinus limited to 8-10Gy and 16-18 Gy respectively. royal sinus invasion has been reported as a significant predictor of poor outcomes after surgical resection. Different series have shown good local control using GK for positive residuals within the cavernous sinus after surgical resection [35-39].
Although the total dose delivered with fractionated meanings of irradiation is largely consistent within different publications (45-50.4 Gy), the range of dose prescriptions between secretory and non-functioning adenomas treated with single fraction SRS tends to be different. The rationale behind this practice is based on the observation that a more rapid hormone normalization was reported in single studies using higher doses to treat secreting tumors [40, 41]. In absence of a strong radiobiological model and of prospective randomized studies in support, the relationship between dose and endocrine remission warrants further investigation.
Robotic Mounted Linac SRS
Cyberknife has been used to treat pituitary adenomas using a variety of dose/fractionation regimens, with a tendency to deliver treatment as hypofractionated radiotherapy in 3 to 5 fractions, rather than as single fraction SRS doses.
Proton Therapy
Proton beams, heavy charged particles with similar radiobiological effectiveness as photons, have been in use at a small number of centers with the relevant facilities since the late 1960s [42, 43]. Proton therapy was initially used in two US centers (Boston, MA, and Loma Linda, CA) and then subsequently in Europe (d’Orsay, France) and Japan (Tsukuba, Japan); these centers have reported the majority of the initial clinical results. The introduction of proton therapy had been underpinned by planning studies demonstrating, in selected cases, improved dose distribution of protons compared with photons.
The principal theoretical advantage of proton therapy over photon therapy is the deposition of energy at a defined depth in tissue (the Bragg peak) with little energy deposition beyond that point [44]. These properties make the use of protons appealing for tumors lying in close proximity to critical dose-limiting normal tissues, which is a bar to safe dose escalation using conventional photon radiotherapy, or when a reduction of low dose (the low dose radiation “bath” responsible for the late sequelae of radiotherapy) to the normal brain tissues is of particular clinical evidence, as in children.
Current indications for the use of protons within the UK Specialized Commissioning Team include the treatment of craniopharyngiomas and pituitary adenomas up to the age of 24 years old based on theoretical reduction in the possible late side effects of brain radiation, such as second malignancy, neuro-cognitive deficits and cerebrovascular disease [45].
Peptide Receptor Radionuclide Therapy (PRRT)
PRRT is a form of internal radiation therapy directed to the tumor tissues expressing peptide receptors using gamma emitting radiopharmaceuticals. It is typically used for neuroendocrine tumors; however, it was investigated as a treatment option for aggressive pituitary tumors refractory to other treatment modalities. Different pituitary tumors express somatostatin receptors and show uptake of radiolabeled somatostatin analogues like 68Ga-DOTATATE. The 2018 guidelines of the European Society of Endocrinology listed PRRT as an alternative treatment option for aggressive pituitary tumors refractory to other lines of treatment including temozolomide [46].The treatment doses and the type of nucleotide used varied in the available studies, with only small patient numbers being reported [47, 48].
CLINICAL OUTCOMES FOLLOWING PITUITARY RADIOTHERAPY
The clinical efficacy of radiotherapy for pituitary adenomas should be assessed by overall survival, actuarial tumor control (progression-free survival, PFS), and quality of life. Few publications focused on quality of life assessment after radiotherapy in pituitary tumors [49-51], while commonly reported endpoints for retrospective studies of radiation treatment for non-functioning pituitary adenomas are local tumor control, and long term morbidity.
In patients with functioning pituitary adenomas, the principal endpoint, in addition to PFS and morbidity, is the rate of normalization of elevated pituitary hormone levels. The rate of pituitary hormone decline after irradiation varies with the type of functioning tumor, and the time to reach normal hormone levels is dependent on the initial pre-treatment hormone levels [52]. The appropriate comparative measure for each pituitary hormone is the time to reach 50% of the pre-treatment hormone level, and this should be corrected for the confounding effect of medical treatment.
Surrogate endpoints such as ‘tumor control rate’ and the ‘proportion of patients achieving normal hormone levels’ do not, of themselves, provide adequate information on the efficacy of different pituitary irradiation techniques and are potentially misleading [53]. Tumor control rate must be quoted with an indication of the time or duration of follow-up required to achieve the stated level of control. Similarly, the proportion of patients achieving normal hormone levels following treatment is meaningful only when described in terms of the relationship to pre-treatment hormone levels. Due to the use of such surrogate endpoints in published retrospective series, inappropriate and incorrect claims have been made in the literature for superiority of one technique of irradiation over another.
Given that the published data on the efficacy of the various available techniques for pituitary irradiation consist entirely of retrospective case-series, the available data inevitably suffer from selection bias. While SCRT is suitable for the treatment of all pituitary tumors, irrespective of size, shape or proximity to critical normal tissue structures, SRS is only suitable for treatment of small tumors away from the optic chiasm. As a result, studies reporting the efficacy of SRS mostly deal with smaller tumors, which are typically associated with lower hormone levels if the adenomas are functioning. Therefore, the reported results of studies of SRS do not necessarily apply to the generality of pituitary adenomas and may give a false impression of greater efficacy if only more favorable cases are treated.
THE EFFICACY AND TOXICITIES OF TREATMENT
Conventional RT and CRT
The efficacy of modern stereotactic pituitary radiotherapy and pituitary radiosurgical techniques must be assessed in the light of the results achieved with standard treatment, which is conventional conformal radiotherapy. Large and mature case series provide data on the long-term effectiveness of CRT in controlling pituitary tumor growth and hormone secretion.
TUMOR CONTROL
The long-term results following pituitary CRT from case series published in the literature are shown in Table 1 [5-14, 17, 54-66]. The actuarial PFS is in the region of 80%-90% at 10 years and 75%-90% at 20 years [14, 55]. The single largest series of patients with pituitary adenomas treated with conventional fractionated radiotherapy is that from The Royal Marsden Hospital which reported a 10-year PFS of 92% and a 20-year PFS of 88% [8].
Post operative radiotherapy has been reported to provide excellent local control of non-functioning tumors when offered for progressive residual disease with almost no radiological evidence of tumor progression up to 15 years of follow-up [67].
ENDOCRINE CONTROL
Fractionated irradiation leads to normalization of excess pituitary hormone secretion in the majority of patients, albeit with some time delay following treatment. For acromegaly, RT achieves normalization of GH/IGF-I levels in 30-50% of patients at 5-10 years, and in 75% of patients at 15 years, after treatment (Table 2) [14, 55]. As the time to normalization of GH levels is related to the pre-treatment GH level, the time to achieve a 50% reduction in GH levels, which takes into account the starting GH level, is in the region of 2 years, with IGF-1 reaching half of pre-treatment levels somewhat after the GH [58, 60].
A 10-year follow-up for more than 600 acromegaly patients was published by the Swedish Pituitary Register 2022. It has reported 78% of IGF-1 normalization rate with an annual rate of increased hormonal control of 1.23%. One third of the patients required bi-modality therapy to achieve hormonal control and 5% required triplet therapy i.e. surgical resection, medical treatment and radiotherapy with a trend towards reduced use of conventional radiotherapy doses [68].
Table 1. Summary of Results of Published Series on Conventional RT for Pituitary Adenomas |
||||||
Authors |
Type of adenoma |
Number of patients |
Follow-up (median years) |
Actuarial progression free survival (PFS) (%) |
Late toxicity (%) Visual Hypopituitarism |
|
Grigby at al.,1989 [6] |
NFA, SA |
121 |
11.7 |
89.9 at 10 years |
1.7 |
NA |
McCollough et al., 1991 [7] |
NFA, SA |
105 |
7.8 |
95 at 10 years |
NA |
NA |
Brada et al., 1993 [8] |
NFA, SA |
411 |
10.8 |
94 at 10 years 88 at 20 years |
1.5 |
30 at 10 years |
Tsang et al., 1994 [9] |
NFA, SA |
160 |
8.7 |
87 at 10 years |
0 |
23** |
Zierhut et al., 1995 [10] |
NFA, SA |
138 |
6.5 |
95 at 5 years |
1.5 |
27** |
Estrada et al., 1997 [56] |
SA (ACTH) |
30 |
3.5 |
73 at 2 years* |
0 |
48** |
Rush et al., 1997 [11] |
NFA, SA |
70 |
8 |
NA |
NA |
42** |
Breen et al., 1998 [12] |
NFA |
120 |
9 |
87.5 at 10 years |
1 |
NA |
Gittoes et al., 1998 [5] |
NFA |
126 |
7.5 |
93 at 10 and 15 years |
NA |
NA |
Barrande et al., 2000 [57] |
SA (GH) |
128 |
11 |
53 at 10 years* |
0 |
50 at 10 years |
Biermasz et al., 2000 [58] |
SA (GH) |
36 |
10 |
60 at 10 years* |
0 |
54 at 10 years |
Sasaki et al., 2000 [13] |
NFA, SA |
91 |
8.2 |
93 at 10 years |
1 |
NA |
Epaminonda et al., 2001 [59] |
SA (GH) |
67 |
10 |
65 at 15 years* |
0 |
NA |
Minniti et al., 2005 [60] |
SA (GH) |
45 |
12 |
52 at 10 years* |
0 |
45 at 10 years |
Langsenlehner et al., 2007 [61] |
NFA, SA |
87 |
15 |
93 at 15 years
|
0 |
88 at 10 years |
Minniti et al., 2007 [62] |
SA(ACTH) |
40 |
9 |
78 and 84 at 5 and 10 years* |
0 |
62 at 10 years |
Rim et al., 2011 [63] |
NFA, SA |
60 |
5.6 |
96 at 10 years (NFA), 66 at 10 years (SA) |
0 |
76 at 10 years |
Kim et al., 2016 [65] |
NFA, SA |
73 |
8 |
98 at 10 years |
0 |
NA |
Patt et al., 2016 [66] |
SA (GH) |
36 |
4.9 (mean) |
89 at 5 years |
0 |
33 |
NFA, non-functioning adenoma; SA, secreting adenoma; NA, not assessed, ACTH-Cushing, GH- acromegaly, *hormone concentration normalization, **no time specified
After RT for Cushing’s disease, urinary free cortisol (UFC) is reduced to 50% of the pre-treatment levels after an interval of 6-12 months, and plasma cortisol after around 12 months [62]. The median time to cortisol level normalization is around 24 months after treatment [62]. The overall tumor and hormone control rates in the reported studies, after a median follow-up of 8 years, are 97% and 74% respectively [64]. Pituitary radiotherapy is rarely used to treat patients with prolactinoma. Occasional patients who fail surgery and medical therapy have been treated with RT, and the reported 10-year tumor and hormone control rates are 90% and 50% respectively [69-71].
TOXICITY
The toxicity of RT with total treatment doses of 45-50Gy with daily fraction sizes of < 2Gy is low. The principal toxicities reported in studies of CRT are described in Table 1.
Hypopituitarism
Hypopituitarism is the most common long-term complication following RT, reported to occur in 30-60 % of patients by 10 years after treatment [8, 9, 14]. Pituitary hormone loss is observed to occur in a characteristic sequence, with GH secretion being affected most frequently, followed by the gonadotrophins, ACTH, and then TSH. Long term follow-up after pituitary irradiation, with intermittent testing for deficiency of all pituitary axes, is therefore an essential part of the post-treatment management of these patients.
Visual Pathways Deficit and Other Structural CNS Damage
The reported incidence of optic neuropathy resulting in visual deficit following CRT is 1-3% [8, 9]. The occurrence of necrosis of normal brain tissue is almost unknown following pituitary RT, although this complication has been reported to occur in 0.2% of patients [72].
Cerebrovascular Disease
Pituitary disease is, in itself, associated with increased mortality, principally due to vascular disease [73]. An increased incidence of stroke (relative to the general population) in patients treated with RT for both non-functioning and functioning pituitary adenomas has been reported in a number of retrospective cohort studies [74-77]. Whilst it is has long been known that radiotherapy can lead to vascular injury [78], it is not at present clear how much of the excess stroke risk following RT is attributable to radiotherapy, and how much may be due to other potential causes including the metabolic and cardiovascular consequences of hypopituitarism, the effects of associated endocrine syndromes, and the consequences of surgery.
In a retrospective cohort study of 342 patients treated with pituitary surgery and post-operative RT, 31 patients died from stroke after a median follow-up interval of 21 years (range, 2-33) [77] and in all cases the probable location of the stroke lesion was within the irradiated volume. Comparison of stroke patients with matched control patients without stroke drawn from the same cohort showed no significant differences in radiotherapy-dependent variables with the exception of the pre-treatment duration of symptoms of hypopituitarism. This suggests that untreated hormone deficiency may be a significant factor in the pathogenesis of stroke in patients treated for pituitary adenoma, rather than or in addition to treatment with radiotherapy. It is likely that the cause of stroke in patients treated with RT for pituitary adenoma is multi-factorial, and the relative contributions of the various possible contributory factors remains to be determined.
Second Brain Tumor
Intracranial radiotherapy is associated with the development of second, radiation-induced, brain tumors. The cumulative incidence of gliomas and meningiomas following radiotherapy for pituitary adenomas in retrospective case series is reported to be in the region of 2% at 20 years [77, 79-81]. A large retrospective study of patients who received radiotherapy for pituitary and sellar lesions has shown a relative risk of 3.34 (95% confidence interval 1.06-10.6) for development of malignant brain tumors and 4.06 (95% confidence interval 1.51-10.9) for development of meningiomas in comparison with patients who did not receive radiotherapy. Rates were higher in those treated with radiotherapy at a younger age, and there was no difference in incidence rates between conventional or stereotactic radiotherapy (70).
In another large retrospective cohort of more than 3600 patients from six adult endocrinology registries, incidence of secondary brain tumors was compared between irradiated and non-irradiated patients with pituitary adenomas and craniopharyngiomas. The relative risk of secondary brain tumors for irradiated patients was 2·18 (95% CI 1·31-3·62, p<0·0001). Cumulative probability of second brain tumor was 4% for the irradiated and 2·1% for the controls at 20 years. Radiotherapy exposure and older age at pituitary tumor detection were associated with increased risk of second brain tumor [82].
Cognitive Deficit
Radiotherapy treatment to significant volumes of normal brain in children is associated with subsequent neuro-cognitive impairment [27]. However, the evidence for the effect of radiotherapy treatment to small volumes of brain on neuro-cognitive function in adults is weak [27]. The effect of pituitary radiotherapy on neuro-cognitive function is particularly difficult to discern as this cannot be differentiated from the effect of other treatment interventions, and from the effects of the tumor itself [83-85].
A retrospective study of 84 patients following transsphenoidal surgery, of whom 39 received post-operative radiotherapy, compared neuro-cognitive function with a large reference sample, considered to be representative of normal population without pituitary disease. While the pituitary cohort had lower scores on the tests of both memory and executive function in comparison with the reference sample, patients who had received radiotherapy showed no significant difference compared to patients treated with surgery alone [86]. A dosimetric study did not find a correlation between radiotherapy dose to the hippocampus and pre-frontal cortex (brain regions known to be important in memory and executive function) and conformal technique of irradiation with cognitive performance [87].
Stereotactic Conformal Radiotherapy (SCRT/FSRT)
SCRT achieves tumor control and normalization of pituitary hormone hypersecretion at rates similar to the best reports following conventional RT. Longer duration follow-up is required to demonstrate the presumed lower incidence of long-term morbidity following SCRT compared to conventional RT. The results from reported studies of SCRT are summarized below.
TUMOR CONTROL
SCRT data for 1166 patients with either non-functioning or functioning pituitary adenomas have been reported in 21 studies to date (Table 2) [14, 17, 55, 64, 88-105]. Analysis of published data up to 2020 shows that, at a corrected median follow-up of 56 months (range 9-152 months), tumor control was achieved in 96% of patients. The 5-year actuarial PFS of 92 patients (67 non-functioning, 25 functioning) treated at The Royal Marsden Hospital was 97% [93]. These results are similar to the results seen in patient cohorts treated with conventional RT (Table 1).
ENDOCRINE CONTROL
Detailed data on the rate of pituitary hormone decline are not available, although this is expected to be similar to that seen following conventional RT as the same dose-fractionation is used. In The Royal Marsden case series, 6 of 18 acromegalic patients (35%) had normalization of GH/IGF-I levels after a median follow-up of 39 months [93]. Similarly, in another single center study of 20 patients treated with SCRT, normalization of GH levels was reported in 70%, and local tumor control in 100% after a median follow-up of 26 months [90]. The data available on SCRT for patients with Cushing’s disease are limited. In a small series of 12 patients, control of elevated cortisol was reported in 9 out of 12 patients (75%) after a median follow-up of 29 months [92].
TOXICITIES
Following SCRT, hypopituitarism has been reported in 22% of patients after an overall corrected median follow-up of 57 months (Table 2). The length of follow-up after SCRT is shorter than reported for the mature cohorts treated with RT. It is likely that the rate of hypopituitarism following SCRT will continue to increase as the duration of follow-up increases particularly as the technique of SCRT generally does not avoid either the hypothalamus or the remaining pituitary gland. Other late complications have been rarely reported after SCRT. While the incidence of treatment-related morbidity with SCRT appears to be low, longer duration follow-up is necessary to detect normal tissue toxicity that may only become manifest at a low frequency many years after treatment.
Table 2. Summary of Results on Published Studies on SCRT for Pituitary Adenomas |
|||||
Authors |
Number of patients |
Follow-up median (months) |
Tumor growth control rate (%) |
Late toxicity (%) Visual Hypopituitarism |
|
Coke et al., 1997 [88] |
19* |
9 |
100 |
0 |
0 |
Mitsumori et al., 1998 [89] |
30* |
33 |
86 at 3 years |
0 |
20 |
Milker-Zabel et al., 2001 [90] |
68* |
38 |
93 at 5 years |
7 |
5 |
Paek et al., 2005 [91] |
68 |
30 |
98 at 5 years |
3 |
6 |
Colin et al., 2005 [92] |
110* |
48 |
99 at 5 years |
2 |
29 at 4 years |
Minniti et al., 2006 [93] |
92* |
32 |
98 at 5 years |
1 |
22 |
Selch et al., 2006 [94] |
39* |
60 |
100 |
0 |
15 |
Kong et al., 2007 [95] |
64* |
37 |
97 at 4 years |
0 |
11 |
Snead et al., 2008 [96] |
100* |
6.7 years |
98 and 73 at 10 years for NFA and SA |
1 |
35 |
Roug et al., 2010 [97] |
34* |
34 |
91 (50% hormonal normalization) |
- |
- |
Schalin-Jantti et al., 2010 [98] |
30 |
5.3 years |
100 |
0 |
23 |
Weber et al., 2011 [99] |
27* |
72.4 |
96 |
4 |
8 |
Wilson et al., 2012 [100] |
67 |
5.12 years |
88 |
2 |
6 |
Kim et al., 2013 [101] |
76* |
6.8 years |
97.1 at 7 years |
0 |
48 (one or more hormone) |
Kopp et al., 2013 [102] |
37 |
57 |
91.9 |
5 |
43 |
Liao et al., 2014 [106] |
34~ |
36.8 (mean) |
100 |
0 |
NA |
Minniti et al., 2015 [103] |
68 |
75 |
97 and 91 at 5 and 10 years |
0 |
26 |
Puataweepong et al., 2015 [107] |
94* |
72 |
95 |
3 |
9.6 |
Diallo et al., 2015 [104] |
34* |
152 (mean) |
97 |
0 |
39 |
Barber et al., 2016 [105] |
75* |
47.5 (mean) |
100 |
1.5 |
28 |
Lian et al., 2020 [108] |
113* |
36 |
99 |
0 |
28.3 |
* Case series includes secreting adenomas
Radiosurgery (SRS)
TUMOR CONTROL
The published results of GK SRS for patients with non-functioning and functioning pituitary adenomas have been summarized in systematic reviews [14, 17, 55, 64] and an update with more recently published studies is given in Table 3 [14, 17, 35, 55, 64, 100, 109-130]. The majority of published reports provide information on tumor ‘control rate’, without specifying a time-frame, and therefore provide little useful information on the efficacy of GK SRS. The summary figure for the actuarial 5-year control rate (PFS) following GK SRS for non-functioning adenomas is 95% at 5 years (few 10-year results are available). This is a lower rate of tumor control than expected following RT & SCRT, particularly when it is considered that only small tumors suitable for GK SRS are treated, compared to that adenoma of all sizes treated with RT, CRT & SCRT.
Table 3. Summary of Results of Published Series on SRS for Non-Functioning Pituitary Adenomas |
|
|||||
Authors |
Number of patients |
Follow-up median (months) |
Tumor control growth rate (%) |
Late toxicity (%) Visual Hypopituitarism |
|
|
Martinez et al., 1998 [109] |
14 |
26-45 |
100 |
0 |
0 |
|
Pan et al., 1998 [110] |
17 |
29 |
95 |
0 |
0 |
|
Ikeda et al., 1998 [35] |
13 |
45 |
100 |
0 |
0 |
|
Mokry et al., 1999 [111] |
31 |
20 |
98 |
NA |
NA |
|
Sheehan et al., 2002 [112] |
42 |
31* |
97 |
2.3 |
0 |
|
Wowra et al., 2002 [113] |
45 |
55 |
93 at 3 years |
0 |
14 |
|
Petrovich et al., 2003 [114] |
56 |
36 |
94 at 3 years |
4 |
NA |
|
Pollock et al., 2003 [115] |
33 |
43 |
97 at 5 years |
0 |
28 and 41 at 2 and 5 years |
|
Losa et al., 2004 [116] |
56 |
41* |
88 at 5 years |
0 |
24 |
|
Iwai et al., 2005 [117] |
34 |
60 |
93 at 5 years |
0 |
6 |
|
Mingione et al., 2006 [118] |
100 |
45* |
92 |
0 |
25 |
|
Liscak et al., 2007 [119] |
140 |
60 |
100 |
0 |
2 |
|
Pollock et al., 2008 [120] |
62 |
63 |
95 at 3 and 7 years |
0 |
32 at 5 years |
|
Kobayashi et al., 2009 [121] |
60 |
>3 years |
97 |
4.3 |
8.2 worsening |
|
Gopalan et al., 2011 [122] |
48 |
80.5 |
83 |
9.4 |
39 |
|
Park et al., 2011 [124] |
125 |
62 |
94 at 5 years and 76 at 10 years |
1 |
24 at 2 years |
|
Wilson et al., 2012 [100] |
51 |
4.17 years |
100 |
0 |
0 |
|
Runge et al., 2012 [123] |
61 |
83 |
98 |
0 |
9.8 |
|
Starke et al., 2012 [125] |
140 |
4.2 years |
97 at 5 and 87 at 10 years |
12.8 |
30.3 |
|
El-Shehaby et al., 2012 [126] |
38 |
44* |
97 |
0 |
0 |
|
Sheehan et al., 2013+ [127] |
512 |
36 |
95 at 5 years |
6.6 |
21 |
|
Lee et al., 2014 [128] |
41 |
48 |
94 at 5 and 85 at 10 years |
2.4 |
24.4 |
|
Xu et al., 2014 [129] |
34 |
56 |
73 at 3 years |
24 |
29 |
|
Hasegawa et al., 2015 [130] |
16 |
98 |
100 |
0 |
6 |
|
Graffeo et al., 2018[131] |
57 |
48 |
99 |
NA |
31 at 5years |
|
Oh et al., 2018 [132] |
76 |
53.5 |
96 |
NA |
24.5 |
|
Cordeiro et al., 2018 [133] |
410 |
51 |
94.4 |
NA |
34.7 |
|
Narayan et al., 2018 [134] |
87 |
48.2 |
90 |
8.1 |
20.7 |
|
Slavinsky P et al., 2022 [135]
|
28 |
63 |
94.2 |
NA |
26% |
|
Maldar AN, et al.,2022[136] |
63 |
47 |
87.3 |
NA |
26% at 5 years 29.7% at 10 years |
*Mean follow-up; NA: not available, + multicenter study, 34 patients had prior CFSR
ENDOCRINE CONTROL WITH GK SRS
The reported endocrine outcomes following GK SRS for acromegaly are shown in Table 4 [14, 36, 40, 55, 64, 109-111, 114, 121, 137-163]. A summary analysis of the published literature up to 2020 shows that -41% of patients achieved normalization of serum GH, after a median follow-up of 46 months. The time to reach 50% of baseline serum GH, reported in only three studies, is in the region of 1.5-2 years with a slower reduction in IGF-I levels [147, 150, 164], which is similar to the rate reported following conventional RT/CRT.
Table 4. Summary of Results of Published Series on SRS for GH-Secreting Pituitary Adenomas |
|
|||||
Authors |
Number of patients |
Follow-up median (months) |
Hormone normalization* (%) |
Late toxicity (%) Visual Hypopituitarism |
|
|
Thoren et al., 1991 [137] |
21 |
64 |
10 |
0 |
15 |
|
Martinez et al., 1998 [109] |
7 |
26-45 |
NA |
0 |
0 |
|
Pan et al., 1998 [110] |
15 |
29 |
NA |
0 |
0 |
|
Morange-Ramos et al., 1998 [138] |
15 |
20 |
20 |
6 |
16 |
|
Lim et al., 1998 [139] |
20 |
26 |
30 |
5 |
5 |
|
Kim et al., 1999 [165] |
11 |
27 |
35 |
NA |
NA |
|
Landolt et al., 1998 [141] |
16 |
17 |
50 |
0 |
16 |
|
Mokry et al., 1999 [111] |
16 |
46 |
31 |
0 |
NA |
|
Hayashi et al., 1999 [142] |
22 |
>6 |
41 |
0 |
0 |
|
Inoue et al., 1999 [143] |
12 |
>24 |
58 |
0 |
0 |
|
Zhang et al., 2000 [144] |
68 |
>12 |
40 |
NA |
NA |
|
Izawa et al., 2000 [145] |
29 |
>6 |
41 |
0 |
0 |
|
Pollock et al., 2002 [146] |
26 |
36 |
47 |
4 |
16 |
|
Attanasio et al., 2003 [147] |
30 |
46 |
23 |
0 |
6 |
|
Choi et al., 2003 [148] |
12 |
43 |
30 |
0 |
0 |
|
Jane et al., 2003 [149] |
64 |
>18 |
36 |
0 |
28 |
|
Petrovich et al., 2003 [114] |
6 |
36 |
100 |
0 |
NA |
|
Castinetti et al., 2005 [150] |
82 |
49.5* |
17 |
0 |
18 |
|
Gutt et al., 2005 [151] |
44 |
22 |
48 |
NA |
NA |
|
Kobayashi et al., 2005 [152] |
67 |
63 |
17 |
0 |
NA |
|
Jezkova et al., 2006 [153] |
96 |
54 |
50 |
0 |
26 |
|
Pollock et al., 2007 [154] |
46 |
63 |
11 and 60 at 2 and 5 years |
0 |
33 at 5 years |
|
Jagannathan et al., 2009 [155] |
95 |
57 * |
53 |
5# |
34 (new) |
|
Kobayashi, 2009 [121] |
49 |
63 |
17 (normal or nearly normal) |
11 |
15 |
|
Wan et al., 2009 [156] |
103 |
60 (minimum) |
37 |
0 |
1.7** |
|
Castinetti et al., 2009 [157] |
27 |
60 (minimum) |
42 at 50 months |
1.3** |
23** |
|
Iwai et al., 2010 [158] |
26 |
84 |
38 |
0 |
8 |
|
Hayashi et al., 2010 [36] |
25 |
36* |
40 |
0 |
0 |
|
Erdur et al., 2011 [159] |
22 |
60 |
55 |
0 |
29 |
|
Sheehan et al., 2011 [40] |
130 |
30 |
53 at 30 months |
0 |
34 |
|
Franzin et al., 2012 [160] |
103 |
71 |
56.9 at 5 years |
0 |
7.8 (new) |
|
Liu et al., 2012 [161] |
40 |
72 |
57.5 |
0 |
40 (new) |
|
Zeiler et al., 2013 [162] |
21 |
33 |
30 |
3.9 |
13.2 |
|
Lee et al., 2014 [163] |
136 |
61.5 |
64.5 and 82.6 at 4 and 8 years |
3 |
33.1 |
|
Cordeiro et al., 2018 [133] |
351 |
51 |
NA |
NA |
38.7 |
|
Gupta et al.,2018 [166] |
25 |
69.5 |
28 |
NA |
19.6 |
|
Ding et al., 2019 [167] |
371 |
79 |
59 at 10 years |
4 |
26 |
|
*mean follow-up; NA not assessed, #3 had previous RT, **whole series
A summary analysis of the published literature up to 2020, for patients with Cushing’s disease, shows that 52% achieved biochemical remission (as defined by plasma cortisol and 24-hour UFC level) at a corrected median follow-up of 50 months after SRS (Table 5) [14, 36, 40, 55, 64, 109-111, 114, 121, 138-140, 142, 143, 145, 146, 148, 149, 155-157, 162, 165, 168-178]. The reported time to hormonal normalization ranged from 3 months to 3 years, with no clear difference in the rate of decline of hormone level compared to RT/CRT. The largest single series of GK SRS for Cushing’s disease reported a remission rate of 54%, with 20% of patients who achieved remission subsequently relapsing, suggesting a higher failure rate following GK SRS than following RT/CRT [179].
Table 5. Summary of Results of Published Series on SRS for ACTH-Secreting Pituitary Adenomas |
|
||||||
Authors |
Number of patients |
Follow-up median (months) |
Tumor growth control rate (%) |
Hormone normalization*% |
Late toxicity (%) Visual Hypopituitarism |
|
|
Degerblad et al., 1986 [168] |
29 |
3-9 years |
76 |
48 |
NA |
55 |
|
Ganz et al., 1993 [169] |
4 |
18 |
NA |
NA |
0 |
NA |
|
Seo et al., 1995 [170] |
2 |
24 |
100 |
NA |
0 |
NA |
|
Martinez et al., 1998 [109] |
3 |
26-45 |
100 |
100 |
0 |
0 |
|
Pan et al., 1998 [110] |
4 |
29 |
95 |
NA |
0 |
0 |
|
Morange-Ramos et al., 1998 [138] |
6 |
20 |
100 |
66 |
0 |
16 |
|
Lim et al., 1998 [139] |
4 |
26 |
NA |
25 |
2 |
2 |
|
Mokry et al., 1999 [111] |
5 |
26 |
93 |
20 |
0 |
2 |
|
Kim et al., 1999 [165] |
8 |
26 |
100 |
60 |
NA |
NA |
|
Hayashi et al., 1999 [142] |
10 |
>6 |
100 |
10 |
0 |
5 |
|
Inoue et al., 1999 [143] |
3 |
>24 |
100 |
100 |
0 |
0 |
|
Izawa et al., 2000 [145] |
12 |
>6 |
100 |
17 |
NA |
0 |
|
Sheehan et al., 2000 [171] |
43 |
44 |
100 |
63 |
2 |
16 |
|
Hoybye et al., 2001 [172] |
18 |
17 years |
100 |
83 |
0 |
66 |
|
Kobayashi et al., 2002 [173] |
20 |
60 |
100 |
35 |
NA |
NA |
|
Pollock et al., 2002 [146] |
11 |
36 |
85 |
35 |
35 |
8 |
|
Choi et al., 2003 [148] |
9 |
43 |
100 |
55 |
0 |
0 |
|
Jane et al., 2003 [149] |
45 |
>18 |
100 |
63 |
1 |
31 |
|
Petrovich et al., 2003 [114] |
4 |
36 |
NA |
50 |
0 |
NA |
|
Devin et al., 2004 [174] |
35 |
35 |
91 |
49 |
0 |
40 |
|
Castinetti et al., 2007 [175] |
40 |
54 |
100 |
42 |
0 |
NA |
|
Jagannathan et al., 2009 [155] |
90 |
45 |
96 |
54 |
6 |
22 |
|
Kobayashi, 2009 [121] |
25 |
64(mean) |
100 |
35 |
NA |
NA |
|
Wan et al., 2009 [156] |
68 |
60(minimum) |
90 |
28 |
0 |
1.7 |
|
Castinetti et al., 2009 [157] |
18 |
60(minimum) |
NA |
50 at 28 months |
1.3** |
23** |
|
Hayashi et al., 2010 [36] |
13 |
36(mean) |
97 |
38 |
0 |
0 |
|
Sicignano et al., 2012 [178] |
15 |
60 |
97.7 |
64 |
NA |
12.3 |
|
Zeiler et al., 2013 [162] |
8 |
35 |
100 |
50 |
3.9 |
32 |
|
Sheehan et al., 2013 [177] |
96 |
48 |
98 |
70 |
4 |
36 |
|
Marek et al., 2015 [176] |
26 |
78 |
90.9 at 5 and 10 years |
80.7 |
0 |
23 |
|
Cordeiro et al., 2018 [133] |
262 |
51 |
95.8 |
NA |
NA |
26.6 |
|
Knappe et al., 2020 [180] |
119 |
107 |
NA |
78 |
NA |
NA |
|
Gupta et al., 2018 [166] |
21 |
69.5 |
100 |
81% |
NA |
19.6% |
*time not specified; NA not assessed
In patients with prolactinomas treated with GK SRS the reported time to hormonal response ranged from 5 months to 40 months (Table 6) [14, 40, 55, 64, 109-111, 114, 121, 138-140, 142, 143, 145, 146, 148, 149, 156, 157, 161, 169, 181-186]. At a corrected median follow-up of 43 months (median range 6-60 months), 33% of patients had normalization of serum prolactin concentrations following GK SRS [14]. One study of 35 patients reported a hormonal normalization of 80% after a median of 96 months and a tumor control rate of 97% [184]. There is insufficient information to assess the rate of decline of prolactin following GK SRS in comparison to that following CRT.
Table 6. Summary of Results of Published Series on SRS for Prolactin Secreting Pituitary Adenomas |
|||||
Authors |
Number of patients |
Follow-up median (months) |
Hormone normalization*% |
Late toxicity (%) Visual Hypopituitarism |
|
Ganz et al., 1993 [169] |
3 |
18 |
0 |
0 |
NA |
Martinez et al., 1998 [109] |
5 |
26-45 |
0 |
0 |
0 |
Pan et al., 1998 [110] |
27 |
29 |
30 |
0 |
0 |
Morange-Ramos et al., 1998 [138] |
4 |
20 |
0 |
0 |
16 |
Lim et al., 1998 [139] |
19 |
26 |
50 |
NA |
NA |
Mokry et al., 1999 [111] |
21 |
31 |
57 |
0 |
19 |
Kim et al., 1999 [165] |
18 |
27 |
16 |
NA |
NA |
Hayashi et al., 1999 [142] |
13 |
>6 |
15 |
NA |
5 |
Inoue et al., 1999 [143] |
2 |
>24 |
50 |
0 |
0 |
Landolt et al., 2000 [181] |
20 |
29 |
25 |
0 |
NA |
Pan et al., 2000 [182] |
128 |
33 |
41 |
0 |
NA |
Izawa et al., 2000 [145] |
15 |
>6 |
16 |
0 |
NA |
Pollock et al., 2002 [146] |
7 |
26 |
29 |
14 |
16 |
Choi et al., 2003 [148] |
21 |
43 |
23 |
0 |
0 |
Jane et al., 2003 [149] |
19 |
>18 |
11 |
0 |
21 |
Petrovich et al., 2003 [114] |
12 |
36 |
83 |
0 |
NA |
Pouratian et al., 2006 [183] |
23 |
55 |
26 |
7 |
28 |
Jezkova et al., 2009 [184] |
35 |
96 |
80 |
NA |
NA |
Kobayashi, 2009 [121] |
27 |
37(mean) |
17 |
0 |
0 |
Wan et al., 2009 [156] |
176 |
60 (minimum) |
23 |
0 |
1.7 |
Castinetti et al., 2009 [157] |
15 |
60 (minimum) |
46 at 24 months |
1.3** |
23** |
Liu et al., 2013 [185] |
22 |
36 |
27 |
- |
4.5 |
Cohen-Inbar et al., 2015 [186] |
38 |
42.3 |
50 |
NA |
30.3 |
Ježková et al., 2019 [187] |
28 |
140 |
82.1 |
3.6 |
8.3 |
Early studies of linac based SRS reported results on small numbers of patients, but the available results are broadly equivalent to those reported for GK SRS [17]. In the largest linac based SRS study to date, which included 175 patients with both non-functioning and functioning pituitary adenomas treated using a single dose of 20 Gy, the local tumor control rate was 97% after a minimum of 12 months follow-up [188]. Actuarial 5-year PFS was not reported. Hormonal normalization rates were 47% for GH-secreting adenomas, 65% with Cushing’s disease, and 39% with prolactinomas. The mean time for hormone normalization was 36±24 months. Within the limited follow-up period, 12% developed additional pituitary dysfunction, 3% radiation-induced CNS tissue damage, and 1% radiation-induced optic neuropathy. These results from linac SRS are difficult to evaluate but are broadly similar to those achieved with GK SRS and appear inferior to those obtained with fractionated treatment.
TOXICITY
In common with other modalities of pituitary irradiation, the most commonly reported complication following GK SRS is hypopituitarism, with a crude incidence ranging from 0% to 66% [14, 55]; the actuarial incidence has not been defined. The expected frequency of visual complications would be low if GK SRS is only offered to patients with a pituitary adenoma at a safe distance from the optic chiasm and nerves (~ 5mm). However, one study in patients with Cushing’s disease reported a 10% incidence of new cranial nerve deficit, with a 6% incidence of optic neuropathy [155]. Similarly, a study reporting results of SRS for prolactinoma noted a 7% incidence of cranial nerve deficit [183]. Although the absolute numbers of patients treated in these studies of GK SRS were small, there is a suggestion that for some patients, possibly with larger tumors, the incidence of optic pathway toxicity with GK SRS is well above what is seen in patients following CRT. Long-term risks of cerebrovascular events and the incidence of second tumors following GK SRS are not yet defined. GK toxicity is expected to be higher when offered after surgical excision rather than as a primary treatment option. In a recently published systematic review and meta-analysis on 1381 patients with pituitary adenomas treated with GK SRS, rates of radiation-induced hypopituitarism were (11.4%) in primary GK compared to (18-32%) in post-operative GK SRS. This highlights the importance of long-term endocrinology follow-up [189].
Robotic SRS
A small number of retrospective case series on outcomes following CK SRS for pituitary adenomas have been published to date (Table 7) [190-197]. While the published results are comparable to the outcomes achieved with GK SRS, the same criticisms levelled at the GK SRS studies also apply to these early CK SRS series. The duration of follow-up in all the existing CK SRS series is too short to allow meaningful conclusions to be drawn with regard to both efficacy and toxicity outcomes.
Table 7. Summary of Results of Published Series on Cyberknife SRS for Functioning & Non-Functioning Pituitary Adenomas |
|
|||||||
Author |
Tumor type |
Number of patients |
Follow-up mean (months) |
Tumor Control or Hormone normalization* (%) |
Late toxicity (%) Visual Hypopituitarism |
|
||
Kajiwara et al., 2005 [190] |
14 NFA, 3 PRL, 2 GH, 2 ACTH |
21 |
35.3 |
95.2TC, 50 HN |
4.7 |
9.5 |
|
|
Adler et al., 2006 [191] |
12 NFA, 4 GH, 2 ACTH, 1 PRL |
19 |
46 |
18/19 TC |
5.2 |
NA |
|
|
Roberts et al., 2007 [192] |
GH |
9 |
25.4 |
44.4 HN |
0 |
33 |
|
|
Killory et al., 2009 [193] |
14 NFA, 4GH, 1 PRL, 1 TSH |
20 |
26.6 |
100 TC |
0 |
NA |
|
|
Cho et al., 2009 [194] |
17 NFA, 3 PRL, 6 GH |
26 |
30 |
92.3 TC, 44 HN |
7.6 |
0 |
|
|
Iwata et al., 2011 [195] |
NFA |
100 |
33 median |
98 TC |
1 |
4 |
|
|
Puataweepong et al., 2015[196] |
27 NFA, 7 GH, 5 PRL, 1 ACTH |
40 |
38.5 median |
97.5 TC, 54 HN |
0 |
0 |
|
|
Iwata et al., 2016 [197] |
GH |
52 |
60 median |
100 TC, 20.4 HN |
0 |
2.2 |
|
|
Plitt et al., 2019 [198] |
NFA |
53 |
32.5 |
98.1 TC |
0 |
1.9 |
|
|
Romero-Gameros et al., 2023 [199] |
GH |
57 |
48 |
45.6% HN |
0 |
24.5 |
||
TC: Tumor Control; HN: hormone normalization
Proton Beam Therapy
An early study, published in 1989, of proton beam therapy for pituitary adenomas attempted to compare the effectiveness of this treatment modality to RT/CRT [200]. Follow-up after CRT in 17 patients and after proton therapy in 13 patients found a similar reduction of GH levels in both groups and the small number of patients does not allow for any statistically meaningful comparison. Nevertheless, treatment related side effects, including new hypopituitarism and oculomotor palsies, were more frequent in proton therapy group. Since the efficacy of both pituitary irradiation methods were similar, but proton therapy was associated with a higher incidence of serious side effects, the authors concluded that RT/CRT is the preferred treatment modality [200].
In a study from 2006, of 47 patients treated with fractionated proton therapy for both non-functioning and functioning pituitary adenomas reported tumor stabilization in 41 (87%) patients after a minimum 6-month follow-up; 1 patient developed temporal lobe necrosis, 3 developed new significant visual deficits, and 11 developed new hypopituitarism [201]. These are disappointing results suggesting considerably worse outcome both in terms of efficacy and toxicity than seen with photon irradiation.
A study of proton beam stereotactic radiosurgery in 22 patients with acromegaly reported normalization of GH in 59% after a median of 42 months. New pituitary deficiency was reported in 38% of patients, but no visual complications were reported [43]. The same group reported on the biochemical response in a larger population of secreting adenomas (74 ACTH-secreting, 50 GH-secreting, 9 PRL-secreting, 8 Nelson’s syndromes, 3 TSH-secreting) treated with the same technique. The study included 27 patients previously irradiated (14 pts) or treated with fractionated proton beam radiotherapy. At a median follow-up of 52 months, 42% of patients did not achieve endocrine control with patients with acromegaly having the longer time to biochemical response (49% at 5 years). The risk of developing hypopituitarism was 62% at 5 years and four patients (3%) experienced post treatment temporal lobe seizures, with associated temporal lobe changes on imaging (1 month to 9 years from proton treatment). [202]).
An evidence-based review of proton therapy from ASTRO’s emerging technology committee examined the evidence for proton therapy across multiple tumor sites and concluded that currently available evidence provides only limited indications for proton therapy [203]). The report recommended that robust prospective clinical trials be conducted to determine the appropriate clinical indications for proton therapy. In the present context, the available published reports of proton therapy for pituitary adenoma demonstrate disappointing efficacy and increased toxicity relative to much more readily available photon-based treatment. Also, in dosimetric comparisons, proton beam did not provide superior dose coverage advantage over photon radiation with comparable doses to OARs with both modalities [204]. Therefore, it seems difficult to justify proton therapy to the pituitary outside of the context of a clinical trial.
RE-IRRADIATION FOR RECURRENT DISEASE
Re-irradiation for progression of pituitary adenoma after previous pituitary radiotherapy is considered to be associated with a high risk of radiation-induced damage due to the presumed cumulative effect of radiation to the optic apparatus, the cranial nerves, and the normal brain tissues. However, re-irradiation using fractionated conventional or stereotactic techniques is feasible, with acceptable toxicity [53], provided that there has been at least a 3-4 year gap following primary radiotherapy treatment to doses below radiation tolerance of the CNS (which is the case for the conventional dose of 45Gy delivered at <1.8Gy per fraction). GK SRS has also been used to re-irradiate small recurrent lesions, particularly if they are not in close proximity to the optic apparatus [205].
While the current impression is that late toxicity following pituitary re-irradiation is uncommon, a high incidence of adverse side effects (13% radiation induced optic neuropathy and 13% of temporal lobe necrosis) was reported in a series of 15 patients re-irradiated with both single fraction and fractionated approaches (median time from previous RT 5.8 years) [206]. Nonetheless, there are at present insufficient long-term data to demonstrate the safety of pituitary re-irradiation for recurrent disease, although the use of high precision techniques and fractionation should theoretically reduce late toxicity.
With the lack of consensus, variations in the management of pituitary recurrences are discussed in MDT meetings and decisions vary based on expertise and scope of practicing physicians. For example, in a survey study for Canadian neurosurgeons and radiation oncologists, it was observed that physicians newer to practice had a greater tendency to advocate for stereotactic radiosurgery (SRS) or re-resection (54.5% and 36.4%, respectively), as compared to older surgeons who showed a higher propensity (22.2%) to advocate for observation. The presence of cavernous sinus extension encouraged radiation oncologists to offer earlier radiotherapy sooner (61.4%), compared to 40% of neurosurgeons [207].
OUTLOOK
The techniques of pituitary radiotherapy have gradually evolved over a number of decades with apparent choice between different technologies. All technologies share the aim of concentrating the radiation dose to the tumor with minimal dose to surrounding tissue and the irradiation is given in one, few or many fractions. There has been a lack of randomized comparative studies comparing the techniques to date. Systematic review of case series reported in the literature assessing the efficacy and toxicity provides a reasonably objective assessment of the techniques. While prospective randomized trials would provide the best objective comparative information, the beliefs of practitioners in particular treatment modalities, vested interests in technologies, and general difficulty of conducting studies in diseases with such long natural history make such comparative trials an unlikely prospect. This is compounded by the fact that new radiotherapy technologies continue to be introduced into clinical practice without the need for establishing efficacy as demanded for new drugs. Therefore, controversy will persist with regard to the appropriate and optimal methods for treating pituitary adenomas using radiation, and that all of the treatment modalities described here will continue in clinical use for the foreseeable future despite systematic reviews suggesting that some of the techniques may be less effective and potentially more toxic.
Conformal techniques of fractionated pituitary radiotherapy are standard practice, with many centers able to offer the additional accuracy of higher precision radiotherapy previously termed stereotactic but currently part of mainstream high-precision RT. Successful application of high-precision treatment is highly dependent on expertise in accurate target definition using modern MR imaging, on the precision of the immobilization system based on an exhaustive quality assurance program, and on infrastructure particularly in the form of expertise of staff in complex techniques of treatment planning and delivery. It seems most likely that it is the available expertise at all levels of staff in a treatment center that is the principal determinant of the success of pituitary radiotherapy rather than the choice of equipment and the precise treatment technique that is used.
SUMMARY
Fractionated radiotherapy is an effective treatment for pituitary adenomas, able to achieve excellent disease control and normalization of hormone levels. While the overall safety profile of this treatment modality is favorable, it is not devoid of side effects and it should only be employed when the risks from the disease itself are considered to outweigh the risks from the treatment. The balance of risks should take into account not only the early consequences of the disease and treatment, measured in terms of disease control and immediate morbidity, but also the long-term effects, particularly in terms of the influence of treatment on survival and quality of life, both of which are less well defined.
Residual pituitary adenomas, most of which have an indolent natural history, pose little threat to function, unless they lie close to the optic apparatus, or unless they destructively invade adjacent structures, which is an uncommon event. The risks of residual adenoma are therefore often minimal, and in the absence of progression or hormone hypersecretion, there is currently little justification for adjuvant radiation, whether in the form of fractionated or single fraction treatment. However, a policy of postoperative surveillance does require a program of close monitoring, usually in the form of annual MR imaging, and proceeding to timely irradiation if necessary, and certainly well before the need for further surgery. The aim of radiation treatment is to arrest tumor growth without the risks of re-operation.
For functioning tumors radiation treatment is generally offered to patients with persistent hormone elevation that is not decreasing at the expected rate following previous intervention of surgery and medical therapy. This usually means persistent hormone elevation in patients with acromegaly, Cushing’s disease, and other functioning adenomas, regardless of how far the actual hormone level is from normal, as the aim in most cases is to achieve normalization. In patients with acromegaly treated with somatostatin analogues, the expense and inconvenience of protracted systemic treatment also warrants early radiation treatment to allow for the withdrawal of medical treatment. The alternative is to continue medical management indefinitely without radiotherapy. It is not clear at present which policy is associated with better long-term survival and quality of life, and this should ideally be the subject of a prospective randomized trial.
Current clinical practice is therefore to offer treatment to patients with progressive non-functioning (or functioning) pituitary adenomas considered to be a threat to function, and to patients with functioning pituitary adenomas with persistent hypersecretion. Fractionated radiotherapy, as high-precision IMRT (previously considered as SCRT/fSRT), is the current standard of care for patients requiring radiation treatment for pituitary adenoma. Single fraction radiosurgery can be considered to treat small adenomas away from critical structures in view of the significant risk of radiation-induced damage carried by a high single dose of radiation. Long-term follow-up data are needed to fully evaluate the clinical efficacy of single fraction radiosurgery in comparison with fractionated radiotherapy.
ACKNOWLEDGEMENTS
MK and NF would like to thank Dr Francesca Solda, Dr Liam Welsh, Dr Thankamma Ajithkumar and Professor Michael Brada who authored previous versions of this review. MK is funded by the NIHR Biomedical Research Centre at University College London Hospitals NHS Foundation Trust and University College London.
REFERENCES
- Surawicz, T.S., et al., Descriptive epidemiology of primary brain and CNS tumors: results from the Central Brain Tumor Registry of the United States, 1990-1994. Neuro Oncol, 1999. 1(1): p. 14-25.
- Ezzat, S., et al., The prevalence of pituitary adenomas: a systematic review. Cancer, 2004. 101(3): p. 613-9.
- Beclere, J., The radiotherapeutic treatment of tumours of the hypophysis, gigantism and acromegaly. Archives of the Roentgen Ray, 1909. III(1): p. 8.
- Turner, H.E., et al., Audit of selected patients with nonfunctioning pituitary adenomas treated without irradiation - a follow-up study. Clin Endocrinol (Oxf), 1999. 51(3): p. 281-4.
- Gittoes, N.J., et al., Radiotherapy for non-function pituitary tumours. Clin Endocrinol (Oxf), 1998. 48(3): p. 331-7.
- Grigsby, P.W., et al., Prognostic factors and results of surgery and postoperative irradiation in the management of pituitary adenomas. Int J Radiat Oncol Biol Phys, 1989. 16(6): p. 1411-7.
- McCollough, W.M., et al., Long-term follow-up of radiotherapy for pituitary adenoma: the absence of late recurrence after greater than or equal to 4500 cGy. Int J Radiat Oncol Biol Phys, 1991. 21(3): p. 607-14.
- Brada, M., et al., The long-term efficacy of conservative surgery and radiotherapy in the control of pituitary adenomas. Clin Endocrinol (Oxf), 1993. 38(6): p. 571-8.
- Tsang, R.W., et al., Radiation therapy for pituitary adenoma: treatment outcome and prognostic factors. Int J Radiat Oncol Biol Phys, 1994. 30(3): p. 557-65.
- Zierhut, D., et al., External radiotherapy of pituitary adenomas. Int J Radiat Oncol Biol Phys, 1995. 33(2): p. 307-14.
- Rush, S. and P.R. Cooper, Symptom resolution, tumor control, and side effects following postoperative radiotherapy for pituitary macroadenomas. Int J Radiat Oncol Biol Phys, 1997. 37(5): p. 1031-4.
- Breen, P., et al., Radiotherapy for nonfunctional pituitary adenoma: analysis of long-term tumor control. J Neurosurg, 1998. 89(6): p. 933-8.
- Sasaki, R., et al., The efficacy of conventional radiation therapy in the management of pituitary adenoma. Int J Radiat Oncol Biol Phys, 2000. 47(5): p. 1337-45.
- Minniti, G., D.C. Gilbert, and M. Brada, Modern techniques for pituitary radiotherapy. Rev Endocr Metab Disord, 2009. 10(2): p. 135-44.
- Khoo, V.S., et al., Comparison of intensity-modulated tomotherapy with stereotactically guided conformal radiotherapy for brain tumors. Int J Radiat Oncol Biol Phys, 1999. 45(2): p. 415-25.
- Karger, C.P., et al., Three-dimensional accuracy and interfractional reproducibility of patient fixation and positioning using a stereotactic head mask system. Int J Radiat Oncol Biol Phys, 2001. 49(5): p. 1493-504.
- Ajithkumar, T. and M. Brada, Stereotactic linear accelerator radiotherapy for pituitary tumors. Treat Endocrinol, 2004. 3(4): p. 211-6.
- Leksell, L., The stereotaxic method and radiosurgery of the brain. Acta Chir Scand, 1951. 102(4): p. 316-9.
- Levy, R., A Short History of Stereotactic Neurosurgery. Cyber Museum of neurosurgery. 1999. Available from: http://www.neurosurgery.org/cybermuseum/stereotactichall/stereoarticle.html.
- Lasak, J.M. and J.P. Gorecki, The history of stereotactic radiosurgery and radiotherapy. Otolaryngol Clin North Am, 2009. 42(4): p. 593-9.
- Gill, S.S., et al., Relocatable frame for stereotactic external beam radiotherapy. Int J Radiat Oncol Biol Phys, 1991. 20(3): p. 599-603.
- Graham, J.D., et al., A non-invasive, relocatable stereotactic frame for fractionated radiotherapy and multiple imaging. Radiother Oncol, 1991. 21(1): p. 60-2.
- Adler, J.R., Jr., et al., The Cyberknife: a frameless robotic system for radiosurgery. Stereotact Funct Neurosurg, 1997. 69(1-4 Pt 2): p. 124-8.
- Steel, G.G., Basic Clinical Radiobiology. 3 ed. Vol. Arnold. 2002, London.
- Larson, D.A., J.C. Flickinger, and J.S. Loeffler, The radiobiology of radiosurgery. Int J Radiat Oncol Biol Phys, 1993. 25(3): p. 557-61.
- Park, C., et al., Universal survival curve and single fraction equivalent dose: useful tools in understanding potency of ablative radiotherapy. Int J Radiat Oncol Biol Phys, 2008. 70(3): p. 847-52.
- Lawrence, Y.R., et al., Radiation dose-volume effects in the brain. Int J Radiat Oncol Biol Phys, 2010. 76(3 Suppl): p. S20-7.
- Mayo, C., et al., Radiation dose-volume effects of optic nerves and chiasm. Int J Radiat Oncol Biol Phys, 2010. 76(3 Suppl): p. S28-35.
- Khattab, M.H., et al., Stereotactic Radiosurgery and Hypofractionated Stereotactic Radiotherapy for Nonfunctioning Pituitary Adenoma. J Neurol Surg B Skull Base, 2021. 82(Suppl 3): p. e51-e58.
- Rosenfelder, N.A., et al., Achieving the relocation accuracy of stereotactic frame-based cranial radiotherapy in a three-point thermoplastic shell. Clin Oncol (R Coll Radiol), 2013. 25(1): p. 66-73.
- Kumar, S., et al., Treatment accuracy of fractionated stereotactic radiotherapy. Radiother Oncol, 2005. 74(1): p. 53-9.
- Veselsky, T., et al., Pituitary adenoma treatment plan quality comparison between linear accelerator volumetric modulated arc therapy and Leksell Gamma Knife(R) radiosurgery. Med Dosim, 2021. 46(4): p. 440-448.
- Sayer, F.T., et al., Initial experience with the eXtend System: a relocatable frame system for multiple-session gamma knife radiosurgery. World Neurosurg, 2011. 75(5-6): p. 665-72.
- Zhao, K., et al., Fractionated Gamma Knife surgery for giant pituitary adenomas. Clin Neurol Neurosurg, 2016. 150: p. 139-142.
- Ikeda, H., H. Jokura, and T. Yoshimoto, Gamma knife radiosurgery for pituitary adenomas: usefulness of combined transsphenoidal and gamma knife radiosurgery for adenomas invading the cavernous sinus. Radiat Oncol Investig, 1998. 6(1): p. 26-34.
- Hayashi, M., et al., Gamma Knife robotic microradiosurgery of pituitary adenomas invading the cavernous sinus: treatment concept and results in 89 cases. J Neurooncol, 2010. 98(2): p. 185-94.
- Kim, E.H., et al., Postoperative Gamma Knife Radiosurgery for Cavernous Sinus-Invading Growth Hormone-Secreting Pituitary Adenomas. World Neurosurg, 2018. 110: p. e534-e545.
- Hayashi, M., et al., Gamma Knife Radiosurgery for Pituitary Adenomas Invading the Cavernous Sinus: Tokyo Women's Medical University Experience. Acta Neurochir Suppl, 2021. 128: p. 29-41.
- Lee, W.J., et al., Gamma Knife Radiosurgery as a Primary Treatment for Nonfunctioning Pituitary Adenoma Invading the Cavernous Sinus. Stereotact Funct Neurosurg, 2020. 98(6): p. 371-377.
- Sheehan, J.P., et al., Gamma Knife surgery for pituitary adenomas: factors related to radiological and endocrine outcomes. J Neurosurg, 2011. 114(2): p. 303-9.
- Grant, R.A., et al., Efficacy and safety of higher dose stereotactic radiosurgery for functional pituitary adenomas: a preliminary report. World Neurosurg, 2014. 82(1-2): p. 195-201.
- Kjellberg, R.N., et al., Proton-beam therapy in acromegaly. N Engl J Med, 1968. 278(13): p. 689-95.
- Petit, J.H., et al., Proton stereotactic radiosurgery in management of persistent acromegaly. Endocr Pract, 2007. 13(7): p. 726-34.
- Durante, M. and J.S. Loeffler, Charged particles in radiation oncology. Nat Rev Clin Oncol, 2010. 7(1): p. 37-43.
- Specialised Commissioning Team, N.E. B01/P/c Proton Beam Therapy for Cancer in Teenagers and Young Adults 2015; Available from: https://www.england.nhs.uk/commissioning/wp-content/uploads/sites/12/2015/10/b01-pc-prtn-bm-thrpy-teens-yng-oct15.pdf.
- Raverot, G., et al., European Society of Endocrinology Clinical Practice Guidelines for the management of aggressive pituitary tumours and carcinomas. Eur J Endocrinol, 2018. 178(1): p. G1-G24.
- Giuffrida, G., et al., Peptide receptor radionuclide therapy for aggressive pituitary tumors: a monocentric experience. Endocr Connect, 2019. 8(5): p. 528-535.
- Maclean, J., et al., Peptide receptor radionuclide therapy for aggressive atypical pituitary adenoma/carcinoma: variable clinical response in preliminary evaluation. Pituitary, 2014. 17(6): p. 530-8.
- Page, R.C., et al., An account of the quality of life of patients after treatment for non-functioning pituitary tumours. Clin Endocrinol (Oxf), 1997. 46(4): p. 401-6.
- van Beek, A.P., et al., Radiotherapy is not associated with reduced quality of life and cognitive function in patients treated for nonfunctioning pituitary adenoma. Int J Radiat Oncol Biol Phys, 2007. 68(4): p. 986-91.
- Yang, C.J., et al., Symptom distress and quality of life after stereotactic radiosurgery in patients with pituitary tumors: a questionnaire survey. PLoS One, 2014. 9(2): p. e88460.
- Jenkins, P.J., et al., Conventional pituitary irradiation is effective in lowering serum growth hormone and insulin-like growth factor-I in patients with acromegaly. J Clin Endocrinol Metab, 2006. 91(4): p. 1239-45.
- Brada, M. and P. Jankowska, Radiotherapy for pituitary adenomas. Endocrinol Metab Clin North Am, 2008. 37(1): p. 263-75, xi.
- Rowland, N.C. and M.K. Aghi, Radiation treatment strategies for acromegaly. Neurosurg Focus, 2010. 29(4): p. E12.
- Brada, M., T.V. Ajithkumar, and G. Minniti, Radiosurgery for pituitary adenomas. Clin Endocrinol (Oxf), 2004. 61(5): p. 531-43.
- Estrada, J., et al., The long-term outcome of pituitary irradiation after unsuccessful transsphenoidal surgery in Cushing's disease. N Engl J Med, 1997. 336(3): p. 172-7.
- Barrande, G., et al., Hormonal and metabolic effects of radiotherapy in acromegaly: long-term results in 128 patients followed in a single center. J Clin Endocrinol Metab, 2000. 85(10): p. 3779-85.
- Biermasz, N.R., H.V. Dulken, and F. Roelfsema, Postoperative radiotherapy in acromegaly is effective in reducing GH concentration to safe levels. Clin Endocrinol (Oxf), 2000. 53(3): p. 321-7.
- Epaminonda, P., et al., Efficacy of radiotherapy in normalizing serum IGF-I, acid-labile subunit (ALS) and IGFBP-3 levels in acromegaly. Clin Endocrinol (Oxf), 2001. 55(2): p. 183-9.
- Minniti, G., et al., The long-term efficacy of conventional radiotherapy in patients with GH-secreting pituitary adenomas. Clin Endocrinol (Oxf), 2005. 62(2): p. 210-6.
- Langsenlehner, T., et al., Long-term follow-up of patients with pituitary macroadenomas after postoperative radiation therapy: analysis of tumor control and functional outcome. Strahlenther Onkol, 2007. 183(5): p. 241-7.
- Minniti, G., et al., Long-term follow-up results of postoperative radiation therapy for Cushing's disease. J Neurooncol, 2007. 84(1): p. 79-84.
- Rim, C.H., et al., Radiotherapy for pituitary adenomas: long-term outcome and complications. Radiat Oncol J, 2011. 29(3): p. 156-63.
- Ajithkumar, T.B., M., Pituitary radiotherapy. Oxford Textbook of Endocrinology and Diabetes. 2011: Oxford Press University.
- Kim, M.Y., et al., Long-term outcomes of surgery and radiotherapy for secreting and non-secreting pituitary adenoma. Radiation Oncology Journal, 2016. 34(2): p. 121-127.
- Patt, H., et al., High-Precision Conformal Fractionated Radiotherapy Is Effective in Achieving Remission in Patients with Acromegaly after Failed Transsphenoidal Surgery. Endocr Pract, 2016. 22(2): p. 162-72.
- Hussein, Z., et al., Non-functioning pituitary macroadenoma following surgery: long-term outcomes and development of an optimal follow-up strategy. Front Surg, 2023. 10: p. 1129387.
- Arnardottir, S., et al., Long-term outcomes of patients with acromegaly: a report from the Swedish Pituitary Register. Eur J Endocrinol, 2022. 186(3): p. 329-339.
- Tsagarakis, S., et al., Megavoltage pituitary irradiation in the management of prolactinomas: long-term follow-up. Clin Endocrinol (Oxf), 1991. 34(5): p. 399-406.
- Johnston, D.G., et al., The long-term effects of megavoltage radiotherapy as sole or combined therapy for large prolactinomas: studies with high definition computerized tomography. Clin Endocrinol (Oxf), 1986. 24(6): p. 675-85.
- Mehta, A.E., F.I. Reyes, and C. Faiman, Primary radiotherapy of prolactinomas. Eight- to 15-year follow-up. Am J Med, 1987. 83(1): p. 49-58.
- Becker, G., et al., Radiation therapy in the multimodal treatment approach of pituitary adenoma. Strahlenther Onkol, 2002. 178(4): p. 173-86.
- Sherlock, M., et al., Mortality in patients with pituitary disease. Endocr Rev, 2010. 31(3): p. 301-42.
- Brada, M., et al., Cerebrovascular mortality in patients with pituitary adenoma. Clin Endocrinol (Oxf), 2002. 57(6): p. 713-7.
- Brada, M., et al., The incidence of cerebrovascular accidents in patients with pituitary adenoma. Int J Radiat Oncol Biol Phys, 1999. 45(3): p. 693-8.
- Tomlinson, J.W., et al., Association between premature mortality and hypopituitarism. West Midlands Prospective Hypopituitary Study Group. Lancet, 2001. 357(9254): p. 425-31.
- Erfurth, E.M., et al., Risk factors for cerebrovascular deaths in patients operated and irradiated for pituitary tumors. J Clin Endocrinol Metab, 2002. 87(11): p. 4892-9.
- Fajardo, L.F., The pathology of ionizing radiation as defined by morphologic patterns. Acta Oncol, 2005. 44(1): p. 13-22.
- Bliss, P., G.R. Kerr, and A. Gregor, Incidence of second brain tumours after pituitary irradiation in Edinburgh 1962-1990. Clin Oncol (R Coll Radiol), 1994. 6(6): p. 361-3.
- Tsang, R.W., et al., Glioma arising after radiation therapy for pituitary adenoma. A report of four patients and estimation of risk. Cancer, 1993. 72(7): p. 2227-33.
- Brada, M., et al., Risk of second brain tumour after conservative surgery and radiotherapy for pituitary adenoma. BMJ, 1992. 304(6838): p. 1343-6.
- Hamblin, R., et al., Risk of second brain tumour after radiotherapy for pituitary adenoma or craniopharyngioma: a retrospective, multicentre, cohort study of 3679 patients with long-term imaging surveillance. Lancet Diabetes Endocrinol, 2022. 10(8): p. 581-588.
- Peace, K.A., et al., Cognitive dysfunction in patients with pituitary tumour who have been treated with transfrontal or transsphenoidal surgery or medication. Clin Endocrinol (Oxf), 1998. 49(3): p. 391-6.
- Guinan, E.M., et al., Cognitive effects of pituitary tumours and their treatments: two case studies and an investigation of 90 patients. J Neurol Neurosurg Psychiatry, 1998. 65(6): p. 870-6.
- McCord, M.W., et al., Radiotherapy for pituitary adenoma: long-term outcome and sequelae. Int J Radiat Oncol Biol Phys, 1997. 39(2): p. 437-44.
- Brummelman, P., et al., Cognitive functioning in patients treated for nonfunctioning pituitary macroadenoma and the effects of pituitary radiotherapy. Clin Endocrinol (Oxf), 2011. 74(4): p. 481-7.
- Brummelman, P., et al., Cognitive performance after postoperative pituitary radiotherapy: a dosimetric study of the hippocampus and the prefrontal cortex. Eur J Endocrinol, 2012. 166(2): p. 171-9.
- Coke, C., et al., Multiple fractionated stereotactic radiotherapy of residual pituitary macroadenomas: initial experience. Stereotact Funct Neurosurg, 1997. 69(1-4 Pt 2): p. 183-90.
- Mitsumori, M., et al., Initial clinical results of LINAC-based stereotactic radiosurgery and stereotactic radiotherapy for pituitary adenomas. Int J Radiat Oncol Biol Phys, 1998. 42(3): p. 573-80.
- Milker-Zabel, S., et al., Fractionated stereotactically guided radiotherapy and radiosurgery in the treatment of functional and nonfunctional adenomas of the pituitary gland. Int J Radiat Oncol Biol Phys, 2001. 50(5): p. 1279-86.
- Paek, S.H., et al., Integration of surgery with fractionated stereotactic radiotherapy for treatment of nonfunctioning pituitary macroadenomas. Int J Radiat Oncol Biol Phys, 2005. 61(3): p. 795-808.
- Colin, P., et al., Treatment of pituitary adenomas by fractionated stereotactic radiotherapy: a prospective study of 110 patients. Int J Radiat Oncol Biol Phys, 2005. 62(2): p. 333-41.
- Minniti, G., et al., Fractionated stereotactic conformal radiotherapy for secreting and nonsecreting pituitary adenomas. Clin Endocrinol (Oxf), 2006. 64(5): p. 542-8.
- Selch, M.T., et al., Stereotactic radiotherapy for the treatment of pituitary adenomas. Minim Invasive Neurosurg, 2006. 49(3): p. 150-5.
- Kong, D.S., et al., The efficacy of fractionated radiotherapy and stereotactic radiosurgery for pituitary adenomas: long-term results of 125 consecutive patients treated in a single institution. Cancer, 2007. 110(4): p. 854-60.
- Snead, F.E., et al., Long-term outcomes of radiotherapy for pituitary adenomas. Int J Radiat Oncol Biol Phys, 2008. 71(4): p. 994-8.
- Roug, S., et al., Fractionated stereotactic radiotherapy in patients with acromegaly: an interim single-centre audit. Eur J Endocrinol, 2010. 162(4): p. 685-94.
- Schalin-Jantti, C., et al., Outcome of fractionated stereotactic radiotherapy in patients with pituitary adenomas resistant to conventional treatments: a 5.25-year follow-up study. Clin Endocrinol (Oxf), 2010. 73(1): p. 72-7.
- Weber, D.C., et al., Adjuvant or radical fractionated stereotactic radiotherapy for patients with pituitary functional and nonfunctional macroadenoma. Radiat Oncol, 2011. 6: p. 169.
- Wilson, P.J., et al., A single centre's experience of stereotactic radiosurgery and radiotherapy for non-functioning pituitary adenomas with the Linear Accelerator (Linac). J Clin Neurosci, 2012. 19(3): p. 370-4.
- Kim, J.O., et al., Long-term outcomes of fractionated stereotactic radiation therapy for pituitary adenomas at the BC Cancer Agency. Int J Radiat Oncol Biol Phys, 2013. 87(3): p. 528-33.
- Kopp, C., et al., Fractionated stereotactic radiotherapy in the treatment of pituitary adenomas. Strahlenther Onkol, 2013. 189(11): p. 932-7.
- Minniti, G., et al., Fractionated stereotactic radiotherapy for large and invasive non-functioning pituitary adenomas: long-term clinical outcomes and volumetric MRI assessment of tumor response. Eur J Endocrinol, 2015. 172(4): p. 433-41.
- Diallo, A.M., et al., Long-term results of fractionated stereotactic radiotherapy as third-line treatment in acromegaly. Endocrine, 2015. 50(3): p. 741-8.
- Barber, S.M., B.S. Teh, and D.S. Baskin, Fractionated Stereotactic Radiotherapy for Pituitary Adenomas: Single-Center Experience in 75 Consecutive Patients. Neurosurgery, 2016. 79(3): p. 406-17.
- Liao, H.I., et al., Fractionated stereotactic radiosurgery using the Novalis system for the management of pituitary adenomas close to the optic apparatus. J Clin Neurosci, 2014. 21(1): p. 111-5.
- Puataweepong, P., et al., Outcomes for Pituitary Adenoma Patients Treated with Linac- Based Stereotactic Radiosurgery and Radiotherapy: a Long Term Experience in Thailand. Asian Pac J Cancer Prev, 2015. 16(13): p. 5279-84.
- Lian, X., et al., Intensity-modulated Radiotherapy for Pituitary Somatotroph Adenomas. J Clin Endocrinol Metab, 2020. 105(12).
- Martinez, R., et al., Pituitary tumors and gamma knife surgery. Clinical experience with more than two years of follow-up. Stereotact Funct Neurosurg, 1998. 70 Suppl 1: p. 110-8.
- Pan, L., et al., Pituitary adenomas: the effect of gamma knife radiosurgery on tumor growth and endocrinopathies. Stereotact Funct Neurosurg, 1998. 70 Suppl 1: p. 119-26.
- Mokry, M., et al., A six year experience with the postoperative radiosurgical management of pituitary adenomas. Stereotact Funct Neurosurg, 1999. 72 Suppl 1: p. 88-100.
- Sheehan, J.P., et al., Radiosurgery for residual or recurrent nonfunctioning pituitary adenoma. J Neurosurg, 2002. 97(5 Suppl): p. 408-14.
- Wowra, B. and W. Stummer, Efficacy of gamma knife radiosurgery for nonfunctioning pituitary adenomas: a quantitative follow up with magnetic resonance imaging-based volumetric analysis. J Neurosurg, 2002. 97(5 Suppl): p. 429-32.
- Petrovich, Z., et al., Gamma knife radiosurgery for pituitary adenoma: early results. Neurosurgery, 2003. 53(1): p. 51-9; discussion 59-61.
- Pollock, B.E. and P.C. Carpenter, Stereotactic radiosurgery as an alternative to fractionated radiotherapy for patients with recurrent or residual nonfunctioning pituitary adenomas. Neurosurgery, 2003. 53(5): p. 1086-91; discussion 1091-4.
- Losa, M., et al., Gamma knife surgery for treatment of residual nonfunctioning pituitary adenomas after surgical debulking. J Neurosurg, 2004. 100(3): p. 438-44.
- Iwai, Y., K. Yamanaka, and K. Yoshioka, Radiosurgery for nonfunctioning pituitary adenomas. Neurosurgery, 2005. 56(4): p. 699-705; discussion 699-705.
- Mingione, V., et al., Gamma surgery in the treatment of nonsecretory pituitary macroadenoma. J Neurosurg, 2006. 104(6): p. 876-83.
- Liscak, R., et al., Gamma knife radiosurgery for endocrine-inactive pituitary adenomas. Acta Neurochir (Wien), 2007. 149(10): p. 999-1006; discussion 1006.
- Pollock, B.E., et al., Pituitary tumor type affects the chance of biochemical remission after radiosurgery of hormone-secreting pituitary adenomas. Neurosurgery, 2008. 62(6): p. 1271-6; discussion 1276-8.
- Kobayashi, T., Long-term results of stereotactic gamma knife radiosurgery for pituitary adenomas. Specific strategies for different types of adenoma. Prog Neurol Surg, 2009. 22: p. 77-95.
- Gopalan, R., et al., Long-term outcomes after Gamma Knife radiosurgery for patients with a nonfunctioning pituitary adenoma. Neurosurgery, 2011. 69(2): p. 284-93.
- Runge, M.J., et al., LINAC-radiosurgery for nonsecreting pituitary adenomas. Long-term results. Strahlenther Onkol, 2012. 188(4): p. 319-25.
- Park, K.J., et al., Long-term outcomes after gamma knife stereotactic radiosurgery for nonfunctional pituitary adenomas. Neurosurgery, 2011. 69(6): p. 1188-99.
- Starke, R.M., et al., Gamma Knife surgery for patients with nonfunctioning pituitary macroadenomas: predictors of tumor control, neurological deficits, and hypopituitarism. J Neurosurg, 2012. 117(1): p. 129-35.
- El-Shehaby, A.M., et al., Low-dose Gamma Knife surgery for nonfunctioning pituitary adenomas. J Neurosurg, 2012. 117 Suppl: p. 84-8.
- Sheehan, J.P., et al., Gamma Knife radiosurgery for the management of nonfunctioning pituitary adenomas: a multicenter study. J Neurosurg, 2013. 119(2): p. 446-56.
- Lee, C.C., et al., Initial Gamma Knife radiosurgery for nonfunctioning pituitary adenomas. J Neurosurg, 2014. 120(3): p. 647-54.
- Xu, Z., et al., Silent corticotroph adenomas after stereotactic radiosurgery: a case-control study. Int J Radiat Oncol Biol Phys, 2014. 90(4): p. 903-10.
- Hasegawa, T., et al., Stereotactic Radiosurgery as the Initial Treatment for Patients with Nonfunctioning Pituitary Adenomas. World Neurosurg, 2015. 83(6): p. 1173-9.
- Graffeo, C.S., et al., Hypopituitarism After Single-Fraction Pituitary Adenoma Radiosurgery: Dosimetric Analysis Based on Patients Treated Using Contemporary Techniques. Int J Radiat Oncol Biol Phys, 2018. 101(3): p. 618-623.
- Oh, J.W., et al., Hypopituitarism after Gamma Knife surgery for postoperative nonfunctioning pituitary adenoma. J Neurosurg, 2018. 129(Suppl1): p. 47-54.
- Cordeiro, D., et al., Hypopituitarism after Gamma Knife radiosurgery for pituitary adenomas: a multicenter, international study. J Neurosurg, 2018.
- Narayan, V., et al., Long-Term Outcome of Nonfunctioning and Hormonal Active Pituitary Adenoma After Gamma Knife Radiosurgery. World Neurosurg, 2018. 114: p. e824-e832.
- Slavinsky, P., et al., Gamma knife radiosurgery in pituitary adenomas. A single-center experience. Medicina (B Aires), 2022. 82(1): p. 111-116.
- Maldar, A.N., et al., Long-term hormonal and imaging outcomes of adjunctive gamma knife radiosurgery in non-functioning pituitary adenomas: a single center experience. J Neurooncol, 2022. 158(3): p. 423-433.
- Thoren, M., et al., Stereotactic radiosurgery with the cobalt-60 gamma unit in the treatment of growth hormone-producing pituitary tumors. Neurosurgery, 1991. 29(5): p. 663-8.
- Morange-Ramos, I., et al., Short-term endocrinological results after gamma knife surgery of pituitary adenomas. Stereotact Funct Neurosurg, 1998. 70 Suppl 1: p. 127-38.
- Lim, Y.L., et al., Four years' experiences in the treatment of pituitary adenomas with gamma knife radiosurgery. Stereotact Funct Neurosurg, 1998. 70 Suppl 1: p. 95-109.
- Kim, M.S., S.I. Lee, and J.H. Sim, Gamma Knife radiosurgery for functioning pituitary microadenoma. Stereotact Funct Neurosurg, 1999. 72 Suppl 1: p. 119-24.
- Landolt, A.M., et al., Stereotactic radiosurgery for recurrent surgically treated acromegaly: comparison with fractionated radiotherapy. J Neurosurg, 1998. 88(6): p. 1002-8.
- Hayashi, M., et al., Gamma Knife radiosurgery for pituitary adenomas. Stereotact Funct Neurosurg, 1999. 72 Suppl 1: p. 111-8.
- Inoue, H.K., et al., Pituitary adenomas treated by microsurgery with or without Gamma Knife surgery: experience in 122 cases. Stereotact Funct Neurosurg, 1999. 72 Suppl 1: p. 125-31.
- Zhang, N., et al., Radiosurgery for growth hormone-producing pituitary adenomas. J Neurosurg, 2000. 93 Suppl 3: p. 6-9.
- Izawa, M., et al., Gamma knife radiosurgery for pituitary adenomas. J Neurosurg, 2000. 93 Suppl 3: p. 19-22.
- Pollock, B.E., et al., Results of stereotactic radiosurgery in patients with hormone-producing pituitary adenomas: factors associated with endocrine normalization. J Neurosurg, 2002. 97(3): p. 525-30.
- Attanasio, R., et al., Gamma-knife radiosurgery in acromegaly: a 4-year follow-up study. J Clin Endocrinol Metab, 2003. 88(7): p. 3105-12.
- Choi, J.Y., et al., Radiological and hormonal responses of functioning pituitary adenomas after gamma knife radiosurgery. Yonsei Med J, 2003. 44(4): p. 602-7.
- Jane, J.A., Jr. and E.R. Laws, Jr., The management of non-functioning pituitary adenomas. Neurol India, 2003. 51(4): p. 461-5.
- Castinetti, F., et al., Outcome of gamma knife radiosurgery in 82 patients with acromegaly: correlation with initial hypersecretion. J Clin Endocrinol Metab, 2005. 90(8): p. 4483-8.
- Gutt, B., et al., Gamma-knife surgery is effective in normalising plasma insulin-like growth factor I in patients with acromegaly. Exp Clin Endocrinol Diabetes, 2005. 113(4): p. 219-24.
- Kobayashi, T., et al., Long-term results of gamma knife surgery for growth hormone-producing pituitary adenoma: is the disease difficult to cure? J Neurosurg, 2005. 102 Suppl: p. 119-23.
- Jezkova, J., et al., Gamma knife radiosurgery for acromegaly--long-term experience. Clin Endocrinol (Oxf), 2006. 64(5): p. 588-95.
- Pollock, B.E., et al., Radiosurgery of growth hormone-producing pituitary adenomas: factors associated with biochemical remission. J Neurosurg, 2007. 106(5): p. 833-8.
- Jagannathan, J., et al., Stereotactic radiosurgery for pituitary adenomas: a comprehensive review of indications, techniques and long-term results using the Gamma Knife. J Neurooncol, 2009. 92(3): p. 345-56.
- Wan, H., O. Chihiro, and S. Yuan, MASEP gamma knife radiosurgery for secretory pituitary adenomas: experience in 347 consecutive cases. J Exp Clin Cancer Res, 2009. 28: p. 36.
- Castinetti, F., et al., Long-term results of stereotactic radiosurgery in secretory pituitary adenomas. J Clin Endocrinol Metab, 2009. 94(9): p. 3400-7.
- Iwai, Y., et al., Gamma knife radiosurgery for growth hormone-producing adenomas. J Clin Neurosci, 2010. 17(3): p. 299-304.
- Erdur, F.M., et al., Gammaknife radiosurgery in patients with acromegaly. J Clin Neurosci, 2011. 18(12): p. 1616-20.
- Franzin, A., et al., Results of gamma knife radiosurgery in acromegaly. Int J Endocrinol, 2012. 2012: p. 342034.
- Liu, X., et al., Gamma knife radiosurgery for clinically persistent acromegaly. J Neurooncol, 2012. 109(1): p. 71-9.
- Zeiler, F.A., et al., Gamma knife in the treatment of pituitary adenomas: results of a single center. Can J Neurol Sci, 2013. 40(4): p. 546-52.
- Lee, C.C., et al., Stereotactic radiosurgery for acromegaly. J Clin Endocrinol Metab, 2014. 99(4): p. 1273-81.
- Jagannathan, J., et al., Gamma knife radiosurgery for acromegaly: outcomes after failed transsphenoidal surgery. Neurosurgery, 2008. 62(6): p. 1262-9; discussion 1269-70.
- Kim, S.H., et al., Gamma Knife radiosurgery for functioning pituitary adenomas. Stereotact Funct Neurosurg, 1999. 72 Suppl 1: p. 101-10.
- Gupta, A., et al., Upfront Gamma Knife radiosurgery for Cushing's disease and acromegaly: a multicenter, international study. J Neurosurg, 2018. 131(2): p. 532-538.
- Ding, D., et al., Stereotactic Radiosurgery for Acromegaly: An International Multicenter Retrospective Cohort Study. Neurosurgery, 2019. 84(3): p. 717-725.
- Degerblad, M., et al., Long-term results of stereotactic radiosurgery to the pituitary gland in Cushing's disease. Acta Endocrinol (Copenh), 1986. 112(3): p. 310-4.
- Ganz, J.C., E.O. Backlund, and F.A. Thorsen, The effects of Gamma Knife surgery of pituitary adenomas on tumor growth and endocrinopathies. Stereotact Funct Neurosurg, 1993. 61 Suppl 1: p. 30-7.
- Seo, Y., et al., Gamma knife surgery for Cushing's disease. Surg Neurol, 1995. 43(2): p. 170-5; discussion 175-6.
- Sheehan, J.M., et al., Radiosurgery for Cushing's disease after failed transsphenoidal surgery. J Neurosurg, 2000. 93(5): p. 738-42.
- Hoybye, C., et al., Adrenocorticotropic hormone-producing pituitary tumors: 12- to 22-year follow-up after treatment with stereotactic radiosurgery. Neurosurgery, 2001. 49(2): p. 284-91; discussion 291-2.
- Kobayashi, T., Y. Kida, and Y. Mori, Gamma knife radiosurgery in the treatment of Cushing disease: long-term results. J Neurosurg, 2002. 97(5 Suppl): p. 422-8.
- Devin, J.K., et al., The efficacy of linear accelerator radiosurgery in the management of patients with Cushing's disease. Stereotact Funct Neurosurg, 2004. 82(5-6): p. 254-62.
- Castinetti, F., et al., Gamma knife radiosurgery is a successful adjunctive treatment in Cushing's disease. Eur J Endocrinol, 2007. 156(1): p. 91-8.
- Marek, J., et al., Gamma knife radiosurgery for Cushing's disease and Nelson's syndrome. Pituitary, 2015. 18(3): p. 376-84.
- Sheehan, J.P., et al., Results of gamma knife surgery for Cushing's disease. J Neurosurg, 2013. 119(6): p. 1486-92.
- Sicignano, G., et al., Dosimetric factors associated with pituitary function after Gamma Knife Surgery (GKS) of pituitary adenomas. Radiother Oncol, 2012. 104(1): p. 119-24.
- Jagannathan, J., et al., Gamma Knife surgery for Cushing's disease. J Neurosurg, 2007. 106(6): p. 980-7.
- Knappe, U.J., et al., Fractionated radiotherapy and radiosurgery in acromegaly: analysis of 352 patients from the German Acromegaly Registry. Eur J Endocrinol, 2020. 182(3): p. 275-284.
- Landolt, A.M. and N. Lomax, Gamma knife radiosurgery for prolactinomas. J Neurosurg, 2000. 93 Suppl 3: p. 14-8.
- Pan, L., et al., Gamma knife radiosurgery as a primary treatment for prolactinomas. J Neurosurg, 2000. 93 Suppl 3: p. 10-3.
- Pouratian, N., et al., Gamma knife radiosurgery for medically and surgically refractory prolactinomas. Neurosurgery, 2006. 59(2): p. 255-66; discussion 255-66.
- Jezkova, J., et al., Use of the Leksell gamma knife in the treatment of prolactinoma patients. Clin Endocrinol (Oxf), 2009. 70(5): p. 732-41.
- Liu, X., et al., Gamma knife stereotactic radiosurgery for drug resistant or intolerant invasive prolactinomas. Pituitary, 2013. 16(1): p. 68-75.
- Cohen-Inbar, O., et al., Gamma Knife radiosurgery for medically and surgically refractory prolactinomas: long-term results. Pituitary, 2015. 18(6): p. 820-30.
- Jezkova, J., et al., Role of gamma knife radiosurgery in the treatment of prolactinomas. Pituitary, 2019. 22(4): p. 411-421.
- Voges, J., et al., Linear accelerator radiosurgery for pituitary macroadenomas: a 7-year follow-up study. Cancer, 2006. 107(6): p. 1355-64.
- Palmisciano, P., et al., Endocrine disorders after primary gamma knife radiosurgery for pituitary adenomas: A systematic review and meta-analysis. Pituitary, 2022. 25(3): p. 404-419.
- Kajiwara, K., et al., Image-guided stereotactic radiosurgery with the CyberKnife for pituitary adenomas. Minim Invasive Neurosurg, 2005. 48(2): p. 91-6.
- Adler, J.R., Jr., et al., Visual field preservation after multisession cyberknife radiosurgery for perioptic lesions. Neurosurgery, 2006. 59(2): p. 244-54; discussion 244-54.
- Roberts, B.K., et al., Efficacy and safety of CyberKnife radiosurgery for acromegaly. Pituitary, 2007. 10(1): p. 17.
- Killory, B.D., et al., Hypofractionated CyberKnife radiosurgery for perichiasmatic pituitary adenomas: early results. Neurosurgery, 2009. 64(2 Suppl): p. A19-25.
- Cho, C.B., et al., Stereotactic Radiosurgery with the CyberKnife for Pituitary Adenomas. J Korean Neurosurg Soc, 2009. 45(3): p. 157-63.
- Iwata, H., et al., Hypofractionated stereotactic radiotherapy with CyberKnife for nonfunctioning pituitary adenoma: high local control with low toxicity. Neuro Oncol, 2011. 13(8): p. 916-22.
- Puataweepong, P., et al., The Clinical Outcome of Hypofractionated Stereotactic Radiotherapy With CyberKnife Robotic Radiosurgery for Perioptic Pituitary Adenoma. Technol Cancer Res Treat, 2016. 15(6): p. NP10-NP15.
- Iwata, H., et al., Long-term results of hypofractionated stereotactic radiotherapy with CyberKnife for growth hormone-secreting pituitary adenoma: evaluation by the Cortina consensus. J Neurooncol, 2016. 128(2): p. 267-75.
- Plitt, A.R., et al., Fractionated CyberKnife Stereotactic Radiotherapy for Perioptic Pituitary Adenomas. World Neurosurg, 2019. 126: p. e1359-e1364.
- Romero-Gameros, C.A., et al., Efficiency and Safety of CyberKnife Robotic Radiosurgery in the Multimodal Management of Patients with Acromegaly. Cancers (Basel), 2023. 15(5).
- Ludecke, D.K., B.S. Lutz, and G. Niedworok, The choice of treatment after incomplete adenomectomy in acromegaly: proton--versus high voltage radiation. Acta Neurochir (Wien), 1989. 96(1-2): p. 32-8.
- Ronson, B.B., et al., Fractionated proton beam irradiation of pituitary adenomas. Int J Radiat Oncol Biol Phys, 2006. 64(2): p. 425-34.
- Wattson, D.A., et al., Outcomes of proton therapy for patients with functional pituitary adenomas. Int J Radiat Oncol Biol Phys, 2014. 90(3): p. 532-9.
- Allen, A.M., et al., An evidence based review of proton beam therapy: the report of ASTRO's emerging technology committee. Radiother Oncol, 2012. 103(1): p. 8-11.
- Sud, S., et al., Dosimetric Comparison of Proton Versus Photon Radiosurgery for Treatment of Pituitary Adenoma. Adv Radiat Oncol, 2021. 6(6): p. 100806.
- Edwards, A.A., F.M. Swords, and P.N. Plowman, Focal radiation therapy for patients with persistent/recurrent pituitary adenoma, despite previous radiotherapy. Pituitary, 2009. 12(1): p. 30-4.
- Verma, J., et al., Feasibility and outcome of re-irradiation in the treatment of multiply recurrent pituitary adenomas. Pituitary, 2014. 17(6): p. 539-45.
- Kasper, G., et al., Practice patterns in the management of recurrent and residual non-functioning pituitary adenomas: Results from a Canada-wide survey. eNeurologicalSci, 2021. 22: p. 100317.