ABSTRACT
The role of lipids and lipoproteins as causal factors for cardiovascular disease (CVD) is well established. Dietary saturated fatty acids (SFA), which are in milk, butter, cheese, beef, lamb, pork, poultry, palm oil, and coconut oil increase LDL-C and HDL-C. The increase in LDL-C is due to a decrease in hepatic LDL clearance and an increase in LDL production secondary to a decrease in hepatic LDL receptors. Monounsaturated fatty acids (MUFA) are in olive, canola, peanut, safflower, and sesame oil, and avocados, peanut butter, and many nuts and seeds and polyunsaturated fatty acids (PUFA) are in soybean, corn, and sunflower oil, and some nuts and seeds, tofu, and soybeans. Both MUFA and PUFA lower LDL-C by increasing hepatic LDL receptor activity. Dietary cholesterol is found in egg yolks, shrimp, beef, pork, poultry, cheese, and butter and increase LDL-C but the effect is modest and varies with approximately 15-25% of individuals being hyper-responders with more robust increases. Dietary cholesterol reduces hepatic LDL receptor activity, decreasing the clearance and increasing the production of LDL. Trans fatty acids (TFA) occur naturally in meat and dairy products and are formed during the partial hydrogenation of vegetable fat. TFA increase LDL-C and decrease HDL-C. Carbohydrates (CHO) can be divided into high-quality, for example fruits, legumes, vegetables, and whole grains, or low-quality, which include refined grains, starches, and added sugars. CHO increase TG with low quality CHO, particularly added sugars, having a more robust effect. Dietary CHO, particularly fructose, promotes hepatic de novo fatty acid synthesis leading to increased VLDL secretion. Fiber is found mostly in fruits, vegetables, whole and unrefined grains, nuts, seeds, beans, and legumes and phytosterols are naturally occurring constituents of plants and are found in vegetable oils, cereals, nuts, fruit and vegetables. Both dietary fiber and phytosterols decrease LDL-C by decreasing intestinal cholesterol absorption.
Summary of the Effect of Dietary Constituents on Lipid and Lipoproteins |
|
---|---|
SFA |
|
MUFA and PUFA |
Decrease LDL-C |
TFA |
Increase LDL-C and decrease HDL-C |
Cholesterol |
Increase LDL-C |
CHO |
Increase TGs particularly simple sugars |
Fiber |
Decrease LDL-C |
Phytosterols |
Decrease LDL-C |
With regards to CVD there are very few well conducted randomized controlled trials and most of the information is derived from observational studies that demonstrate associations. These observational studies have found that fruits, vegetables, beans/legumes, nuts/seeds, whole grains, fish, yogurt, fiber, seafood omega-3 fatty acids, and polyunsaturated fats were associated with a decreased risk of CVD while unprocessed red meats, processed meats, sugar-sweetened beverages, high glycemic load CHO, and trans-fats were associated with an increased risk of CVD. Randomized trials have shown that a Mediterranean diet reduces CVD. Based on this information current guidelines for the general population recommend 1. A diet emphasizing intake of vegetables, fruits, legumes, nuts, whole grains, and fish 2. Replacement of SFA with MUFA and PUFA 3. A reduced amount of dietary cholesterol 4. Minimizing intake of processed meats, refined CHO, and sweetened beverages and 5. Avoidance of TFA. For individuals with a high LDL-C limiting dietary SFA, TFA, and cholesterol and increasing fiber and phytosterols will help lower LDL-C while in individuals with high TG limiting low quality CHO, particularly simple sugars, and ethanol with weight loss, if indicated, will help lower TG.
INTRODUCTION
There is a huge literature describing the effect of diet on the risk of cardiovascular disease (CVD) and this literature is often conflicting and controversial. Several well recognized investigators have discussed the limitations of the information linking various diets and dietary constituents and the risk of disease (1,2). The major problem is that almost all of the information is based on observational studies and well conducted randomized trials measuring important cardiovascular outcomes are very rare. Observational studies can demonstrate associations but do not necessarily indicate that there is a cause-and-effect relationship. Unrecognized confounding variables can result in false associations. In several instances a robust association was observed in observation trials but randomized trials failed to confirm these observations (3). For example, several observational studies showed that higher vitamin E intake from dietary sources or supplements was associated with a lower risk of CVD (4-8), but randomized controlled trials failed to demonstrate a reduction in cardiovascular events with vitamin E supplementation (9-12). Observational studies have also reported that vitamin B6, B12, or folic acid intake reduced the risk of CVD (13-15), but again randomized controlled trials failed to demonstrate a benefit of increased vitamin intake on CVD (16-19). These results point to potential deficiencies in observational studies and the need to recognize that the associations demonstrated in observational studies may not always be causal. Therefore, in this chapter, where possible, we will focus on randomized controlled trials.
Moreover, even the interpretation of the results of observational trials is often debated. For example, a 2019 meta-analysis and systematic review published in the Annals of Internal Medicine reached the conclusion that “the magnitude of association between red and processed meat consumption and all-cause mortality and adverse cardiometabolic outcomes is very small, and the evidence is of low certainty” (20). This conclusion is contrary to the recommendations of almost all dietary guidelines and as would be expected this resulted in a critique challenging this conclusion (21). There are numerous other instances where there are conflicting results and interpretations in the literature linking diet with CVD making it difficult to sort out fact from fiction.
The information pertaining to the effect of dietary manipulations on lipid and lipoprotein levels are frequently based on randomized controlled trials rather than observational studies and therefore tend to be more consistent. However, even in these studies the results are sometimes conflicting. There are many factors that could account for this variability including the heterogeneity in study settings, type of individuals studied, study designs, differences in baseline diets, adherence to the study diet, differences in types of diet or dietary composition, methods and accuracy of the methods used to measure lipid and lipoprotein levels, and many other factors.
Additionally, the clinician should recognize that the lipid response of an individual patient to dietary manipulations can vary greatly, is very modest on average (in the range of 10% reductions, typically), and in most cases will not prevent the need for lipid lowering medications. The importance of genetic differences on these responses is often under recognized by patients and providers. For example, individuals with an apo E4 allele have a more robust decrease in LDL-C in response to a decrease in dietary fat and cholesterol than subjects carrying the apo E3 or apo E2 alleles (22). Polymorphisms in other genes have also been shown to modulate the lipid and lipoprotein response to dietary manipulations (22,23). Clinical conditions can also affect the response to diet. For example, the expected lipid and lipoprotein response to a low cholesterol, low saturated fatty acids (SFA) diet is blunted in obese individuals (24). Therefore, the effect of a specific diet can vary from individual to individual and the clinician will have to monitor a patient’s response.
It should be recognized that when one increases or decreases a particular macronutrient in the diet (lipids, carbohydrates (CHO), or protein) there needs to be a reciprocal change in another macronutrient to maintain caloric balance. It can therefore be difficult to know whether the increase in a particular nutrient or a decrease in another nutrient is accounting for the observed effect (for example decreasing SFA and increasing CHO). Where possible I will try to specify which nutrient was decreased and which was increased in the studies described.
Finally, it is important to look at the effect of diet on lipids independent of weight loss. Weight loss per se can affect lipid levels resulting in a decrease in triglycerides and LDL-C and an increase in HDL-C levels (25). For a detailed discussion of the effect of weight loss on lipid levels see the chapter on obesity and dyslipidemia (25).
In this chapter we will first discuss the effect of various macronutrients, then specific foods, and finally specific diets on lipids and lipoprotein levels.
DIETARY SATURATED FATTY ACIDS
Major sources of saturated fatty acids (SFA) in the diet are milk, butter, cheese, other dairy products, beef, lamb, pork, poultry particularly the skin, palm oil, palm kernel oil, and coconut oil (tables 1 and 3).
Table 1. Fatty Acid Composition of Foods High in Saturated Fat |
||||
---|---|---|---|---|
|
Total Fat grams/100 grams |
SFA grams/100 grams |
MUFA grams/100 grams |
PUFA grams/100 grams |
Hamburger |
15.0 |
5.89 |
6.66 |
0.49 |
Pork loin |
13.3 |
5.23 |
6.19 |
1.20 |
Chicken |
12.6 |
3.50 |
4.93 |
2.74 |
Lamb |
15.1 |
6.90 |
7.00 |
1.20 |
Whole milk* |
3.9 |
2.5 |
1.0 |
0.1 |
Gouda cheese** |
30.6 |
20.3 |
7.4 |
0.9 |
Butter*** |
82.2 |
52.1 |
20.9 |
2.8 |
*TFA = 0.1g/100g; **TFA = 1.1g/100g; TFA = 2.9g/100g.
TFA= trans fatty acids, MUFA= monounsaturated fatty acids, PUFA= polyunsaturated fatty acids.
Effect of Dietary Saturated Fatty Acids on Cardiovascular Disease
OBSERVATIONAL STUDIES
Dietary guidelines uniformly recommend reducing the intake of SFA. There are a large number of observational trials that have shown an association between dietary SFA intake and CVD (26-31). However, there are meta-analyses that have not found an association between dietary SFA intake and CVD (32-36). A possible explanation for this discordance is whether the SFA in the diet is replaced by polyunsaturated fatty acids (PUFA) vs. replaced by CHO. When SFA is replaced by PUFA there is a reduction in CVD whereas replacement with CHO has no benefit on CVD (27-29,37-39). However, replacement of SFA with high quality CHO may be beneficial (27,37,38). Additionally, in one study SFA from meat was associated with an increased risk of CVD while SFA from dairy products was associated with a decrease in CVD (40). Thus, the source of SFA may be important.
As noted above, observational studies can demonstrate an association but are not able to definitively demonstrate a causal relationship. It is therefore essential to review the results of randomized controlled trials on the effect of decreasing dietary SFA on preventing cardiovascular events.
RANDOMIZED CONTROLLED OUTCOME TRIALS
This section will review the major randomized trials analyzing the effect of decreasing SFA intake on preventing CVD. Studies with very few participants, few cardiovascular events, or very short-term studies will not be included. It is important to note that many of these studies were carried out in the 1950’s and 1960’s when the diagnosis and treatment of CVD was very primitive compared to current standards. Also, typical diets were much different (higher in SFA) and mean plasma cholesterol levels were higher. Lastly, the methodology of these studies was not up to the current standards by which randomized controlled trials are performed (small number of patients, often not blinded, inadequate statistical power, non-specific endpoints, etc.). Thus, the accuracy of these trials and the relevancy of these older studies to current times is uncertain.
In a study from England initiated in 1957, 252 men under the age of sixty-five who recently
had a myocardial infarction were assigned to a low-fat diet or usual diet (41). The low-fat diet was limited to 40 grams per day of fat with decreases in butter and meat. The intake of fat during the trial was approximately 100-120 grams per day in the usual diet group and slightly greater than 40 grams per day in the low-fat diet group. At the time of the study the typical diet was high in SFA so a decrease in total fat would have resulted in a significant decrease in SFA. During the trial serum cholesterol levels were in the 240mg/dL range in the usual diet group and 220mg/dL in the low-fat diet group. There were no differences between the two groups in cardiovascular events during the 5 years of the trial. To see a reduction in cardiovascular events with the modest reduction in serum cholesterol levels this study would have required a much larger number of participants.
The Oslo Diet-Heart Study randomized men under 65 years of age with a history of a myocardial infarction to a diet low in SFA and cholesterol, and high in PUFA (n=206) or their usual diet (n=206) (42). Cholesterol levels were approximately 295mg/dL and decreased to approximately 240mg/dL in the patients on the low SFA diet with minimal changes in the control group. After 5 years major cardiovascular events and cardiovascular mortality were reduced in the group on the low SFA diet (Events- 61 low SFA group vs. 81 control group; Mortality- 38 low SFA group vs. 52 control group).
The Medical Research Council soya-bean trial randomized men under 60 years of age with a recent myocardial infarction to continue their usual diet (n=194) or a diet low in SFA and containing 85 grams of soya-bean oil daily (PUFA) (n=199) (43). The low SFA diet lowered cholesterol from 272 to 213mg/dL (22% decrease) while in the controls, cholesterol decreased from 273 to 259mg/dL (6% decrease). The primary outcome was first relapse (myocardial infarction, angina, sudden death). After 4 years, 62 of 199 in the soybean oil group had a recurrent coronary event compared with 74 of 194 in the usual diet group; the difference, −18% (95% CI, −38 to 7), was not statistically significant but given the small number of participants was suggestive of benefit.
The Los Angeles Veterans Administration Center study randomized 422 men to the conventional control diet and 424 to the experimental diet low in SFA and cholesterol and enriched in PUFA (44,45). 30% of the men had CVD. The baseline plasma cholesterol was 233mg/dL and on treatment there was a 13% decrease in the experimental diet compared to controls. Over 8 years the primary endpoint of myocardial infarction and sudden death from ischemia were reduced in the experimental diet group (control 67 vs experimental diet 45). The difference in the primary end point of the study-sudden death or myocardial infarction was not statistically significant but when these data were pooled with those for cerebral infarction and other secondary end points, the totals were 96 in the control group and 66 in the experimental group; P=0.01. Fatal atherosclerotic events occurred in 70 patients in the control group and 48 in the experimental group (P<0.05). For all primary and secondary end points the incidence rates were 47.7% and 31.3% for the control and experimental groups respectively (P= 0.02).
The Finnish Mental Hospital Study was carried out in two mental hospitals. One hospital was switched to a diet low in SFA and cholesterol and relatively high in PUFA, while the other hospital continued the usual hospital diet (46-48). After 6 years the type of diet was reversed in each hospital. The individuals in this study were hospitalized men between 34 to 64 years of age and women age 44 to 64 years. During the study individuals were removed from the study and others added to the study cohort. The serum cholesterol level on the usual diet was 268mg/dL while on the low SFA diet the serum cholesterol level was 226mg/dL. The incidence of CVD was consistently much lower during the low SFA diet periods than during the normal-diet periods but detailed comparisons are difficult due to the lack of randomization of individuals and the adding and removal of individuals during the study leading to only 36% of the men and 20.6% of the women completing both periods of the study. Nevertheless, this study provides evidence of the benefit of a diet low in SFA and cholesterol and enriched in PUFA.
The Sydney Diet Heart Study was a randomized controlled trial conducted from 1966 to 1973 that evaluated the effects of increasing linoleic acid from safflower oil (PUFA ~ 15% of calories) in place of SFA (<10% of calories) in men aged 30-59 years with a history of coronary artery disease (49). Participants were randomized to the dietary intervention group (n=221) or a control group with no specific dietary instruction (n=237). Baseline cholesterol levels were ~280mg/dL and decreased to 267mg/dL in the control group and 244mg/dL in the diet intervention group. Compared with the control group, the intervention group had an increased risk of all-cause mortality (17.6% v 11.8%; P=0.051), cardiovascular mortality (17.2% v 11.0%; P=0.037), and mortality from coronary heart disease (16.3% v 10.1%; P=0.036) over the 5 years of the trial. The reason for the increase in mortality is not clear but the safflower oil margarine substitute for animal fats may have contained trans fatty acids, which could have increased CVD.
The DART trial was a multicenter trial in men less than 70 years of age with a diagnosis of an acute myocardial infarction (50). There were several different dietary approaches used in this trial but the one of interest reduced fat intake to 30% of total energy and increased the PUFA/SFA ratio to 1.0 (n=1018) vs. no advice (n=1015). The fat advice group reduced SFA from 15% to 11% of total calories, increased PUFA from 7% to 9%, and increased carbohydrate intake from 44% to 46%. Cholesterol levels were reduced by 3.6% (baseline 252mg/dL) in the diet advice group. During the 2-year trial the number of cardiovascular events were similar in the diet group vs. no advice group.
The Minnesota Coronary Survey was a 4.5-year, randomized trial that was conducted in six Minnesota state mental hospitals and one nursing home and included 4,393 men and 4,664 women (51). The trial compared the effects of the usual diet (18% SFA, 5% PUFA, 16% monounsaturated fatty acid (MUFA), 446 mg dietary cholesterol per day) versus a low SFA and cholesterol treatment diet (9% SFA, 15% PUFA, 14% MUFA, 166 mg dietary cholesterol per day). The mean duration of time on the diets was 384 days, with 1,568 subjects consuming the diet for over 2 years. The baseline serum cholesterol level was 207 mg/dL, falling to 175 mg/dL in the treatment group and 203 mg/dL in the control group. No differences between the treatment and control groups were observed for cardiovascular events, cardiovascular deaths, or total mortality, perhaps due to the relatively short duration of this study.
The Women’s Health Initiative trial randomized 19,541 postmenopausal women 50-79 years of age to the diet intervention group and 29,294 women to usual dietary advice (52). The goal in the diet intervention group was to reduce total fat intake to 20% of calories and increase intake of vegetables/fruits to 5 servings/day and grains to at least 6 servings/day. Fat intake decreased by 8.2% of energy intake in the intervention vs the comparison group, with small decreases in SFA (2.9%), MUFA (3.3%), and PUFA (1.5%) with increased consumption of vegetables, fruits, and grains. LDL-C levels were reduced by 3.55 mg/dL in the intervention group while levels of HDL-C and TGs were not significantly different in the intervention vs comparison groups. The dietary intervention did not significantly decrease CVD. In fact, in the women with pre-existing CVD there was an increase in cardiovascular events with diet therapy.
Summary of Dietary Randomized Controlled Trials
In reviewing these randomized controlled trials, it appears that the dietary studies that produce a long-term decrease in plasma cholesterol levels resulted in a reduction in cardiovascular events (Oslo Diet-Heart Study, soya-bean trial, Los Angeles Veterans Administration Center, Finnish Mental Hospital Study) while the dietary studies that did not produce a long-term decrease in plasma cholesterol levels failed to demonstrate a reduction in CVD. The baseline plasma cholesterol levels in the positive studies tended to be high and allowed for a robust cholesterol lowering with dietary manipulation. Additionally, as will presented in the next section the greater the reduction in SFA in the diet the greater the decrease in TC and LDL-C levels and many of the positive studies were carried out in an era when the content of SFA in the diet was high. Additionally, studies in non-human primates have also demonstrated that reducing SFA intake reduces atherosclerosis (53,54).
These results correspond very nicely with the large number of trials demonstrating that using a variety of different pharmacologic agents that lower plasma cholesterol levels results in a decrease in cardiovascular events (55). In an analysis comparing cholesterol lowering with diet vs. drug therapy it was observed that a similar decrease in cardiovascular events occurred adjusting for the magnitude of cholesterol lowering (56). Thus, it would appear that diets that decrease dietary SFA and thereby lead to a significant decrease in plasma cholesterol levels for an extended period of time have benefits on CVD with the caveat that there is not an increase in other nutrients that will adversely affect other parameters thereby negating the beneficial effects of decreasing SFA. For example, an increase in dietary simple sugars for SFA could lead to an increase in TG levels with negative effects.
REVERSAL OF ATHEROSCLEROSIS TRIALS
Two studies have examined the effect of decreasing dietary SFA on atherosclerotic lesions.
The St Thomas’ Atherosclerosis Regression Study (STARS) determined the effect of decreasing dietary saturated fat in the diet (n=26) vs. usual diet (n=24) in men less than 66 years of age with a plasma cholesterol greater than 234mg/dL referred for coronary angiography to investigate angina pectoris or other findings suggestive of coronary heart disease (57). In the diet group total fat intake was reduced to 27% of dietary energy, saturated fatty acid content to 8-10% of dietary energy, and dietary cholesterol to 100 mg/1000 kcal; omega-6 and omega-3 polyunsaturated fatty acids were increased to 8% of dietary energy, and plant-derived soluble fiber intake was increased to the equivalent of 3-6 g polygalacturonate/1000 kcal. During the trial LDL-C levels were 163mg/dL in the diet intervention group vs.182mg/dL in the usual diet group. Additionally, TGs decreased in the diet intervention group (206mg/dL to 165mg/dl) with no change in TG levels in the usual diet group. After approximately 3 years coronary angiography revealed that the percentage of patients who showed progression of coronary narrowing was significantly reduced by the dietary intervention (usual diet 46% vs, dietary intervention 15%), whereas the percentage who showed an increase in luminal diameter rose significantly (usual diet 4% vs. dietary intervention 38%). While the number of cardiovascular events was small, they were significantly reduced in the dietary intervention group (usual diet 36% vs dietary intervention 11%; p< 0.05). Finally, the improvement in angiographic appearance correlated with LDL-C levels.
The Lifestyle Heart Trial was a one year randomized, controlled trial to determine whether lifestyle changes affect coronary atherosclerosis in patients with angiographically documented coronary artery disease (58). Patients were assigned to the lifestyle group (low-fat vegetarian diet, stopping smoking, stress management training, and moderate exercise) (n= 22) or a usual-care control group (n=19). The lifestyle diet contained approximately 10% of calories as fat PUFA/SFA ratio greater than 1), 15-20% protein, and 70-75% predominantly complex carbohydrates. Cholesterol intake was limited to 5 mg/day or less. In the lifestyle group LDL-C decreased from 153mg/dL to 96mg/dl (37% decrease) whereas in the usual care group LDL-C decreased from 168mg/dL to 159mg/dL. Patients in the lifestyle group reported a 91% decrease in the frequency of angina, a 42% decrease in the duration of angina, and a 28% decrease in the severity of angina. In contrast, patients in the usual care group reported a 165% increase in the frequency of angina, a 95% increase in the duration of angina, and a 39% increase in the severity of angina. In the lifestyle group regression of coronary atherosclerosis occurred in 18 of the 22 patients (82%) whereas in the usual care group progression of coronary atherosclerosis occurred in 10 of 19 patients (53%).
These two regression trials provide strong support for the results observed in the randomized cardiovascular outcome studies described above i.e., that lowering LDL-C levels by decreasing dietary SFA can reduce atherosclerosis and cardiovascular events.
Effect of Dietary Saturated Fatty Acids on Lipid Levels
It should be recognized that when one increases or decreases a particular macronutrient in the diet there needs to be a reciprocal change in another macronutrient to maintain caloric balance.
The effect of substituting PUFA, MUFA, or carbohydrates (CHO) for SFA is shown in table 1. Note that this table shows the effect of replacing 5% of energy from SFA for the indicated dietary component. Thus, going from a diet where 15% of the calories is from SFA to a diet where 10% of the calories is from SFA is estimated to lower LDL-C levels from 6 to 9mg/dL depending on which dietary component replaces the SFA. To keep this decrease in LDL-C in perspective it is estimated that a 40mg/dL decrease in LDL-C induced by statin therapy will result in an approximate 20% decrease in cardiovascular events over a 5 year period of time but the lifetime benefits of a 10 mg/dL decrease in LDL-C due to genetic variants will result in a 16–18% decrease in cardiovascular events (59). The effect on TGs is dependent on the dietary component replacing SFA with CHO resulting in a large increase in TG levels. One should note that there is also a decrease in HDL-C with replacement of SFA (table 2).
Table 2. Effect of Decreasing Dietary Saturated Fatty Acids on Lipid Levels |
|||
---|---|---|---|
Dietary Component |
LDL-C (mg/dL) |
TGs (mg/dL) |
HDL-C (mg/dL) |
PUFA |
−9.0 |
-2.0 |
-1.0 |
MUFA |
-6.5 |
+1.0 |
-6.0 |
CHO |
-6.0 |
+9.5 |
-2.0 |
PUFA- polyunsaturated fatty acids; MUFA- monounsaturated fatty acids; CHO- carbohydrates.
Effects on lipoprotein lipids of replacing 5% of energy from SFA with the 5% of energy from the specified dietary component. Table adapted from references (26,60).
SFA in the diet predominantly increases LDL-C levels, predominantly larger, cholesterol-enriched LDL, with modest increases in HDL-C (60,61). As expected, Apo B and apo AI levels also increase (60). These effects are observed in both men and women (60). The effect of a decrease or increase in SFA intake on lipids and lipoproteins is linear with a consistent effect on serum lipids and lipoproteins across a wide range of SFA intakes (60). Of note the effects of decreasing SFA intake was observed even when the SFA intake was already less than 10% of the daily energy intake. Most studies have suggested that replacement of SFA with carbohydrate or unsaturated fat modestly increases Lp(a) but the results have varied from study to study with replacement of SFA with unsaturated fat from particular food sources such as nuts showing no increase in Lp(a) (62).
Individual SFA have diverse biological and cholesterol-raising effects with chain length of SFA playing an important role in determining the effect on lipid and lipoprotein levels. The most commonly consumed SFA are palmitic acid (16:0; major source: vegetable oil, dairy, and meat), stearic acid (18:0; meat, dairy, and chocolate), myristic acid (14:0; dairy and tropical oil, particularly coconut oil) and lauric acid (12:0; dairy and tropical oil). A meta-analysis of 60 controlled trials by Mensink et al. reported an increase in LDL-C and HDL-C concentrations by isocaloric replacement of carbohydrates with palmitic, myristic, and lauric acids (63). As expected, apolipoprotein B and A-I also increase (60,64). Myristic and palmitic acids increased LDL-C and HDL-C levels to a similar extent, whereas lauric acid had the largest LDL-C- and HDL-C-raising effect (63,65). Stearic acid did not increase LDL-C levels (63,65).The lack of an association between stearic acid and changes in LDL-C levels has been linked to a slower and/or less efficient absorption as well as desaturation of stearic acid to oleic acid (66). Compared with carbohydrates, an increased intake of lauric, myristic, palmitic or stearic acid lowered TG levels (63,65). For a specific individual many factors including lifestyle factors such as overall dietary composition and physical activity, clinical conditions such as obesity, insulin resistance and hypertriglyceridemia, as well as genetic factors may modify these responses.
MECHANISM FOR THE INCREASE IN LDL-C
Dietary SFA have been shown to decrease hepatic LDL receptor activity, protein, and mRNA levels and this results in a decrease in the clearance of circulating LDL leading to increased LDL-C levels (67,68). Additionally, the decrease in LDL receptors could result in an increase in the conversion of intermediate density lipoproteins to LDL rather than clearance by the liver (i.e., LDL production is enhanced).
SFA have been shown to decrease the formation of cholesterol esters, a reaction catalyzed by the enzyme acyl CoA:cholesterol acyltransferase (ACAT) (68). Free cholesterol in the endoplasmic reticulum is the primary regulator of the activation of sterol receptor binding protein (SREBP), which translocates to the nucleus and enhances the transcription of the LDL receptor (69). Elevated levels of cholesterol in the endoplasmic reticulum prevents the activation of SREBP (69). When free cholesterol is esterified into cholesterol esters it no longer prevents the activation of SREBP and the up-regulation LDL receptor expression. Thus, SFA by decreasing the formation of cholesterol esters and increasing free cholesterol may lead to the down-regulation of LDL receptor expression (68).
DIETARY MONOUNSATURATED AND POLYUNSATURATED FATTY ACIDS
Olive oil, canola oil, peanut oil, safflower oil, sesame oil, avocados, peanut butter, and many nuts and seeds are major sources of MUFA (table 3). Soybean oil, corn oil, sunflower oil, some nuts and seeds such as walnuts and sunflower seeds, tofu, and soybeans are major sources of PUFA (table 3). Omega-3-fatty acids, eicosapentaenoic acid (EPA, 20:5) and docosahexaenoic acid (DHA, 22:6), are mostly found in fish and other seafood, while another omega-3 fatty acid, alpha-linolenic acid (ALA, 18:3) is found mostly in nuts and seeds such as walnuts, flaxseed, and some vegetable oils such as soybean and canola oils. The body is capable of converting ALA into EPA and DHA but the conversion rates are low.
Table 3. Fat Composition of Oils, Lard, Butter, and Margarine |
|||
---|---|---|---|
Type of Oil |
SFA (%) |
MUFA (%) |
PUFA (%) |
Corn oil |
13.6 |
28.97 |
57.43 |
Safflower oil (linoleic) |
6.51 |
15.1 |
78.4 |
Canola oil |
7.46 |
64.1 |
28.49 |
Almond oil |
8.59 |
73.19 |
18.22 |
Olive oil |
14.19 |
74.99 |
10.82 |
Soybean oil |
16.27 |
23.69 |
60.0 |
Sesame oil |
14.85 |
41.53 |
43.62 |
Sunflower oil (linoleic) |
10.79 |
20.42 |
68.8 |
Avocado oil |
12.1 |
73.8 |
14.11 |
Peanut oil |
17.77 |
48.58 |
33.65 |
Palm oil |
51.57 |
38.7 |
9.73 |
Coconut oil |
91.92 |
6.16 |
1.91 |
Lard |
41.1 |
47.23 |
11.73 |
Butter |
68.1 |
27.87 |
4.0 |
Margarine (soft) |
20 |
47 |
33 |
Margarine (hard) |
80 |
14 |
6 |
U.S. Department of Agriculture
Effect of Dietary Monounsaturated and Polyunsaturated Fatty Acids on Cardiovascular Disease
MONOUNSATURATED FATTY ACIDS
Many meta-analyses, but not all, have failed to demonstrate that MUFA intake reduces cardiovascular events (29,33,35,70). However, one meta-analysis and the Nurses’ Health Study and Health Professionals Follow-Up Study, two very large observational studies, found that MUFA when delivered from plant sources was protective but MUFA from other sources was not protective from developing cardiovascular events (71,72).
The PREDIMED a randomized controlled outcome trial employing a Mediterranean diet (increased MUFA) reduced the incidence of major CVD (73-75). In this multicenter trial, carried out in Spain, over 7,000 individuals at high risk for developing CVD were randomized to three diets (primary prevention trial). A Mediterranean diet supplemented with extra-virgin olive oil, a Mediterranean diet supplemented with mixed nuts, or a control diet. In the patients assigned to the Mediterranean diets there was 29% decrease in the primary composite end point (myocardial infarction, stroke, and death from CVD), which was primarily due to a decrease in strokes. The Mediterranean diet resulted in a small but significant increase in HDL-C levels and a small decrease in both LDL-C and TG levels (76). The changes in lipids were unlikely to account for the beneficial effects of the Mediterranean diet on CVD.
The Lyon Diet Heart Study randomized 584 patients who had a myocardial infarction within 6 months to a Mediterranean type diet vs usual diet (77,78). The oils recommended for salads and food preparation were rapeseed and olive oils exclusively. Additionally, they were also supplied with a rapeseed (canola) oil-based margarine. There was a marked reduction in events in the group of patients randomized to the Mediterranean diet (cardiac death and nonfatal myocardial infarction rate was 4.07 per 100 patient years in the control diet vs.1.24 in the Mediterranean diet; p<0.0001). Lipid levels were similar in both groups in this trial (77).
The CORDIOPREV study was a single center randomized trial that compared a Mediterranean diet to a low-fat diet in 1,002 patients with cardiovascular disease (79). The Mediterranean diet contained a minimum of 35% of the calories as fat (22% monounsaturated fatty acids, 6% polyunsaturated fatty acids, and <10% saturated fat), 15% proteins, and a maximum of 50% carbohydrates while the low-fat diet contained less than 30% of total fat (<10% saturated fat, 12–14% monounsaturated fatty acids, and 6–8% polyunsaturated fatty acids), 15% protein, and a minimum of 55% carbohydrates. The risk of an ASCVD event was reduced by approximately 25-30% in the Mediterranean diet group. Whether these diets differed in their effects on fasting lipid levels has not been reported.
The results of these three randomized trials indicate that a Mediterranean diet enriched in plant MUFA reduce the risk of CVD. It is likely that the beneficial effects of the Mediterranean diet on CVD is mediated by multiple mechanisms with alterations in lipid levels making only a minor contribution. It should be noted that in addition to an increase in MUFA the diet also includes low to moderate red wine consumption, high consumption of whole grains and cereals, low consumption of meat and meat products, increased consumption of fish, and moderate consumption of milk and dairy products. As in many dietary studies it is difficult to change a single variable and therefore the interpretation of which factor or factors account for the benefits is difficult to untangle.
POLYUNSATURATED FATTY ACIDS
Recent meta-analyses of the effect of PUFA on cardiovascular events in observational studies have demonstrated either no effect or a modestly lower risk of CVD and mortality (80-84). Randomized trials are described in the section on saturated fats and CVD and describe the results of replacing SFA with PUFA. It appears that dietary PUFA has a neutral effect on CVD except in the circumstances where it replaces SFA and results in a sustained decrease in plasma cholesterol levels leading to a decrease in cardiovascular events.
OMEGA-3-FATTY ACIDS
As discussed in detail in the chapter entitled “Triglyceride Lowering Drugs” numerous randomized controlled trials of the effect of low dose omega-3-fatty acids (approximately ≤1 gram/day) on CVD have been published and the bulk of the evidence indicates no benefit (85). The effect of pharmacologic doses of omega-3-fatty acids (≥1.8 grams/day) on cardiovascular outcomes is discussed in the chapter entitled “Triglyceride Lowering Drugs” (85).
Effect of Dietary Monounsaturated and Polyunsaturated Fatty Acids on Lipid Levels
Table 4 shows the effect of substituting PUFA or MUFA for carbohydrates on LDL-C, HDL-C, and TG levels. Both PUFA and MUFA decrease LDL-C and TGs but PUFA induces a greater decrease (60). Both PUFA and MUFA increase HDL-C levels (60).
Table 4. Effect of Decreasing Dietary Carbohydrate on Lipid Levels |
|||
---|---|---|---|
Dietary Component |
LDL-C (mg/dL) |
TGs (mg/dL) |
HDL-C (mg/dL) |
PUFA |
-4.3 |
-9.2 |
1.2 |
MUFA |
-1.8 |
-6.6 |
1.6 |
PUFA- polyunsaturated fatty acids; MUFA- monounsaturated fatty acids;
Effects on lipoprotein lipids of replacing 5% of energy from carbohydrates with the 5% of energy from the specified dietary component. Table adapted from reference (60).
In a meta-analysis of 14 studies no significant differences in TC, LDL-C, or HDL-C levels were observed when diets high in MUFA or PUFA were compared directly (86). TG levels were modestly but consistently lower on the diets high in PUFA (P = .05) (86).
While high dose omega-3-fatty acids (3-4 grams/day) lower TG levels, lower doses (≤1 gram/day) have minimal effects on lipid levels (85).
MECHANISM FOR THE DECREASE IN LDL-C
Unsaturated fatty acids increase hepatic LDL receptor activity, protein, and mRNA abundance, which will increase the clearance of LDL from the circulation (67,68). Unsaturated fatty acids are a preferred substrate for ACAT and thereby result in an increase in cholesterol ester formation and a decrease in free cholesterol in the liver (68). A decrease in hepatic free cholesterol will result in the up-regulation of LDL receptor expression leading to a decrease in LDL-C levels. PUFA also increase membrane fluidity leading to an increase in the ability of LDL receptors to bind LDL (67). Additionally, the increase in LDL receptors could result in a decrease in the conversion of intermediate density lipoproteins (IDL) to LDL due to increased uptake of IDL by the liver (i.e., LDL production is decreased).
DIETARY TRANS FATTY ACIDS
The two major sources of dietary trans fatty acids (TFA) are those that occur naturally in meat and dairy products as a result of anaerobic bacterial fermentation in ruminant animals and those formed during the partial hydrogenation of vegetable fat (the fatty acids in vegetable oils have cis double bonds) (87). Partial hydrogenation and the formation of TFA converts the liquid vegetable oil into a solid form at room temperature allowing for ease of use in food products and increased shelf life (87,88). TFA acids were widely used in baked products, packaged snack foods, margarines, and crackers (88). With the recognition of the adverse effects of TFA the use of partial hydrogenated oils in food products has markedly diminished World-wide and in the US is no longer allowed.
Effect of Trans Fatty Acids on Cardiovascular Disease
A meta-analysis by de Souza and colleagues of 5 studies with 70,864 participants found that the relative risk of coronary heart disease mortality disease was increased with dietary TFA (1.28; p=0.003) (34). Similarly, the relative risk of coronary heart disease was also increased (1.21; p<0,001) (34). Another meta-analysis by Chowdhury and colleagues of 5 studies with 155,270 participants found that the relative risk of coronary events was increased with higher intake of TFA (RR 1.16; CI 1.06-1.27) (33). It has been estimated that a 2 percent increase in energy intake from TFA was associated with a 23 percent increase in the incidence of coronary heart disease (88). Thus, observational studies have consistently demonstrated that an increase in dietary TFA increase the risk of CVD. Clearly it would not be ethical to carry out randomized trials of the effect of TFA acids on CVD.
Effect of Trans Fatty Acids on Lipid Levels
The effect of replacing SFA, MUFA or PUFA with TFA acids is shown in table 5. TFA increase LDL-C levels and decrease HDL-C levels. Of note TFA increase LDL-C even when substituting for SFA. There appears to be a nearly linear relationship between TFA intake and LDL-C concentration, but this relationship does not seem to exist between TFA intake and HDL-C (89). HDL-C seems to be lowered significantly by TFA only when intake is >2% to 4% of the total energy intake (89). TFA also increases TG and Lp(a) levels (88). Additionally, dietary TFA increases small dense LDL and the increase correlates with the quantity of TFA in the diet (90).
Table 5. Effect on Lipids of Replacing Various Fatty Acids with Trans Fatty Acids |
||
---|---|---|
Dietary Component |
LDL-C (mg/dL) |
HDL-C (mg/dL) |
SFA |
2.0 |
-2.0 |
PUFA |
11.5 |
-1.3 |
MUFA |
9.5 |
-1.5 |
SFA- saturated fatty acids; PUFA- polyunsaturated fatty acids; MUFA- monounsaturated fatty acids. All results are statistically significant (P<0.05) except the increase in LDL-C with SFA replacement. Effects on lipoprotein lipids of replacing 5% of energy from various fatty acids with 5% of the energy from TFA. Table adapted from reference (88).
Replacing carbohydrates with TFA results in an increase in LDL-C and apo B and no change in HDL-C, apo AI, or TG levels (63).
RUMINANT TRANS FATTY ACID
A key question now that TFA derived from partial hydrogenation of vegetable fat in the diet have been markedly reduced is whether ruminant derived TFA which are present in milk, butter, cheese, and beef have harmful effects similar to industrial created TFA. It is important to note that ruminant derived TFA have a different composition with ruminant TFA being enriched in vaccenic acid, which is the predominant TFA, and conjugated linoleic acid (89,91). Also the quantities of ruminant TFA ingested is much lower than the quantities of industrial TFA ingested (89). In an analysis of a large number of studies of the effect of ruminant and industrial TFA on lipid levels it was observed that the effect of ruminant TFA on LDL-C and HDL-C was similar but slightly less than that of industrial TFA (the difference was not significant) (91). Whether the low quantities of ruminant TFA in the diet will influence the risk of CVD is unknown (89) but a meta-analysis of 4 observational trials did not find a link between ruminant-TFA intake (increments ranging from 0.5 to 1.9 g/day) and the risk of CHD (RR=0.92; CI 0.76-1.11; P=0.36) (92). Another meta-analysis also did not find a link between ruminant TFA and CVD (34).
MECHANISM FOR THE LIPID EFFECTS OF TRANS FATTY ACIDS
The mechanism for the increase in LDL-C levels by dietary TFA is thought to be due to decreased LDL-Apo B catabolism without a change in LDL-Apo B production (87,93). The decrease in HDL-C induced by TFA has been attributed to an increase in HDL Apo A-I catabolism without a significant change in HDL apoA-1 production rate (87,93). Additionally, TFA increases CETP activity which could increase the transfer of cholesterol esters from HDL to LDL thereby contributing to the decreased HDL-C levels and increased LDL-C levels (94).
DIETARY CHOLESTEROL
The primary food sources of dietary cholesterol are egg yolks, shrimp, beef, pork, poultry, cheese, and butter with the top five food sources being eggs and mixed egg dishes, chicken, beef, burgers, and cheese (table 6) (95). In the US the typical cholesterol intake varies from 50 to 400mg per day with a mean of 293 mg/day (348 mg/day for men and 242 mg/day for women) (96).
Table 6. Cholesterol Content of Food |
|
---|---|
Food |
mg per 100 grams |
Egg |
373 |
Butter |
215 |
Shrimp |
125 |
Cheese |
108 |
Beef |
90 |
Chicken |
88 |
Pork |
80 |
Ice Cream |
47 |
Effect of Dietary Cholesterol on Cardiovascular Disease
In reviews of prospective observational studies an association between dietary cholesterol and CVD has not been clearly demonstrated with some studies reporting an association and others no association (97,98). Most of these studies did not adjust for the amount and types of fatty acids consumed, which could influence the results as foods containing large amounts of cholesterol are also rich in SFA. Dietary cholesterol was not associated with cardiovascular risk among >80,000 nurses and 43,000 male health care professionals after adjusting for energy intake, PUFA, trans fatty acid, and SFA intake (99,100).
Most foods that contain cholesterol also contain significant amounts of SFA. An exception are eggs which contain significant amounts of cholesterol and only small amounts of SFA (95). It is therefore of interest to examine the effect of egg consumption on CVD. In an analysis of 7 cohort studies no association between egg intake and coronary heart disease was observed and egg intake may be associated with a reduced risk of stroke (101). A recent meta-analysis of 23 prospective studies with 1,415,839 individuals and a median follow-up of 12.28 years also found that increased consumption of eggs was not associated with increased risk of CVD (102). Other meta-analyses and reviews have also not demonstrated a consistent link between eggs and CVD (98,103-105). However, a recent very large meta-analysis with 3,601,401 participants with 255,479 events showed that the consumption of 1 additional 50-g egg daily was associated with a very small increase in CVD risk (pooled relative risk, 1.04; 95% CI 1.00-1.08) (106). Thus, eggs have either no effect or a very small effect on CVD that can be seen only in very large studies.
There appears to be no randomized studies of the effect of decreasing cholesterol intake on CVD. Do recognize that the studies of decreasing dietary SFA intake described earlier also result in a decrease in cholesterol intake. Thus, at this time there is very limited data linking dietary cholesterol intake with an increased risk of CVD.
Effect of Dietary Cholesterol on Lipid Levels
In a meta-analysis of fifty-five studies with 2,652 subjects the predicted change in LDL-C levels for an increase of 100 mg dietary cholesterol per day adjusted for dietary fatty acids ranged from 1.90mg/dL to 4.58 mg/dL depending upon the model employed (107). An increase of 200mg dietary cholesterol per day increased LDL-C levels from 3.80mg/dL to 6.96mg/dL. It should be noted that the effect of dietary cholesterol levels is greater the higher the LDL-C level (107). For a baseline LDL-C level of 100, 125, 150, and 175 mg/dL the predicted increase in LDL-C for a change in dietary cholesterol of 100mg is 2.7, 3.6, 4.6, and 5.5 mg/dL respectively (107). While the absolute increase is greater if the LDL-C level is higher the percentage increase is similar. Moreover, cholesterol feeding does not alter number of LDL particles – instead it increases the cholesterol content of the LDL particles leading to the formation of large buoyant LDL (108).
The effect of dietary cholesterol on HDL-C levels differs in males and females. In men an increase of 100mg of dietary cholesterol results in a 0.30 to 1.44mg/dL decrease in HDL-C levels while in women this results in a 0.50 to 1.61 increase in HDL-C levels (107). Dietary cholesterol does not impact TG or VLDL cholesterol levels (97).
Approximately 15-25% of the population have an increased response to dietary cholesterol with greater increases in LDL-C levels (i.e., sensitive or hyper-responders), while the majority respond minimally (i.e., non-sensitive or hypo-responders) (109). An intake of 100 mg/day dietary cholesterol leads to a 3-4-fold difference in LDL-C concentration between hyper- and hypo-responders (an increase of 2.84 mg/dL vs. 0.76 mg/dL (110). The mechanism for the increase in cholesterol absorption in hyper-responders is unknown. On average 50% (typical range 40-60%) of dietary cholesterol is absorbed but this varies from person to person (111). A high-cholesterol diet leads to significant increases in non-HDL-C levels in insulin-sensitive individuals but not in lean or obese insulin-resistant subjects whereas HDL-C levels increased in all 3 groups (112). The above observations demonstrate the variable response of lipid and lipoprotein levels that can occur in response to dietary manipulations and emphasize how the response of an individual can be variable.
MECHANISM FOR THE INCREASE IN LDL-C
The increase in LDL-C levels by dietary cholesterol is due to a decrease in hepatic LDL receptors (111). Cholesterol absorbed by the small intestine is packaged into chylomicrons which deliver dietary cholesterol to the liver (111). This increases hepatic cholesterol levels which down-regulates the expression of LDL receptors leading to a decrease in the clearance of LDL from the circulation (111). Additionally, the decrease in LDL receptors could result in an increase in the conversion of intermediate density lipoproteins to LDL rather than clearance by the liver (i.e., LDL production is enhanced).
DIETARY CARBOHYDRATES
Carbohydrates (CHO) can be divided into high-quality CHO, for example fruits, legumes, vegetables, and whole grains, or low-quality CHO, which include refined grains (such as white bread, white rice, cereal, crackers, and bakery desserts), starches (potatoes), and added sugars (sugar-sweetened beverages, candy). The high-quality CHO are typically enriched in fiber and have a low glycemic index/glycemic load (i.e., are slowly absorbed and thus do not rapidly increase plasma glucose levels). The low-quality CHO have a high glycemic index and load and rapidly increase plasma glucose levels.
Effect of Dietary Carbohydrates on Cardiovascular Disease
OBSERVATIONAL STUDIES
When SFA is replaced by CHO there is no reduction in CVD whereas replacement of SFA with high quality CHO may be beneficial (27,37,38). A study by Jakobsen and colleagues found that replacing SFA with CHO with a low-glycemic index value is associated with a lower risk of myocardial infarction whereas replacing SFA with CHO with a high-glycemic index values is associated with a higher risk of myocardial infarction (113). Meta-analyses and reviews of the association of glycemic index with CVD have varied with some showing an association of low glycemic index with CVD and others reporting no link (114,115). Two very large studies found that a diet with a high glycemic index was associated with an increased risk of cardiovascular disease (116,117). It should be noted that in the largest study the relative risk for CVD was relatively modest (RR 1.15; 95% CI 1.11-1.19) (117). An increase in cardiovascular morbidity and mortality was associated with an increase in added sugar intake (118-121). Hazard ratios were 1.30 (95% CI- 1.09-1.55) and 2.75 (95% CI-1.40-5.42), respectively, comparing participants who consumed 10.0% to 24.9% or 25.0% or more calories from added sugar with those who consumed less than 10.0% of calories from added sugar (118). Additionally, in the Health Professionals Follow-up Study participants in the top quartile of sugar-sweetened beverage intake had a 20% higher relative risk of coronary heart disease than those in the bottom quartile (RR=1.20; 95% CI- 1.09-1.33) after adjustment for multiple risk factors (122).
RANDOMIZED CONTROLLED TRIALS
Three of the randomized trials described above in the SFA and CVD section provide information on the role of CHO on CVD. The British Medical Research Council studied 252 men after a myocardial infarction aiming to reduce total fat from 41% to 22% of calories and maintaining total fat at 41% in the control group (41). The type of fat was similar in the high- and low-fat groups, mainly saturated fat from dairy products and meat. It is likely that the decrease in fat calories was substituted by an increase in CHO calories. The type of CHO that replaced the SFA was not specified but the authors indicated that there was a marked increase in sugar intake in the low- fat diet group. There was no difference between the two groups in cardiovascular events during the 5 years of the trial. The DART study decreased SFA which were substituted with PUFA and CHO (50). During the 2-year trial cardiovascular events were similar in the decreased SFA vs. PUFA and CHO group. Finally, the Women’s Health Initiative trial randomized 19,541 postmenopausal women 50-79 years of age to the diet intervention group and 29,294 women to usual dietary advice (52). The goal in the diet intervention group was to reduce total fat intake to 20% of calories and increase intake of vegetables/fruits to 5 servings/day and grains to at least 6 servings/day (i.e., CHO}. Fat intake decreased by 8.2% of energy intake in the intervention vs the comparison group, with small decreases in SFA (2.9%), MUFA (3.3%), and PUFA (1.5%) fat with increased consumption of CHO. The dietary intervention did not significantly decrease CVD even though the CHO recommended was high quality CHO. These randomized studies do not provide support for a benefit of substituting CHO for fat in reducing CVD. Of particular note is the Women’s Health Initiative which decreased fat intake and increased high quality CHO and observed no cardiovascular benefits in contrast to the results of observational studies.
Effect of Dietary Carbohydrates on Lipids
Replacing SFA, MUFA, or PUFA with CHO results in an increase in TGs and a decrease in HDL-C levels (60,63). Replacing SFA with CHO results in a decrease in LDL-C while replacing MUFA or PUFA with CHO results in an increase in LDL-C (see tables 2 and 4) (60,63). In addition, dietary CHO increases the quantity of small dense LDL particles (123). The consumption of moderate amounts of fructose or sucrose (40-80 grams/day) in healthy young men was sufficient to increase small dense LDL levels (124). The effect of increasing dietary CHO on Lp(a) levels has been variable (62).
Conversely, decreasing CHO in the diet and adding fat results in an increase in LDL-C and HDL-C levels and a decrease in TG levels (125). In a meta-analysis of eleven randomized controlled trials with 1,369 participants comparing low fat/high CHO diet to high fat/low CHO diet it was found that the high fat/low CHO led to an increase in LDL-cholesterol (6.24mg/dL; 95 % CI 0.12- 12.9) and HDL-C (5.46mg/dL; 95% CI 3.51- 7.41) compared with subjects on the low fat/high CHO diets (126). The high fat/low CHO decreased TG levels (-22.9mg/dL; 95 % CI -13.4- -32.6 (126). Another meta-analysis of 23 randomized controlled trials also found that a high fat/low CHO diet increased LDL-C and HDL-C levels and decreased TG levels (127). These studies nicely demonstrates that a high fat diet will increase LDL-C and HDL-C levels while a high CHO diet will increase TG levels and decrease HDL-C levels.
COMPARISON OF DIFFERENT CARBOHYDRATES ON LIPIDS
A meta-analysis of twenty-eight randomized controlled trials comparing low- with high glycemic index diets (1,272 participants) reported that low glycemic index diets significantly decreased LDL-C levels by 6.2mg/dL; P < 0.0001) with no effect on HDL-C or TGs (128). The decrease in LDL-C was related to the amount of fiber and/or phytosterols in the low glycemic diet (see Fiber and Plant Sterols/Stanols section below).
High fructose corn syrup (HFCS) has become a major source of fructose intake (HFCS made for beverages contains 55% fructose and 45% glucose). Because sucrose and HFCS are major contributors to total CHO intake there has been interest in the effect of fructose, glucose, and sucrose on lipid levels. In a comparison of isocalorically substituting starch for glucose, fructose, or sucrose there were no difference in TG levels but there was a decrease in LDL-C (approximately 7.8mg/dL) (129).
A meta-analysis by Te Morenga and colleagues examined the effect of the addition of sugar on lipid levels. In studies where energy intake was isocaloric, sugar intake increased TG levels by 11.7mg/dL, LDL-C by 6.6mg/dL, and HDL-C by 0.8mg/dL (130). In a similar meta-analysis by Fattore and colleagues an isocaloric substitution of free sugars for complex CHO increased TGs by 8.3mg/dL, LDL-C by 7.1mg/dL, and HDL-C by 1.3mg/dL (131). The increase in TG and LDL-C levels were larger in the trials where greater amounts of free sugar were employed.
In a meta-analysis of adding fructose to the diet there was no significant effect on fasting TG levels at dietary fructose < 100 grams per day but at higher amounts fructose increased fasting TG levels (132). Fructose is more likely to have adverse effects on lipids when intake is high and/or when caloric excess is present. For example, in young healthy individuals, a 2-week intervention with 25% of energy requirements as HFCS or fructose sweetened beverages resulted in significant increases in fasting LDL-C, small dense LDL particles, non-HDL-C, apo B, and HDL-C and postprandial TGs (133). High quantities of glucose did not affect LDL-C, non-HDL-C, Apo B, HDL-C, or postprandial TG levels but did increase fasting TG levels (133).
Thus, the effect of CHO on lipids can vary depending upon the particular type of CHO studied (table 7). In the case of glycemic index (complex CHO) and starch vs sugar some of the difference in lipid response could be due to other dietary constituents (i.e., fiber, phytosterols).
Table 7. Summary of the Effect of Different Carbohydrates on Lipid and Lipoproteins |
|
---|---|
Comparisons |
Effect on Lipids and Lipoproteins |
Low GI vs. High GI |
High GI increases LDL-C |
Sugar vs. Starch |
Sugar increases LDL-C |
Sugar vs. Complex CHO |
Sugar increases LDL-C and TGs |
Fructose vs. Glucose |
Fructose increases LDL-C and HDL-C and postprandial TGs |
Sugar- sucrose, glucose, or fructose
MECHANISM OF THE EFFECTS OF CARBOHYDRATES ON LIPIDS
Dietary CHO promote hepatic de novo fatty acid synthesis by providing substrate for fatty acid synthesis (Figure 1). This is particularly the case when there is caloric excess. Additionally, the glucose provided by dietary CHO stimulates insulin secretion which also increases hepatic fatty acid synthesis. The increase in fatty acid synthesis in the liver enhances TG synthesis which promotes VLDL formation and secretion leading to an increase in plasma TG levels.
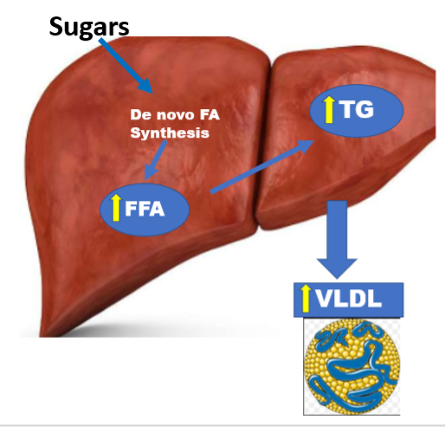
Figure 1. Carbohydrates stimulate VLDL production by stimulating de novo fatty acid synthesis.
Fructose is more potent at increasing de novo fatty acid synthesis than glucose. Small quantities of fructose in the diet are metabolized in the small intestine to glucose and organic acids and do not affect systemic metabolism while high quantities of fructose can escape intestinal metabolism and are delivered to the liver (134). In the liver fructose but not glucose activates SREBP1c and ChREBP leading to the increased expression of the genes that synthesize fatty acids stimulating hepatic lipogenesis (134,135). Additionally, fructose metabolism in the liver is not inhibited providing an unlimited supply of fructose carbons for lipogenesis. In contrast, the first steps in glucose metabolism can be inhibited and thus the utilization of glucose for lipogenesis is regulated (134). In addition, fructose inhibits fatty acid oxidation whereas glucose does not (135). These differences in the metabolism of fructose and glucose in the liver explain the increased ability of fructose to stimulate hepatic lipogenesis and the enhanced formation and secretion of VLDL. In the addition to increased VLDL production fructose does not stimulate the secretion of insulin, which plays a key role in stimulating lipoprotein lipase activity and the clearance of TG rich lipoproteins. The failure of dietary fructose to induce an increase in lipoprotein lipase activity may lead to a decrease in the clearance of TG rich lipoproteins compared to dietary glucose, which stimulates insulin secretion.
The elevation in TG rich lipoproteins in turn may have effects on other lipoproteins (25) (Figure 2). Specifically, cholesterol ester transfer protein (CETP) mediates the equimolar exchange of TGs from TG rich VLDL and chylomicrons for cholesterol from LDL and HDL (25). The increase in TG rich lipoproteins per se leads to an increase in CETP mediated exchange, increasing the TG content and decreasing the cholesterol content of both LDL and HDL particles. This CETP-mediated exchange underlies the commonly observed reciprocal relationship of low HDL-C levels when TG levels are high and the increase in HDL-C when TG levels decrease. The TG on LDL and HDL are then hydrolyzed by hepatic lipase and lipoprotein lipase leading to the production of small dense LDL and small HDL particles.
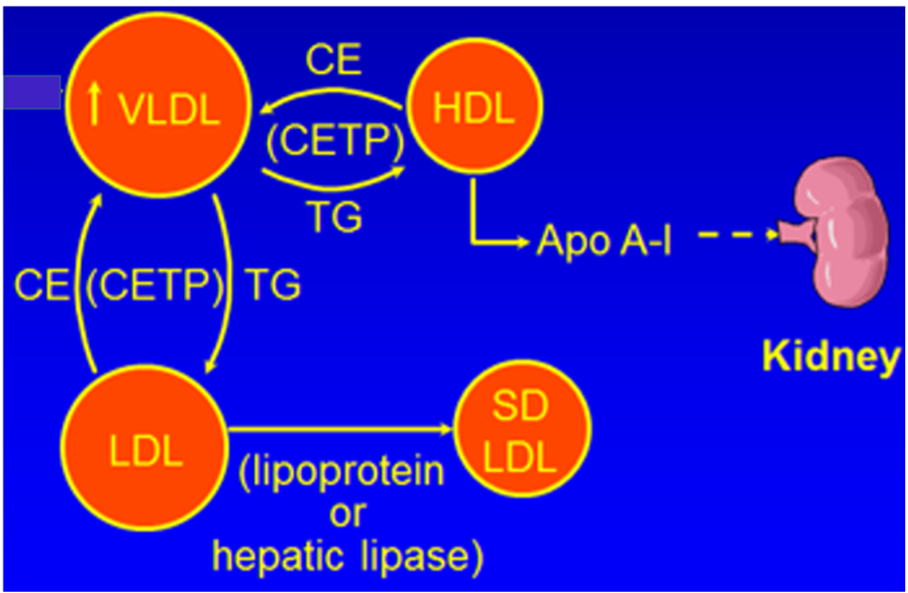
Figure 2. The effect of hypertriglyceridemia on LDL and HDL.
DIETARY PROTEIN
Effect of Dietary Protein on Cardiovascular Disease
In a meta-analysis of 10 studies with 425 ,781 participants intake of plant protein was associated with a decrease in cardiovascular mortality (136). Other meta-analyses have also found that intake of plant proteins was associated with a lower risk of cardiovascular mortality (137-139). In some but not all studies animal protein intake increased the risk of cardiovascular mortality (136-139). The differences in outcomes observed between plant and animal proteins could be due to increased intake of SFA with animal proteins and increased fiber and phytosterol intake with plant proteins.
Effect of Dietary Protein on Lipids
Because a high protein diet is often associated with an increase in SFA intake it is important to control for this variable in determining the effect of dietary protein on lipid levels. In a meta-analysis of a high vs. low protein diets in individuals on a low-fat diet no difference in LDL-C, HDL-C, or TG levels were observed (140). In another meta-analysis of 24 trials with 1,063 participants that compared isocaloric diets matched for fat intake but with differences in protein and CHO intakes no differences in LDL-C and HDL-C levels were observed but TG levels were decreased in the high protein diet group (-20.2mg/dL) (141). Greater weight loss and decreased CHO intake in the high protein diet group likely contributed to the decrease in TGs. In a meta-analysis where fat intake was not controlled the high protein diet was associated with an increase in HDL-C levels and a decrease in TG levels (142). It is obviously difficult to determine the effect of dietary protein on lipid levels as other dietary constituents are changing (SFA, CHO) and secondary effects induced by changes in protein intake (weight loss) could influence lipid levels.
DIETARY FIBER
Dietary fiber are non-digestible carbohydrates including non-starch polysaccharides, cellulose, pectins, hydrocolloids, fructo-oligosaccharides and lignin. Fiber is found mostly in fruits, vegetables, whole grains, nuts, seeds, psyllium seeds, beans, and legumes. There are two main types of dietary fiber; soluble and insoluble. The main sources of soluble fiber are fruits and vegetables and insoluble fiber are cereals and whole-grain products. Most high fiber foods contain both soluble and insoluble fiber. A summary of the fiber content of some foods is shown in tables 8-11.
Table 8. Fiber Content of Selected Vegetables |
||||
---|---|---|---|---|
Vegetables
|
Serving Size |
Total Fiber/ Serving (g) |
Soluble Fiber/ Serving (g) |
Insoluble Fiber/ Serving (g) |
Cooked vegetables |
||||
Turnip |
½ cup |
4.8 |
1.7 |
3.1 |
Peas, green, frozen |
½ cup |
4.3 |
1.3 |
3.0 |
Okra, frozen |
½ cup |
4.1 |
1.0 |
3.1 |
Potato, sweet, flesh |
½ cup |
4.0 |
1.8 |
2.2 |
Brussels sprouts |
½ cup |
3.8 |
2.0 |
1.8 |
Asparagus |
½ cup |
2.8 |
1.7 |
1.1 |
Kale |
½ cup |
2.5 |
0.7 |
1.8 |
Broccoli |
½ cup |
2.4 |
1.2 |
1.2 |
Carrots, sliced |
½ cup |
2.0 |
1.1 |
0.9 |
Green beans, canned |
½ cup |
2.0 |
0.5 |
1.5 |
Beets, flesh only |
½ cup |
1.8 |
0.8 |
1.0 |
Tomato sauce |
½ cup |
1.7 |
0.8 |
0.9 |
Corn, whole, canned |
½ cup |
1.6 |
0.2 |
1.4 |
Spinach |
½ cup |
1.6 |
0.5 |
1.1 |
Cauliflower |
½ cup |
1.0 |
0.4 |
0.6 |
Turnip |
½ cup |
4.8 |
1.7 |
3.1 |
Raw vegetables |
||||
Carrots, fresh |
1, 7 ½ in. long |
2.3 |
1.1 |
1.2 |
Celery, fresh |
1 cup chopped |
1.7 |
0.7 |
1.0 |
Onion, fresh |
½ cup chopped |
1.7 |
0.9 |
0.8 |
Pepper, green, fresh |
1 cup chopped |
1.7 |
0.7 |
1.0 |
Cabbage, red |
1 cup |
1.5 |
0.6 |
0.9 |
Tomato, fresh |
1 medium |
1.0 |
0.1 |
0.9 |
Mushrooms, fresh |
1 cup pieces |
0.8 |
0.1 |
0.7 |
Cucumber, fresh |
1 cup |
0.5 |
0.2 |
0.3 |
Lettuce, iceberg |
1 cup |
0.5 |
0.1 |
0.4 |
Adapted from Anderson JW. Plant Fiber in Foods. 2nd ed. HCF Nutrition Research Foundation Inc, PO Box 22124, Lexington, KY 40522, 1990.
Table 9. Fiber Content of Selected Legumes |
||||
---|---|---|---|---|
Legumes (cooked) |
Serving Size |
Total Fiber/ Serving (g) |
Soluble Fiber/ Serving (g) |
Insoluble Fiber/ Serving (g) |
Kidney beans, light red |
½ cup |
7.9 |
2 |
5.9 |
Navy beans |
½ cup |
6.5 |
2.2 |
4.3 |
Black beans |
½ cup |
6.1 |
2.4 |
3.7 |
Pinto beans |
½ cup |
6.1 |
1.4 |
4.7 |
Lentils |
½ cup |
5.2 |
0.6 |
4.6 |
Black-eyed peas |
½ cup |
4.7 |
0.5 |
4.2 |
Chick peas, dried |
½ cup |
4.3 |
1.3 |
3 |
Lima beans |
½ cup |
4.3 |
1.1 |
3.2 |
Adapted from Anderson JW. Plant Fiber in Foods. 2nd ed. HCF Nutrition Research Foundation Inc, PO Box 22124, Lexington, KY 40522, 1990.
Table 10. Fiber Content of Selected Fruits |
||||
---|---|---|---|---|
Fruits |
Serving Size |
Total Fiber/ Serving (g) |
Soluble Fiber/ Serving (g) |
Insoluble Fiber/ Serving (g) |
Apricots, fresh w/skin |
4 |
3.5 |
1.8 |
1.7 |
Raspberries, fresh |
1 cup |
3.3 |
0.9 |
2.4 |
Figs, dried |
1 ½ |
3 |
1.4 |
1.6 |
Mango, fresh |
½ small |
2.9 |
1.7 |
1.2 |
Orange, fresh |
1 small |
2.9 |
1.8 |
1.1 |
Pear, fresh, w/skin |
½ large |
2.9 |
1.1 |
1.8 |
Apple, red, fresh w/skin |
1 small |
2.8 |
1 |
1.8 |
Strawberries, fresh |
1 ¼ cup |
2.8 |
1.1 |
1.7 |
Plum, red, fresh |
2 medium |
2.4 |
1.1 |
1.3 |
Applesauce, canned |
½ cup |
2 |
0.7 |
1.3 |
Apricots, dried |
7 halves |
2 |
1.1 |
0.9 |
Peach, fresh, w/skin |
1 medium |
2 |
1 |
1 |
Kiwifruit, fresh |
1 large |
1.7 |
0.7 |
1 |
Prunes, dried |
3 medium |
1.7 |
1 |
0.7 |
Grapefruit, fresh |
½ medium |
1.6 |
1.1 |
0.5 |
Blueberries, fresh |
¾ cup |
1.4 |
0.3 |
1.1 |
Cherries, black, fresh |
12 large |
1.3 |
0.6 |
0.7 |
Banana, fresh |
½ small |
1.1 |
0.3 |
0.8 |
Melon, cantaloupe |
1 cup cubed |
1.1 |
0.3 |
0.8 |
Watermelon |
1 ¼ cup cubed |
0.6 |
0.4 |
0.2 |
Grapes, fresh w/skin |
15 small |
0.5 |
0.2 |
0.3 |
Raisins, dried |
2 tbsp |
0.4 |
0.2 |
0.2 |
Adapted from Anderson JW. Plant Fiber in Foods. 2nd ed. HCF Nutrition Research Foundation Inc, PO Box 22124, Lexington, KY 40522, 1990.
Table 11. Fiber Content of Grains |
||||
---|---|---|---|---|
Food |
Serving Size |
Total Fiber/ Serving (g) |
Soluble Fiber/ Serving (g) |
Insoluble Fiber/ Serving (g) |
Wheat bran |
½ cup |
12.3 |
1.0 |
2.7 |
Barley, pearled, cooked |
½ cup |
3.0 |
0.8 |
2.2 |
Oatmeal, dry |
⅓ cup |
2.7 |
1.4 |
11.3 |
Bread, pumpernickel |
1 slice |
2.7 |
1.2 |
1.5 |
Wheat flakes |
¾ cup |
2.3 |
0.4 |
1.9 |
Bread, rye |
1 slice |
1.8 |
0.8 |
1.0 |
Bread, whole wheat |
1 slice |
1.5 |
0.3 |
1.2 |
Rice, white, cooked |
½ cup |
0.8 |
trace |
0.8 |
Bread, white |
1 slice |
0.6 |
0.3 |
0.3 |
Adapted from Anderson JW. Plant Fiber in Foods. 2nd ed. HCF Nutrition Research Foundation Inc, PO Box 22124, Lexington, KY 40522, 1990.
Effect of Dietary Fiber on Cardiovascular Disease
Several meta-analyses have demonstrated that an increase in total fiber, soluble fiber, and insoluble fiber are associated with a decrease in cardiovascular events (143-148). The greater the intake of fiber the greater the reduction in risk of cardiovascular events.
Effect of Dietary Fiber on Lipids
In a meta-analysis of randomized controlled trials the effect of fiber on lipid levels was evaluated (149). Increased dietary fiber decreased total cholesterol (TC) (−7.8mg/dL; 95% CI −13.3 to −2.3), LDL-C (−5.5mg/dL; 95% CI −8.6 to −2.3), and HDL-C levels ( −1.17mg/dL; 95% CI −2.34 to −0.39) (149,150), There was no change in TG levels. A meta-analysis of randomized controlled studies of whole-grain foods vs non-whole-grain foods found that the whole-grain diet lowered LDL-C (-3.51mg/dL; P < 0.01) and TC levels (-4.68mg/dL; P < 0.001) compared with the non-whole grain foods (151). HDL-C and TG levels were not significantly altered by the whole grain diet. Moreover, 3.4 g of psyllium (Metamucil), a soluble fiber, decreased LDL-C with no significant effects on HDL-C or TGs (152,153). In a meta-analysis of 28 randomized trials psyllium lowered LDL by 12.9mg/dL (P < 0.00001) (154). A mean reduction in LDL-C concentrations of about 1.1 mg/dL can be expected for each g of water-soluble fiber in the diet (155,156).
MECHANISM OF EFFECT OF FIBER ON LDL-C
Fiber is thought to decrease cholesterol absorption by the small intestine (157,158). This leads to a decrease in cholesterol content of chylomicrons and a reduction in the delivery of cholesterol to the liver. The decrease in cholesterol in the liver upregulates LDL receptors resulting in a decrease in plasma LDL-C levels. Fiber may also decrease small intestinal absorption of bile acids which will lead to the increased utilization of hepatic cholesterol for the synthesis of bile acids (159). This will also decrease hepatic cholesterol levels inducing an increase in the expression of LDL receptors lowering plasma LDL-C levels. Finally, colonic fermentation of dietary fiber with production of short-chain fatty acids, such as acetate, propionate, and butyrate, is postulated to inhibit hepatic cholesterol synthesis contributing to a decrease in LDL-C levels (159).
PLANT STEROLS AND STANOLS (PHYTOSTEROLS)
Plant sterols and plant stanols (phytosterols) are naturally occurring constituents of plants and are found in vegetable oils, such as corn oil, soybean oil, and rapeseed oil and cereals, nuts, fruits, and vegetables. The intake of plant sterols and stanols is about 200–400 mg/day. The most commonly occurring phytosterols in the human diet are β-sitosterol, campesterol, and stigmasterol. Higher intakes can be achieved by consuming a vegetable-based diets such as a vegetarian diet (400-800mg/day) or by consuming food products enriched with plant sterols or stanols (for example margarines or yogurt). If using foods enriched in phytosterols it is best to take them with main meals to enhance their effectiveness. High doses of phytosterols can affect the absorption of fat-soluble vitamins. The plant sterol and stanol content of different foods is shown in table 12.
Table 12. Plant Sterol and Stanol Contents in Different Foods |
||
---|---|---|
Food item |
Plant Sterols (mg/100 g) |
Plant Stanols (mg/100 g) |
Vegetable oils |
||
Corn oil |
686-952 |
23-33 |
Rapeseed oil (canola oil) |
250-767 |
2-12 |
Soybean oil |
221-328 |
7 |
Sunflower oil |
263-376 |
4 |
Olive oil |
144-193 |
0.3-4 |
Palm oil |
60-78 |
Traces |
Cereals |
||
Corn |
66-178 |
- |
Rye |
71-113 |
12-22 |
Wheat |
45-83 |
17 |
Barley |
80 |
2 |
Millet |
77 |
- |
Rice |
72 |
3 |
Oats |
35-61 |
1 |
Vegetables |
||
Broccoli |
39 |
2 |
Cauliflower |
18-40 |
Traces |
Carrot |
12-16 |
Traces |
Lettuce |
9-17 |
0.5 |
Potato |
7 |
0.6 |
Tomato |
7 |
1 |
Fruits and berries |
||
Avocado |
75 |
0.5 |
Passion fruit |
44 |
Not detected |
Raspberry |
27 |
0.2 |
Orange |
24 |
Not detected |
Apple |
12-18 |
0.8 |
Banana |
12-16 |
Not detected |
Adapted from Piironen V and Lampi AM (160)
Effect of Phytosterols on Cardiovascular Disease
There is minimal data on the effect of phytosterols on cardiovascular events. From the effect on LDL-C levels one would anticipate that phytosterols would reduce CVD.
Effect of Phytosterols on Lipids
Plant sterols or plant stanols at a dose of 3 grams per day lowers LDL-C by approximately 12% (161). Higher doses do not dramatically further lower LDL-C levels and lower doses have less effect on LDL-C (for example 2 grams/day lowers LDL-C by 8%) (161). HDL-C levels are not affected by plant sterols or stanols but TG levels decrease modestly (~6%) with a greater absolute reduction in individuals with high TG level (percent change is the same) (162). To achieve these high doses consuming food products enriched is phytosterols is necessary.
MECHANISM OF EFFECT OF PHYTOSTEROLS ON LDL-C
Plant sterols or plant stanols reduce LDL-C levels by competing with cholesterol for incorporation into micelles in the gastrointestinal tract, resulting in decreased cholesterol absorption (163). This leads to the decreased delivery of cholesterol to the liver and the up-regulation of LDL-receptor expression lowering LDL-C levels.
SUMMARY OF THE EFFECT OF DIETARY CONSTITUENTS ON LIPID LEVELS
A summary of the major effects of dietary constituents on lipid levels is shown in table 13, typically under isocaloric feeding conditions in short-term feeding studies. Dietary SFA, TFA, and cholesterol increase LDL-C levels whereas CHO increases TG levels. MUFA, PUFA, fiber and phytosterols decrease LDL-C and TFA decrease HDL-C levels.
Table 13. Summary of the Effect of Dietary Constituents on Lipid and Lipoproteins |
|
---|---|
SFA |
Increase LDL-C and modest increase HDL-C |
MUFA and PUFA |
Decrease LDL-C |
TFA |
Increase LDL-C and decrease HDL-C |
Cholesterol |
Increase LDL-C |
CHO |
Increase TGs, increase greater with simple sugars particularly fructose |
Fiber |
Decrease LDL-C |
Phytosterols |
Decrease LDL-C |
EFFECT OF SPECIFIC FOODS ON CARDIOVASCULAR DISEASE
There are a large number of observational trials linking various foods with either an increased or decreased risk of CVD. A large meta-analysis by Micha et al reported that fruits, vegetables, beans/legumes, nuts/seeds, whole grains, fish, yogurt, fiber, seafood omega-3 fatty acids, polyunsaturated fats, and potassium were associated with a decreased risk of CVD while unprocessed red meats, processed meats, sugar-sweetened beverages, and sodium were associated with an increased risk of CVD (164). A similar meta-analysis by Bechthold et al found that whole grains, vegetables and fruits, nuts, and fish consumption were associated with a decrease in CVD while red meat, processed meat, and sugar sweetened beverage consumption was associated with an increase in CVD (165). Note, as discussed in the introduction, observational studies have limitations and cannot be assumed to indicate cause and effect. Additional one can find other meta-analyses that reach different conclusions than the results described above. For example, a meta-analysis by Zeraatkar et al and a meta-analysis by Vernooij et al reached the conclusion that meat and processed meat were not associated with a significant increase in CVD (20,166). Thus, one needs recognize that while these studies can suggest beneficial and harmful effects of eating certain foods more definitive studies are required to be certain. For a detailed analysis of the limitations of observational dietary studies see articles by Ioannidis and Nissen (1,2).
Only a single randomized trial has examined the effect of specific foods on CVD events. The DART trial randomized men with an acute myocardial infarction to at least two weekly portions (200-400 g) of fatty fish (mackerel, herring, kipper, pilchard, sardine, salmon, or trout) (n=1015) or no dietary advice (n=1018) (50). After approximately 2 years total mortality was significantly lower (RR 0.71; CI 0.54-0.93) in the fish advice group than in the no fish advice group, due to a reduction in ischemic heart disease deaths. There were no significant differences in ischemic heart disease events (RR 0.84; CI 0.66-1.07). In a separate portion of the DART trial there was also a group of men with an acute myocardial infarction randomized to increased intake of cereal fiber (18 grams/day) (n=1017) vs. no dietary advice (n=1016). No reduction in cardiovascular events was seen in the cereal fiber group.
Clearly addition randomized trials are required to determine the true benefits of specific foods on cardiovascular events.
EFFECT OF SPECIFIC FOODS ON LIPID LEVELS
In contrast to the paucity of randomized controlled trials on the effect of specific foods on cardiovascular disease there are an abundance of studies on the effect of specific foods on lipid and lipoprotein levels. Given the large number of studies in many instances I will cite the results of meta-analyses to provide the reader with the typical effects that are observed. It should be noted that the effect of specific foods on lipid and lipoprotein levels tend to be small and therefore the results can be inconsistent from study to study.
Nuts and Seeds
The most consumed edible tree nuts are almonds, hazelnuts, walnuts, pistachios, pine nuts, cashews, pecans, macadamias, and Brazil nuts. Peanuts are botanically groundnuts or legumes, and are widely considered to be part of the nut food group. Nuts are generally consumed as snacks (fresh or roasted), in spreads (peanut butter, almond paste), or as oils or baked goods. Seeds come in all different sizes, shapes and colors. Popular seeds include flax, pumpkin, sunflower, chia, sesame, and mustard seeds.
Nuts and seeds are rich in MUFAs, such as oleic acid and in PUFAs, such as linoleic acid and alpha-linolenic acid (ALA). They also contain small amounts of SFA. Almonds, cashews, hazelnuts, pistachios and macadamian nuts have a high MUFA content (>50%) content when compared with other nuts. For other nuts (e.g., Brazil nuts, pine nuts, and walnuts) the PUFA content is high (>50%), while peanuts and pecans have been found to contain relatively high levels of both MUFA and PUFA (table 14). Nuts are a good source of dietary fiber, ranging from 4-11 g/100 g and phytosterols.
Table 14. Nutrient Composition of Nuts |
||||
---|---|---|---|---|
Nuts
|
PUFA (g/100 g) |
MUFA (g/100 g) |
SFA (g/100 g) |
Fiber (g/100 g) |
Walnuts |
47.2 |
8.9 |
6.1 |
6.7 |
Peanuts |
15.6 |
24.4 |
6.3 |
8.8 |
Pistachios |
13.7 |
23.8 |
5.6 |
10.3 |
Almonds |
12.3 |
31.6 |
3.8 |
12.5 |
Hazelnuts |
7.9 |
45.7 |
4.5 |
9.7 |
Cashews |
7.8 |
23.8 |
7.8 |
3.3 |
Pecans |
21.6 |
40.8 |
6.2 |
9.6 |
Macadamias |
1.5 |
58.9 |
12.1 |
8.6 |
Consumption of nuts and seeds lower TC and LDL-C levels in healthy subjects or patients with moderate hypercholesterolemia (167-172). Nuts had no significant or minimal effect on increasing HDL-C. The benefits of nuts and seeds vary depending on the type, nutrient composition, and quantity of nuts and seeds consumed. Studies have noted that the estimated cholesterol lowering effect of nuts was greater in individuals with higher initial values of LDL-C and in those with a lower baseline BMI (169).
Walnuts: A meta-analysis on the effect of walnuts on lipid levels that included 365 participants showed a decrease in LDL-C (9.2 mg/dL), while HDL-C or TG were not significantly affected (173). In another meta-analysis that analyzed 1,059 participants with a walnut enriched diet LDL-C was lowered by 5.5 mg/dL (174).
Almonds: A meta-analysis of 15 studies with 534 participants found that almonds decreased LDL cholesterol (5.8 mg/dL; 95% CI: -9.91, -1.75 mg/dL) and apo B (6.67 mg/dL; 95% CI: -12.63, -0.72 mg/dL) (175). Triglycerides, apo A1, and lipoprotein (a) showed no differences.
Pistachio nuts: A meta-analysis of twelve randomized studies reported that pistachio nuts decreased LDL-C -3.82 mg/dL (95% CI, -5.49 to -2.16) and TG -11.19 mg/dL (95% CI, -14.21 to -8.17) levels without effecting HDL-C levels (176).
A meta-analysis by Houston et al analyzed the effect of a variety of different nuts on lipid levels (table 15) (177). They found that in general nuts lowered LDL-C and minimally lowered TG levels but had no effect on HDL-C levels. A meta-analysis found that whole flaxseed reduced TC and LDL-C by 6 and 8 mg/dL, respectively (178). Thus, both nuts and seeds lower LDL-C levels.
Table 15. Effect of Nuts on Lipid Levels |
|||
---|---|---|---|
|
Number of analyses |
Number of participants |
Effect estimate (mmol/L) 95% CI |
LDL Cholesterol |
|||
Almond |
32 |
2439 |
-0.15 [-0.22, -0.08] |
Brazil nut |
4 |
307 |
-0.30 [-0.70, 0.11] |
Cashew nut |
3 |
432 |
0.02 [-0.12, 0.16] |
Hazelnut |
6 |
374 |
-0.01 [-0.15, 0.12] |
Macadamia |
6 |
410 |
-0.11 [-0.27, 0.04] |
Mixed nuts |
10 |
791 |
0.04 [-0.06, 0.14] |
Peanut |
10 |
1021 |
0.08 [-0.04, 0.20] |
Pecan |
6 |
295 |
-0.23 [-0.46, 0.00] |
Pistachio |
12 |
736 |
-0.15 [-0.30, 0.00] |
Walnut |
35 |
2582 |
-0.12 [-0.18, -0.06] |
Triglycerides |
|||
Almond |
32 |
2439 |
-0.02 [-0.05, 0.02] |
Brazil nut |
4 |
307 |
0.04 [-0.54, 0.63] |
Cashew nut |
3 |
432 |
-0.02 [-0.11, 0.07] |
Hazelnut |
5 |
313 |
0.11 [-0.02, 0.25] |
Macadamia |
5 |
342 |
-0.10 [-0.21, 0.00] |
Mixed nuts |
11 |
888 |
-0.01 [-0.07, 0.06] |
Peanut |
10 |
1021 |
-0.09 [-0.16, -0.02] |
Pecan |
6 |
295 |
-0.11 [-0.24, 0.03] |
Pistachio |
9 |
498 |
-0.12 [-0.21, -0.03] |
Walnut |
35 |
3109 |
-0.09 [-0.12, -0.06] |
Table based on data from a meta-analysis by Houston et al (177). To convert mmol/L cholesterol to mg/dL multiply by 39 and to convert mmol/L triglycerides to mg/dL multiply by 88.
Whole Grains
Whole grains include barley, brown rice, buckwheat, bulgur (cracked wheat), millet, oatmeal, and wild rice. Whole grains contain ~80% more dietary fiber than refined grains, as the latter are milled, a process that removes bran and germ. Refined grains include white flour, white rice, white bread, and corn flower. Health benefits ascribed to whole grains are mainly due to the presence of fiber and bran. A meta-analysis of fifty-five trials with 3900 participants comparing various grains found that oat bran was the most effective intervention strategy for lowering LDL-C (- 12.5mg/dL; 95% CI – 17.2 to – 7.4mg/dL) compared with control (179). Oats also reduced LDC (- 6.6mg/dL; 95% CI – 10.9 to 2.73mg/dL). Barley, brown rice, wheat and wheat bran were not effective in improving blood lipid levels compared with controls. Another meta-analysis also found that whole-grain oats decreased LDL-C levels (–16.7 mg/dL; P < 0.0001) (180).
Soy Protein
Soybeans and soy products as well as supplements contain soy proteins. In a meta-analysis of 43 randomized studies with 2,607 participants the decrease in LDL-C levels reductions for soy protein ranged between −4.2 and −6.7 mg/dL (P<0.006) (181). Numerous other meta-analyses have reported similar decreases in LDL-C (182-187). In addition, soy protein also decreases TG levels (~2-10mg/dL) and increases HDL-C levels (~1-2mg/dL). Soy protein does not affect Lp(a) levels (188). The amount of soy protein that is recommended for lipid lowering is 25–50 grams per day (189).
The decrease in LDL-C is due to the indirect effect of soy protein decreasing the intake of animal protein (SFA and cholesterol) and the intrinsic effects of bioactive compounds in soy protein (190). The intrinsic effect of soy protein might be mediated by phyto-estrogens that could increase levels of HDL-C and TG and decrease levels of LDL-C (189).
Garlic
Garlic supplements are available in several different forms, including garlic powder, allicin, aged garlic extract, and garlic oil. Several meta-analyses have shown that garlic lowers TC levels with variable effects on LDL-C, HDL-C, and TG (191-198). Some studies find a decrease in LDL-C and others a decrease in TG levels. The longer the duration of treatment and the higher the baseline TC the greater the effect. In one meta-analysis TC was reduced by 17 ± 6 mg/dL and low-density lipoprotein cholesterol by 9 ± 6 mg/dL in individuals with elevated TC levels (>200 mg/dL) if treated for longer than 2 months (191). In another meta-analysis garlic powder and aged garlic extract were more effective in reducing TC levels, while garlic oil was more effective in lowering serum TG levels (192). In a meta-analysis of garlic administration to patients with diabetes TC decreased 16.9mg/dL, LDL decreased 9.7mg/dL, TG decreased 12.4mg/dL, and HDL-C increased 3.19mg/dL (all p=0.001) (199). Lp(a) levels are not altered by garlic (198).The mechanism by which garlic alters lipid levels is unknown.
Tea
Green tea contains many catechins (e.g., epigallocatechin-3-gallate) that influence lipid metabolism in animal models and have been shown to upregulate LDL receptors in liver and suppress PCSK9 production (200,201). Epigallocatechin gallate may also interfere with the intestinal absorption of lipids (202). Most but not all meta-analyses have shown that drinking green tea or black tea decreases TC and LDL-C levels with no significant effect on HDL-C or TG levels (203-214). The reduction in LDL-C is approximately 5-10mg/dL.
Coffee
Coffee contains cholesterol-increasing compounds; diterpenes such as cafestol and kahweol (215,216). The amount of these cholesterol increasing compounds in coffee depends on how the coffee is prepared (215,216). Boiling coffee beans extracts diterpenes due to the prolonged contact with hot water resulting in high concentrations in the coffee whereas brewed filtered coffee because of the short contact with hot water and retention of diterpenes by the filter paper has lower concentrations of diterpenes. Instant coffee has very low levels of diterpenes (216). The concentration of the cholesterol-raising compound cafestol is negligible in drip-filtered, instant, and percolator coffee but high in unfiltered coffee such as French press, Turkish, or Scandinavian boiled coffee. Levels of cafestol are intermediate in espresso and coffee made in a Moka pot.
A meta-analysis of 18 trials found that the consumption of unfiltered, boiled coffee dose-dependently increased TC and LDL-C concentrations (23 mg/dL and 14 mg/dL, respectively), while consumption of filtered coffee resulted in only small changes (TC increased by 3 mg/dL and no effect on LDL-C concentration) (217). Additionally, decaffeinated coffee had a smaller effect and the increase in cholesterol levels was greatest in individuals with hypercholesterolemia. Thus coffee, depending upon how it is prepared, can increase TC and LDL-C levels.
Chocolate and Cocoa
Cocoa is the non-fat component of finely ground cocoa beans that is used to produce chocolate. Cocoa is rich in flavanols which are low‐molecular‐weight monomeric compounds, such as epicatechin or complex higher‐molecular‐weight oligomeric and polymeric compounds (218). The flavanol content in cocoa products can vary greatly and is dependent on the crop type, post‐harvest handling practices, and manufacturer processing techniques. The flavanol content of milk and white chocolate is low or even absent (218).
In a meta-analysis of 21 studies with 986 participants very small effects on LDL-C and HDL-C levels were observed (LDL-C 2.7mg/dL decrease; HDL-C 1.2mg/dL increase) with no change in TG levels with chocolate and/or cocoa intake (219). In another meta-analysis there was a decrease in TG levels (-8.8mg/dL), an increase in HDL-C (2.3mg/dL), and a non-significant decrease in LDL-C (-10.1mg/dL) (220). In studies where the epicatechin dose was greater than 100mg per day the decrease in LDL-C levels was greater (5.5mg/dL) (219). Another meta-analysis of 19 studies found that LDL decreased by 3.3mg/dL and HDL-C increased by 1.8mg/dL with cocoa intake (221). A meta-analysis of 10 clinical trials with 320 participants that focused on dark chocolate found a 6.23mg/dl decrease in LDL-C with no significant changes in HDL-C and TG (222). Thus chocolate/cocoa causes a small decrease in LDL-C levels.
Alcohol
It is recommended that females consume no more than 1 drink per day of alcohol (equivalent to 15 grams per day) and that males consume no more than 2 drinks per day (equivalent to 30 grams per day). Alcohol has a relatively high caloric level (7 calories/gram).
EFFECT OF ALCOHOL ON LIPID LEVELS
In a meta-analysis of 25 studies with an average consumption of 40.9 grams of alcohol per day HDL-C concentrations increased by 5.1 mg/dL (223). HDL-C levels increased by 0.122- 0.133 mg/dL per gram of alcohol per day. Consuming 30 grams of alcohol a day would therefore increase HDL-C concentrations by approximately 3.99 mg/dL compared with an individual who abstains (an 8.3% increase from pretreatment values). The increase in HDL-C was observed regardless of sex, duration of study, median age, or beverage type but the increase was greater in individuals with baseline HDL-C < 40mg/dL and who were sedentary. As expected apo A1 levels also increased. In a meta-analysis of 35 studies TG concentrations increased by 0.19 mg/dL per gram of alcohol consumed a day (P=0.001) and 5.69 mg/dL per 30 g consumed per day (5.9% increase over baseline) (223). The increase in TG levels was seen regardless of beverage type and appeared to be greater in males than females.
In a more recent meta-analysis of 33 studies with 796 participants HDL-C levels were increased by 3.67mg/dL by alcohol intake (224). Apo A1 levels were also increased but there were no significant differences in TC, LDL-C, TG, or Lp(a) with alcohol intake. The greater the consumption of alcohol the greater the increase in HDL-C levels. When the consumption of alcohol was greater than 60 grams per day (4 drinks) TG levels were also increased (24.4mg/dL).
In a meta-analysis of 14 studies, comparing 548 beer drinkers and 532 controls TC levels were significantly higher in the beer drinkers compared to controls (difference 3.52 mg/dL; p<0.001) (225). In a meta-analysis of 18 studies, comparing 626 beer drinkers and 635 controls HDL-C levels were higher in the beer drinkers compared to controls (difference 3.63 mg/dL: p<0.001) (225). This increase in HDL-C levels in beer drinkers were seen in both males and females. LDL-C and TG levels were not significantly different between beer drinkers and controls (LDL-C difference -2.85 mg/dL; p = 0.070; TG difference 0.40 mg/dL; p = 0.089) (225).
Genetic factors play a role in the HDL response to alcohol (226). Individuals with an apoE2 allele have greater HDL-C increase and those with an apoE4 allele have a blunted increase in HDL-C with alcohol intake (226).In addition to an increase in HDL-C levels studies have suggested that the ability of HDL to facilitate the efflux of cholesterol from cells is enhanced by alcohol intake (226,227).
One should note in the meta-analyses described above alcohol doesn’t appear to have a major impact on TG levels. However, it must be recognized that the amount of alcohol consumed is a key variable (228,229). At low to moderate amounts alcohol has either no effect or might even decrease TG levels (228). However, at high amounts of alcohol intake increases in TG levels are observed (228,229). As noted above one meta-analysis noted that the consumption of 60 grams per day of alcohol increased TG levels (224). Moreover, alcohol consumed with a meal increases and prolongs the postprandial increase in TG levels (228,229). Additionally, genetic factors and the presence of other abnormalities play a role in the TG response to alcohol intake (229). For example, the increase in TG levels after red wine was -4%, 17%, and 33% in individuals with a BMI 19.60-24.45, 24.46- 26.29, and 26.30-30.44, respectively (P = .001) demonstrating that the increase in TG was strongly influenced by BMI (230). Finally, in patients with pre-existing hypertriglyceridemia moderate alcohol intake increased TG levels (338 ± 71 mg/dL to 498 ± 117 mg/dL; P < 0.05) (231).
MECHANISM FOR THE EFFECT OF ALCOHOL ON HDL
The mechanism for the increase in HDL-C levels is likely due to an increased production of apo A1 and A2 (232). Additionally, alcohol inhibits cholesteryl ester transfer protein (CETP) activity, which will also increase HDL-C levels (229).
MECHANISM FOR THE EFFECT OF ALCOHOL ON TRIGLYCERIDES
Alcohol increases VLDL secretion by the liver (229). The increased production and secretion of VLDL is due to a number of factors including a) alcohol increases lipolysis in adipose tissue and increases the delivery of fatty acids to the liver b) alcohol increases hepatic fatty acid transporters increasing the uptake of circulating fatty acids c) alcohol increases hepatic de novo fatty acid synthesis d) alcohol decreases the beta oxidation of fatty acids in the liver (229,233,234). Together these effects lead to an increased supply of fatty acids in the liver facilitating TG synthesis and the formation and secretion of VLDL.
While moderate alcohol intake increases lipoprotein lipase (LPL) activity acute alcohol intake inhibits LPL activity, which could explain the observation that alcohol consumed with a meal increases postprandial TG levels ((228,229).
Summary of the Effect of Specific Foods on Lipid Levels
Table 16. Major Effects of Specific Foods on Lipid Levels |
|
---|---|
Nuts and Seeds |
Decrease TC and LDL-C |
Whole Grains |
Decrease LDL-C |
Garlic |
Decrease TC, LDL-C, TG |
Green and Black Tea |
Decrease TC and LDL-C |
Coffee (depends on method of preparation) |
Increase TC, LDL-C, TG |
Cocoa and Chocolate |
Decrease LDL-C |
SPECIFIC DIETS
The effect of several dietary strategies on lipid levels is discussed below. Randomized controlled trials on the effect of specific diets on cardiovascular outcomes were discussed in earlier sections (saturated fatty acids section and monounsaturated fatty acids section).
Mediterranean Diet
Mediterranean diets have an abundance of plant foods, including vegetables, legumes, nuts, fruits, and grains, fish, and low to moderate red wine consumption. Low consumption of meat and meat products and moderate consumption of milk and dairy products is encouraged. In the PREDIMED trial the Mediterranean diet resulted in a small but significant increase in HDL-C levels and a small decrease in both LDL-C and TG levels (76). In the Lyon Diet Heart Study lipid levels were similar in the Mediterranean and usual diet groups (77). The cardiovascular outcome benefits of both of these randomized outcome trials are discussed in the effect of MUFA on CVD section. In a meta-analysis of the effect of a Mediterranean diet on lipid levels little or no change in LDL-C, HDL-C, and TGs was observed (235). Another meta-analysis reported a 4.6mg/dL decrease in LDL-C and a 0.61mg/dL increase in HDL-C (236).
Dietary Approach to Stop Hypertension (DASH) Diet
The DASH diet promotes the consumption of fruits, vegetables, low-fat dairy products, whole grains, poultry, fish, and nuts and a decrease in the intake of red meat, sweets, sugar-containing beverages, total fat, saturated fat, and cholesterol. In the initial DASH trial total fat and SFA intake was reduced in the DASH diet group (total fat 27% vs. 39% of calories; SFA 6.2% vs. 15% of calories). MUFA and PUFA intake were similar but cholesterol intake was decreased (194mg/day vs 324mg/day). As expected, CHO and fiber intake were increase (CHO 59% vs. 49% of calories; fiber 35grams/day vs. 17grams/day). The DASH diet lowered TC (15.6 to 19.5mg/dL), LDL-C (11.7 to 15.5mg/dL), and HDL-C (3.12 to 3.90mg/dL) (237). TG levels were not significantly affected. In a meta-analysis of twenty studies of the DASH diet reporting data for 1917 participants TC was decreased (7.8mg/dL; P=0.001) and LDL was decreased (3.9mg/dL; p<0.03) (238). HDL-C and TG levels were not significantly altered (238). Similar results were seen in other meta-analyses (239,240).
Portfolio Diet
The portfolio dietary pattern is a plant-based dietary pattern that includes four cholesterol-lowering foods; a) tree nuts or peanuts, b) plant protein from soy products, beans, peas, chickpeas, or lentils, c) viscous soluble fiber from oats, barley, psyllium, eggplant, okra, apples, oranges, or berries, and d) plant sterols initially provided in a plant sterol-enriched margarine. In a meta-analysis of 5 studies with 439 participants LDL-C was lowered by 17% (28.5mg/dL; p< 0.001) and TGs by 16% (24.6mg/dL; p< 0.001) with no change in HDL-C or weight (241).
Nordic Diet
The Nordic diet is based on the consumption of different healthy foods such as whole grains, fruits (such as berries, apples, and pears), vegetables, legumes (such as oats, barley, and almonds), rapeseed oil, fatty fish (such as salmon, herring and mackerel), shellfish, seaweed, low-fat choices of meat (such as poultry and game), low-fat dairy, and decreased intake of salt and sugar-sweetened products. In a meta-analysis of 5 studies LDL-C was decreased by 11.7mg/dL (p= 0.013) with no changes in TG or HDL-C levels (242). In another meta-analysis of 6 studies LDL-C was decreased by 10.1mg/dL with no changes in TG or HDL-C levels (243).
Ketogenic Diet
Low CHO diets can contain variable amounts of CHO. When the CHO levels are very low, they stimulate the formation of ketones. In a typical ketogenic diet CHO contribute <10% of calories (< 50 grams/day), protein approximately 30% of calories, and fat approximately 60% of calories with no restrictions on the type of fat or cholesterol levels. These diets can be high in beef, poultry, fish, oils, various nuts/seeds, and peanut butter, with moderate amounts of vegetables, salads with low-carbohydrate dressing, cheese, and eggs. Fruits and fruit juices, most dairy products with the exception of hard cheeses and heavy cream, breads, cereals, beans, rice, desserts/sweets, or any other foods containing substantial amounts of CHO are avoided.
It is well recognized that a ketogenic diet results in an increase in LDL-C levels, which varies depending upon the type of fat ingested, the degree of carbohydrate restriction, the presence of other medical conditions, weight loss on the diet, and genetic background (244). This increase in LDL-C levels is best illustrated in children treated with a ketogenic diet for epilepsy and in healthy individuals on a ketogenic diet (245-250). In some studies HDL-C is also increased (246-249). A meta-analysis of randomized studies in normal-weight adults found that a ketogenic diet increased LDL-C by 42mg/dL and HDL-C by 13.7mg/dL with no significant changes in TG levels (251). It should be noted that the increase in LDL-C is often not observed or is modest in patients with obesity or the metabolic syndrome (252,253).
While the typical increases in LDL-C levels observed with a ketogenic diet are relatively modest, recently a series of reports have described marked elevations in LDL-C levels in some patients on a ketogenic diet (253-255). For example, Goldberg et al reported 5 patients with marked increases in LDL-C levels on a ketogenic diet (256). Three patients had LDL-C levels greater than 500mg/dL. Similarly, Schaffer et al described 3 patients in which a very low carbohydrate diet induced LDL-C levels greater then 400mg/dL (257). Finally, Schmidt et al reported 17 patients with LDL-C levels greater than 200mg/dL on a ketogenic diet (258). In these patients there was an average increase in their LDL-C level of 187 mg/dL (258). The elevations in LDL-C levels decrease towards normal with cessation of the ketogenic diet (256-258). It should be noted that most of the patients with marked elevations in LDL-C in response to a ketogenic diet had normal LDL-C levels prior to the dietary change (255).
Many of the individuals who develop marked increases in LDL-C on a very low carbohydrate ketogenic diet have low TG levels, elevated HDL-C levels, and are thin (253,255). This phenotype has been called the lean mass hyper-responder (LMHR) phenotype (253,255). LMHR individuals have been defined as having TG <70mg/dL, HDL-C > 80mg/dL, and LDL-C > 200mg/dL (253,255). The mechanism for the marked increase in LDL-C levels is unknown. It may be due to a genetic predisposition in certain individuals (Apo E2/E2 genotype or high polygenic risk score for hypercholesterolemia) (256). Therefore, it is important for clinicians to monitor lipid levels in patients electing to follow a very low CHO/high fat diet.
Comparison of Low Fat vs. Low Carbohydrate Weight Loss Diets
Numerous randomized studies have compared the effect of low fat vs. low CHO weight loss diets on lipid levels. In a study by Foster et al 154 obese individuals were randomized to a low-fat diet and 153 obese individuals to a low CHO diet (259). In the low CHO diet during the first 12 weeks of treatment participants were instructed to limit CHO intake to 20 grams/day in the form of low–glycemic index vegetables after which the diet was gradually liberalized. In the low-fat diet participants were instructed to limit energy intake with approximately 55% of calories from CHO, 30% from fat, and 15% from protein. Participants were instructed to limit calorie intake, with a focus on decreasing fat intake. After 6 months weight loss was similar in both diet groups. The effect on lipid levels at 6 months is shown in table 17. As one would expect the low CHO was very effective at lowering TG levels and increasing HDL-C levels while the low-fat diet was very effective at lowering LDL-C levels. The large weight loss in this trial may have contributed to the large reduction in lipid levels. A review of a large number of meta-analyses comparing a low CHO diet vs. low fat weight loss diet similarly described that the low CHO diet lowered TG levels and increased HDL-C and LDL-C levels compared to the low-fat diet (244). Note the increase in LDL-C with the low-CHO diet was blunted in patients with diabetes or pre-diabetes (244). Also, the increase in LDL-C levels is likely to be greater in low CHO diets that are enriched in SFA (244).
Table 17. Comparison of Low Fat vs. Low Carbohydrate Weight Loss Diet on Lipid Levels |
|||
---|---|---|---|
|
Low Fat Diet |
Low Carbohydrate Diet |
|
Weight |
-11.3kg |
-12.2kg |
NS |
TGs |
-24mg/dL |
-49mg/dL |
P<0.001 |
LDL-C |
-9.5mg/dL |
0.5mg/dL |
P<0.001 |
HDL-C |
1.0mg/dL |
6.2mg/dL |
P<0.001 |
Comparison of Vegetarian and Omnivore Diet on Lipid Levels
Vegetarian diets exclude all animal flesh. A meta-analysis of 19 studies comparing a vegetarian vs. omnivore diet found that consumption of vegetarian diets resulted in a 12.2mg/dL decrease in LDL-C (p < 0.001) and 3.4mg/dL decrease in HDL-C (p < 0.001) and a nonsignificant increase in TG levels (5.8 mg/dL; P = 0.090) compared with consumption of an omnivorous diet (260). Vegan diets, which exclude all animal products, were associated with larger LDL-C reductions than lacto-ovo vegetarian diets. A meta-analysis of 11 clinical trials comparing a vegetarian vs. omnivore diet observed similar results (LDL‐C decreased 13.3mg/dL ; P<0.001; HDL decreased 3.9mg/dL; P<0.001) (261). It is likely that a decrease in dietary SFA and cholesterol and an increase in dietary fiber and phytosterols account for the differences in a vegetarian and omnivore diets.
Comparison of 14 Different Diets on Lipid Levels
In a network meta-analysis of 121 eligible trials with 21, 942 overweight or obese patients Ge and colleagues compared the effect of 14 different diets on LDL-C and HDL-C levels (236). The diets could be grouped into low CHO diets (Atkins, South Beach, Zone), moderate macronutrients diets (Biggest Loser, DASH, Jenny Craig, Mediterranean, Portfolio, Slimming World, Volumetrics, Weight Watchers), and low-fat diets (Ornish, Rosemary Conley). The effect of these different diets on LDL-C and HDL-C levels are shown in table 18. It should be noted that despite considerable weight loss the effect of these diets on LDL-C and HDL-C levels was very modest except for the LDL-C lowering seen with the Portfolio diet. Unfortunately, a comparison of the effect of these diets on TG levels was not reported.
Table 18. Effect of Different Diets in Comparison with Usual Diet |
|||
---|---|---|---|
Diet vs. Usual Diet |
Decrease in Weight (Kg) |
Change in LDL-C (mg/dL) |
Change in HDL-C (mg/dL) |
Atkins |
5.46 |
+2.75 |
-3.41 |
Zone |
4.07 |
+2.89 |
+0.33 |
Dash |
3.63 |
-3.93 |
+1.90 |
Mediterranean |
2.87 |
-4.59 |
+0.61 |
Paleolithic |
5.31 |
-7.27 |
+2.52 |
Low Fat |
4.87 |
-1.92 |
+2.13 |
Jenny Craig |
7.77 |
-0.21 |
+2.85 |
Volumetrics |
5.95 |
-7.13 |
+0.13 |
Weight Watchers |
3.90 |
-7.13 |
+0.88 |
Rosemary Conley |
3.76 |
-7.15 |
+2.04 |
Ornish |
3.64 |
-4.71 |
+4.87 |
Portfolio |
3.64 |
-21.29 |
+3.26 |
Biggest Loser |
2.88 |
-3.90 |
+0.01 |
Slimming World |
2.15 |
N/A |
N/A |
South Beach |
9.86 |
+0.64 |
-3.60 |
Dietary Advice |
0.31 |
+2.01 |
+1.71 |
Summary of results of popular named diets network meta-analysis for outcomes at six months
In a study carried out in a single center the Atkins, Zone, Weight Watchers, and Ornish diets were compared and the effect on TG levels was also reported (262). Table 19 shows the results of this study at 2 months, a period at which dietary compliance was still high. The magnitude of weight loss was similar but the decrease in LDL-C that occurs with weight loss was blunted with a diet that was high in fat (Atkins diet). In contrast HDL-C levels increased with a high fat diet, particularly SFA (Atkins diet) and decreased with a very low-fat diet (Ornish diet). The weight loss induced decrease in TG levels was blunted by a high CHO intake (Ornish diet). These observations confirm and extend the results described above.
Table 19. Effect of Different Diets on Lipid Levels |
||||
---|---|---|---|---|
|
Weight (kg) |
LDL-C (mg/dL) |
HDL-C (mg/dL) |
TG (mg/dL) |
Atkins |
-3.6 |
1.3 |
3.2 |
-32 |
Zone |
-3.8 |
-9.7 |
1.8 |
-54 |
Weight Watchers |
-3.5 |
-12.1 |
-0.2 |
-9.2 |
Ornish |
-3.6 |
-16.5 |
-3.6 |
-0.4 |
Summary
While diets can significantly affect lipid levels it should be recognized that the effect is typically relatively modest compared to drug therapy. Whether these modest effects on lipid levels can reduce the risk of CVD has not been tested in randomized controlled trials and given the difficulty of carrying out such long-term diet studies is likely not to be attempted. However, diet therapy can be initiated early in life and has the potential to result in long-term decreases in lipid levels. Given that studies have shown that long-term modest reductions in LDL-C levels can have major effects on the risk of CVD (a 10mg/dL life-long decrease in LDL-C due to polymorphisms in ATP citrate lyase, HMGCoA reductase, LDL receptor, PCSK9, and NPC1L1 resulted in a 16%-18% decrease in cardiovascular events (263)) it is likely that a similar long-term decrease induced by dietary changes would also be effective in decreasing CVD. A life-long 70mg/dL decrease in TG levels due to polymorphisms in the lipoprotein lipase gene resulted in a 23% decrease in coronary heart disease suggesting that long-term decreases in TG levels due to dietary changes would also be beneficial (264). Thus, long-term reductions in lipid levels induced by diet therapy may reduce the lifetime risk of developing CVD.
CURRENT DIETARY GUIDELINES
Most dietary guidelines recommended to the general population to prevent disease are very similar so I will only present the recommendations of two organizations. A brief summary of the Guidelines for Americans 2020-2025 is shown in table 20 and the guidelines from the American College of Cardiology/American Heart Association are shown in table 21.
Table 20. Guidelines for Americans 2020-2025 |
|
---|---|
Recommend |
Limit |
Vegetables of all types—dark green; red and orange; beans, peas, and lentils; starchy; and other vegetables |
Added sugars—Less than 10 percent of calories per day |
Fruits, especially whole fruit |
Saturated fat—Less than 10 percent of calories per day |
Grains, at least half of which are whole grain |
Sodium—Less than 2,300 milligrams per day |
Dairy, including fat-free or low-fat milk, yogurt, and cheese, and/or lactose-free versions and fortified soy beverages and yogurt as alternatives |
Alcoholic beverages—Adults can choose not to drink or to drink in moderation by limiting intake to 2 drinks or less in a day for men and 1 drink or less in a day for women |
Protein foods, including lean meats, poultry, and eggs; seafood; beans, peas, and lentils; and nuts, seeds, and soy products |
|
Oils, including vegetable oils and oils in food, such as seafood and nuts |
|
Full guideline is available at DietaryGuidelines.gov
Table 21. ACC/AHA Dietary Recommendations to Reduce Risk of ASCVD (265) |
---|
1. A diet emphasizing intake of vegetables, fruits, legumes, nuts, whole grains, and fish is recommended |
2. Replacement of saturated fat with dietary monounsaturated and polyunsaturated fats can be beneficial |
3. A diet containing reduced amounts of cholesterol and sodium can be beneficial |
4. As a part of a healthy diet, it is reasonable to minimize the intake of processed meats, refined carbohydrates, and sweetened beverages |
5. As a part of a healthy diet, the intake of trans fats should be avoided |
ASCVD- Atherosclerotic CVD
DIETARY RECOMMENDATIONS FOR PATIENTS WITH LIPID DISORDERS
Elevated LDL-C
The dietary approach to reduce LDL-C levels is to avoid TFA and decrease SFA and cholesterol intake while increasing intake of fiber and phytosterols (266). Additionally, weight loss if appropriate can be helpful in lowering LDL-C levels (266). Certain foods are effective in lowering LDL-C levels such as tree nuts or peanuts, plant protein from soy products, beans, peas, chickpeas, or lentils, and viscous soluble fiber from oats, barley, psyllium, eggplant, okra, apples, oranges, or berries and if possible, can be added to the individual’s diet (236,241). If one combines multiple nutritional changes one can have significant reductions in LDL-C levels (table 22).
Table 22. Effect of Multiple LDL-C Lowering Changes on LDL-C Levels |
|
---|---|
Nutritional Intervention |
Estimated LDL-C Decrease |
Replace 5% of energy from SFA with MUFA or PUFA |
5% to 10% |
7.5 grams/day viscous fiber |
6% to 9% |
2 grams/day plant sterols/stanols |
5% to 8% |
Replace 30 grams animal protein or CHO with plant protein |
3% to 5% |
Loss 5% body weight if excess adiposity |
3% to 5% |
Total Effect |
22% to 37% |
Table adapted from (267).
While diet alone usually does not reduce LDL-C sufficiently it adds to the beneficial effect of cholesterol lowering drugs. In a comparison of LDL-C lowering a low-fat diet alone lowered LDL-C by 5%, a statin alone by 27%, and the combination of low-fat diet plus statin by 32% demonstrating an independent and additive effect of combining diet and lipid lowering medications (268).
Modestly Elevated Triglycerides
The dietary approach to reduce TG levels is to reduce CHO intake particularly simple and refined sugars and to avoid or minimize alcohol intake (266). Weight loss if appropriate can be very helpful in lowering TG levels (25,266).
Markedly Elevated Triglycerides
In patients with marked elevations in TGs due to the Familial Chylomicronemia Syndrome a diet very low in fat is often necessary to prevent episodes of pancreatitis (<10% of calories from fat) (269). In patients with this disorder medium chain TGs may be helpful. In patients with the Multifactorial Chylomicronemia Syndrome who present with markedly elevated TGs (>1000mg/dL) initial dietary treatment should be a very low-fat diet until the TGs decrease. Once the TGs decrease one can initiate the diet described above for individuals with modestly elevated TGs.
Elevated Lipoprotein (a)
There is no evidence that healthy dietary changes significantly lower Lp(a) levels (62,270) . In fact, it should be noted that reducing SFA intake while decreasing LDL-C levels increases Lp(a) levels (271). In certain patients with high Lp(a) levels one may need to balance the benefits of decreasing LDL-C levels with the risks of increasing Lp(a) levels (271).
Effect of Dietary Advice on Lipid and Lipoprotein Levels
In a meta-analysis of 44 randomized studies with 18,175 healthy adult participants comparing dietary advise vs. no or minimal advice found that dietary advice reduced total serum cholesterol by 5.9mg/dL (95% CI 2.3 to 9.0) and LDL-C by 6.2mg/dL (95% CI 3.1 to 9.4) with no change in HDL-C and TG levels (272). In a meta-analysis of 7 studies with 1081 participants that compared consultation with a dietician vs. usual care there was no difference in the absolute change in TC, LDL-C, or HDL-C levels but TG levels were decreased by 19.4mg/dL (95%CI -37.8 to -1.8; p=0.03) (273). Similarly, in a meta-analysis of 5 randomized trials in 912 patients with type 2 diabetes found that dietary advice from a dietician vs. usual care resulted in a small decrease in LDL-C (6.6mg/dL) in the group receiving advice from the dietician (274). Finally, as discussed earlier the Women’s Health Initiative trial randomized 19,541 postmenopausal women 50-79 years of age to the diet intervention group and 29,294 women to usual dietary advice (52). The goal in the diet intervention group was to reduce total fat intake to 20% of calories and increase intake of vegetables/fruits to 5 servings/day and grains to at least 6 servings/day. Fat intake decreased by 8.2% of energy intake in the intervention vs the comparison group, with small decreases in SFA (2.9%), MUFA (3.3%), and PUFA (1.5%) with increased consumption of vegetables, fruits, and grains. LDL-C levels were reduced by 3.55 mg/dL in the intervention group while levels of HDL-C and TGs were not significantly different in the intervention vs comparison groups. Taken together these studies illustrate that diet therapy under many circumstances has only modest effects on lipid and lipoprotein levels. Of course, there are studies and individual patients where major reductions in lipid levels occur. For example, in a life style modification study including a vegetarian diet by Ornish and colleagues a marked decrease in LDL-C was observed (153mg/dL decreasing to 96mg/dL) (58). One is most likely to see dramatic effects the greater the change in diet (for example going from a typical Western diet to a vegetarian low-fat diet) and the higher the baseline lipid levels. Patient ability to follow the dietary advise is crucial for success.
ACKNOWLEDGEMENTS
This work was supported by grants from the Northern California Institute for Research and Education.
REFERENCES
- Ioannidis JPA. The Challenge of Reforming Nutritional Epidemiologic Research. JAMA 2018; 320:969-970
- Nissen SE. U.S. Dietary Guidelines: An Evidence-Free Zone. Ann Intern Med 2016; 164:558-559
- Lichtenstein AH. Nutrient supplements and cardiovascular disease: a heartbreaking story. J Lipid Res 2009; 50 Suppl:S429-433
- Rimm EB, Stampfer MJ, Ascherio A, Giovannucci E, Colditz GA, Willett WC. Vitamin E consumption and the risk of coronary heart disease in men. N Engl J Med 1993; 328:1450-1456
- Kushi LH, Folsom AR, Prineas RJ, Mink PJ, Wu Y, Bostick RM. Dietary antioxidant vitamins and death from coronary heart disease in postmenopausal women. N Engl J Med 1996; 334:1156-1162
- Stampfer MJ, Hennekens CH, Manson JE, Colditz GA, Rosner B, Willett WC. Vitamin E consumption and the risk of coronary disease in women. N Engl J Med 1993; 328:1444-1449
- Lee CH, Chan RSM, Wan HYL, Woo YC, Cheung CYY, Fong CHY, Cheung BMY, Lam TH, Janus E, Woo J, Lam KSL. Dietary Intake of Anti-Oxidant Vitamins A, C, and E Is Inversely Associated with Adverse Cardiovascular Outcomes in Chinese-A 22-Years Population-Based Prospective Study. Nutrients 2018; 10
- Knekt P, Reunanen A, Jarvinen R, Seppanen R, Heliovaara M, Aromaa A. Antioxidant vitamin intake and coronary mortality in a longitudinal population study. Am J Epidemiol 1994; 139:1180-1189
- Lee IM, Cook NR, Gaziano JM, Gordon D, Ridker PM, Manson JE, Hennekens CH, Buring JE. Vitamin E in the primary prevention of cardiovascular disease and cancer: the Women's Health Study: a randomized controlled trial. JAMA 2005; 294:56-65
- de Gaetano G. Low-dose aspirin and vitamin E in people at cardiovascular risk: a randomised trial in general practice. Collaborative Group of the Primary Prevention Project. Lancet 2001; 357:89-95
- Sesso HD, Buring JE, Christen WG, Kurth T, Belanger C, MacFadyen J, Bubes V, Manson JE, Glynn RJ, Gaziano JM. Vitamins E and C in the prevention of cardiovascular disease in men: the Physicians' Health Study II randomized controlled trial. JAMA 2008; 300:2123-2133
- Heart Protection Study Collaborative G. MRC/BHF Heart Protection Study of antioxidant vitamin supplementation in 20,536 high-risk individuals: a randomised placebo-controlled trial. Lancet 2002; 360:23-33
- Rimm EB, Willett WC, Hu FB, Sampson L, Colditz GA, Manson JE, Hennekens C, Stampfer MJ. Folate and vitamin B6 from diet and supplements in relation to risk of coronary heart disease among women. JAMA 1998; 279:359-364
- Voutilainen S, Rissanen TH, Virtanen J, Lakka TA, Salonen JT. Low dietary folate intake is associated with an excess incidence of acute coronary events: The Kuopio Ischemic Heart Disease Risk Factor Study. Circulation 2001; 103:2674-2680
- Jayedi A, Zargar MS. Intake of vitamin B6, folate, and vitamin B12 and risk of coronary heart disease: a systematic review and dose-response meta-analysis of prospective cohort studies. Crit Rev Food Sci Nutr 2019; 59:2697-2707
- Armitage JM, Bowman L, Clarke RJ, Wallendszus K, Bulbulia R, Rahimi K, Haynes R, Parish S, Sleight P, Peto R, Collins R. Effects of homocysteine-lowering with folic acid plus vitamin B12 vs placebo on mortality and major morbidity in myocardial infarction survivors: a randomized trial. JAMA 2010; 303:2486-2494
- Albert CM, Cook NR, Gaziano JM, Zaharris E, MacFadyen J, Danielson E, Buring JE, Manson JE. Effect of folic acid and B vitamins on risk of cardiovascular events and total mortality among women at high risk for cardiovascular disease: a randomized trial. JAMA 2008; 299:2027-2036
- Lonn E, Yusuf S, Arnold MJ, Sheridan P, Pogue J, Micks M, McQueen MJ, Probstfield J, Fodor G, Held C, Genest J, Jr. Homocysteine lowering with folic acid and B vitamins in vascular disease. N Engl J Med 2006; 354:1567-1577
- Marti-Carvajal AJ, Sola I, Lathyris D, Dayer M. Homocysteine-lowering interventions for preventing cardiovascular events. Cochrane Database Syst Rev 2017; 8:CD006612
- Zeraatkar D, Han MA, Guyatt GH, Vernooij RWM, El Dib R, Cheung K, Milio K, Zworth M, Bartoszko JJ, Valli C, Rabassa M, Lee Y, Zajac J, Prokop-Dorner A, Lo C, Bala MM, Alonso-Coello P, Hanna SE, Johnston BC. Red and Processed Meat Consumption and Risk for All-Cause Mortality and Cardiometabolic Outcomes: A Systematic Review and Meta-analysis of Cohort Studies. Ann Intern Med 2019; 171:703-710
- Qian F, Riddle MC, Wylie-Rosett J, Hu FB. Red and Processed Meats and Health Risks: How Strong Is the Evidence? Diabetes Care 2020; 43:265-271
- Ordovas JM, Lopez-Miranda J, Mata P, Perez-Jimenez F, Lichtenstein AH, Schaefer EJ. Gene-diet interaction in determining plasma lipid response to dietary intervention. Atherosclerosis 1995; 118 Suppl:S11-27
- Vazquez-Vidal I, Desmarchelier C, Jones PJH. Nutrigenetics of Blood Cholesterol Concentrations: Towards Personalized Nutrition. Curr Cardiol Rep 2019; 21:38
- Flock MR, Green MH, Kris-Etherton PM. Effects of adiposity on plasma lipid response to reductions in dietary saturated fatty acids and cholesterol. Adv Nutr 2011; 2:261-274
- Feingold KR. Obesity and Dyslipidemia. In: Feingold KR, Anawalt B, Boyce A, Chrousos G, de Herder WW, Dungan K, Grossman A, Hershman JM, Hofland J, Kaltsas G, Koch C, Kopp P, Korbonits M, McLachlan R, Morley JE, New M, Purnell J, Singer F, Stratakis CA, Trence DL, Wilson DP, eds. Endotext. South Dartmouth (MA) 2023.
- Kris-Etherton PM, Petersen K, Van Horn L. Convincing evidence supports reducing saturated fat to decrease cardiovascular disease risk. BMJ Nutr Prev Health 2018; 1:23-26
- Li Y, Hruby A, Bernstein AM, Ley SH, Wang DD, Chiuve SE, Sampson L, Rexrode KM, Rimm EB, Willett WC, Hu FB. Saturated Fats Compared With Unsaturated Fats and Sources of Carbohydrates in Relation to Risk of Coronary Heart Disease: A Prospective Cohort Study. J Am Coll Cardiol 2015; 66:1538-1548
- Lichtenstein AH. Dietary Fat and Cardiovascular Disease: Ebb and Flow Over the Last Half Century. Adv Nutr 2019; 10:S332-S339
- Jakobsen MU, O'Reilly EJ, Heitmann BL, Pereira MA, Balter K, Fraser GE, Goldbourt U, Hallmans G, Knekt P, Liu S, Pietinen P, Spiegelman D, Stevens J, Virtamo J, Willett WC, Ascherio A. Major types of dietary fat and risk of coronary heart disease: a pooled analysis of 11 cohort studies. Am J Clin Nutr 2009; 89:1425-1432
- Farvid MS, Ding M, Pan A, Sun Q, Chiuve SE, Steffen LM, Willett WC, Hu FB. Dietary linoleic acid and risk of coronary heart disease: a systematic review and meta-analysis of prospective cohort studies. Circulation 2014; 130:1568-1578
- Zheng Y, Fang Y, Xu X, Ye W, Kang S, Yang K, Cao Y, Xu R, Zheng J, Wang H. Dietary saturated fatty acids increased all-cause and cardiovascular disease mortality in an elderly population: The National Health and Nutrition Examination Survey. Nutr Res 2023; 120:99-114
- Siri-Tarino PW, Sun Q, Hu FB, Krauss RM. Meta-analysis of prospective cohort studies evaluating the association of saturated fat with cardiovascular disease. Am J Clin Nutr 2010; 91:535-546
- Chowdhury R, Warnakula S, Kunutsor S, Crowe F, Ward HA, Johnson L, Franco OH, Butterworth AS, Forouhi NG, Thompson SG, Khaw KT, Mozaffarian D, Danesh J, Di Angelantonio E. Association of dietary, circulating, and supplement fatty acids with coronary risk: a systematic review and meta-analysis. Ann Intern Med 2014; 160:398-406
- de Souza RJ, Mente A, Maroleanu A, Cozma AI, Ha V, Kishibe T, Uleryk E, Budylowski P, Schunemann H, Beyene J, Anand SS. Intake of saturated and trans unsaturated fatty acids and risk of all cause mortality, cardiovascular disease, and type 2 diabetes: systematic review and meta-analysis of observational studies. BMJ 2015; 351:h3978
- Skeaff CM, Miller J. Dietary fat and coronary heart disease: summary of evidence from prospective cohort and randomised controlled trials. Ann Nutr Metab 2009; 55:173-201
- Yamagishi K, Iso H, Yatsuya H, Tanabe N, Date C, Kikuchi S, Yamamoto A, Inaba Y, Tamakoshi A. Dietary intake of saturated fatty acids and mortality from cardiovascular disease in Japanese: the Japan Collaborative Cohort Study for Evaluation of Cancer Risk (JACC) Study. Am J Clin Nutr 2010; 92:759-765
- Clifton PM, Keogh JB. A systematic review of the effect of dietary saturated and polyunsaturated fat on heart disease. Nutr Metab Cardiovasc Dis 2017; 27:1060-1080
- Sacks FM, Lichtenstein AH, Wu JHY, Appel LJ, Creager MA, Kris-Etherton PM, Miller M, Rimm EB, Rudel LL, Robinson JG, Stone NJ, Van Horn LV. Dietary Fats and Cardiovascular Disease: A Presidential Advisory From the American Heart Association. Circulation 2017; 136:e1-e23
- Mozaffarian D, Micha R, Wallace S. Effects on coronary heart disease of increasing polyunsaturated fat in place of saturated fat: a systematic review and meta-analysis of randomized controlled trials. PLoS Med 2010; 7:e1000252
- de Oliveira Otto MC, Mozaffarian D, Kromhout D, Bertoni AG, Sibley CT, Jacobs DR, Jr., Nettleton JA. Dietary intake of saturated fat by food source and incident cardiovascular disease: the Multi-Ethnic Study of Atherosclerosis. Am J Clin Nutr 2012; 96:397-404
- Low-fat diet in myocardial infarction: A controlled trial. Lancet 1965; 2:501-504
- Leren P. The Oslo diet-heart study. Eleven-year report. Circulation 1970; 42:935-942
- Controlled trial of soya-bean oil in myocardial infarction. Lancet 1968; 2:693-699
- Dayton S, Pearce ML, Goldman H, Harnish A, Plotkin D, Shickman M, Winfield M, Zager A, Dixon W. Controlled trial of a diet high in unsaturated fat for prevention of atherosclerotic complications. Lancet 1968; 2:1060-1062
- DAYTON S PM, HASHIMOTO S, DIXON WJ, and TOMIYASU U. A Controlled Clinical Trial of a Diet High in Unsaturated Fat in Preventing Complications of Atherosclerosis. Circulation 1969; 40:II-1–II-63
- Turpeinen O, Karvonen MJ, Pekkarinen M, Miettinen M, Elosuo R, Paavilainen E. Dietary prevention of coronary heart disease: the Finnish Mental Hospital Study. Int J Epidemiol 1979; 8:99-118
- Miettinen M, Turpeinen O, Karvonen MJ, Pekkarinen M, Paavilainen E, Elosuo R. Dietary prevention of coronary heart disease in women: the Finnish mental hospital study. Int J Epidemiol 1983; 12:17-25
- Miettinen M, Turpeinen O, Karvonen MJ, Elosuo R, Paavilainen E. Effect of cholesterol-lowering diet on mortality from coronary heart-disease and other causes. A twelve-year clinical trial in men and women. Lancet 1972; 2:835-838
- Ramsden CE, Zamora D, Leelarthaepin B, Majchrzak-Hong SF, Faurot KR, Suchindran CM, Ringel A, Davis JM, Hibbeln JR. Use of dietary linoleic acid for secondary prevention of coronary heart disease and death: evaluation of recovered data from the Sydney Diet Heart Study and updated meta-analysis. BMJ 2013; 346:e8707
- Burr ML, Fehily AM, Gilbert JF, Rogers S, Holliday RM, Sweetnam PM, Elwood PC, Deadman NM. Effects of changes in fat, fish, and fibre intakes on death and myocardial reinfarction: diet and reinfarction trial (DART). Lancet 1989; 2:757-761
- Frantz ID, Jr., Dawson EA, Ashman PL, Gatewood LC, Bartsch GE, Kuba K, Brewer ER. Test of effect of lipid lowering by diet on cardiovascular risk. The Minnesota Coronary Survey. Arteriosclerosis 1989; 9:129-135
- Howard BV, Van Horn L, Hsia J, Manson JE, Stefanick ML, Wassertheil-Smoller S, Kuller LH, LaCroix AZ, Langer RD, Lasser NL, Lewis CE, Limacher MC, Margolis KL, Mysiw WJ, Ockene JK, Parker LM, Perri MG, Phillips L, Prentice RL, Robbins J, Rossouw JE, Sarto GE, Schatz IJ, Snetselaar LG, Stevens VJ, Tinker LF, Trevisan M, Vitolins MZ, Anderson GL, Assaf AR, Bassford T, Beresford SA, Black HR, Brunner RL, Brzyski RG, Caan B, Chlebowski RT, Gass M, Granek I, Greenland P, Hays J, Heber D, Heiss G, Hendrix SL, Hubbell FA, Johnson KC, Kotchen JM. Low-fat dietary pattern and risk of cardiovascular disease: the Women's Health Initiative Randomized Controlled Dietary Modification Trial. JAMA 2006; 295:655-666
- Wolfe MS, Sawyer JK, Morgan TM, Bullock BC, Rudel LL. Dietary polyunsaturated fat decreases coronary artery atherosclerosis in a pediatric-aged population of African green monkeys. Arterioscler Thromb 1994; 14:587-597
- Rudel LL, Parks JS, Sawyer JK. Compared with dietary monounsaturated and saturated fat, polyunsaturated fat protects African green monkeys from coronary artery atherosclerosis. Arterioscler Thromb Vasc Biol 1995; 15:2101-2110
- Feingold KR. Cholesterol Lowering Drugs. In: Feingold KR, Anawalt B, Boyce A, Chrousos G, de Herder WW, Dungan K, Grossman A, Hershman JM, Hofland J, Kaltsas G, Koch C, Kopp P, Korbonits M, McLachlan R, Morley JE, New M, Purnell J, Singer F, Stratakis CA, Trence DL, Wilson DP, eds. Endotext. South Dartmouth (MA) 2024.
- Silverman MG, Ference BA, Im K, Wiviott SD, Giugliano RP, Grundy SM, Braunwald E, Sabatine MS. Association Between Lowering LDL-C and Cardiovascular Risk Reduction Among Different Therapeutic Interventions: A Systematic Review and Meta-analysis. JAMA 2016; 316:1289-1297
- Watts GF, Lewis B, Brunt JN, Lewis ES, Coltart DJ, Smith LD, Mann JI, Swan AV. Effects on coronary artery disease of lipid-lowering diet, or diet plus cholestyramine, in the St Thomas' Atherosclerosis Regression Study (STARS). Lancet 1992; 339:563-569
- Ornish D, Brown SE, Scherwitz LW, Billings JH, Armstrong WT, Ports TA, McLanahan SM, Kirkeeide RL, Brand RJ, Gould KL. Can lifestyle changes reverse coronary heart disease? The Lifestyle Heart Trial. Lancet 1990; 336:129-133
- Feingold KR. Maximizing the benefits of cholesterol-lowering drugs. Curr Opin Lipidol 2019; 30:388-394
- Mensink RP. Effects of saturated fatty acids on serum lipids and lipoproteins: a systematic review and regression analysis. Geneva, Switzerland: Geneva: World Health Organization; 2016.
- Dreon DM, Fernstrom HA, Campos H, Blanche P, Williams PT, Krauss RM. Change in dietary saturated fat intake is correlated with change in mass of large low-density-lipoprotein particles in men. Am J Clin Nutr 1998; 67:828-836
- Enkhmaa B, Petersen KS, Kris-Etherton PM, Berglund L. Diet and Lp(a): Does Dietary Change Modify Residual Cardiovascular Risk Conferred by Lp(a)? Nutrients 2020; 12
- Mensink RP, Zock PL, Kester AD, Katan MB. Effects of dietary fatty acids and carbohydrates on the ratio of serum total to HDL cholesterol and on serum lipids and apolipoproteins: a meta-analysis of 60 controlled trials. Am J Clin Nutr 2003; 77:1146-1155
- Wu JHY, Micha R, Mozaffarian D. Dietary fats and cardiometabolic disease: mechanisms and effects on risk factors and outcomes. Nat Rev Cardiol 2019; 16:581-601
- Micha R, Mozaffarian D. Saturated fat and cardiometabolic risk factors, coronary heart disease, stroke, and diabetes: a fresh look at the evidence. Lipids 2010; 45:893-905
- Flock MR, Kris-Etherton PM. Diverse physiological effects of long-chain saturated fatty acids: implications for cardiovascular disease. Curr Opin Clin Nutr Metab Care 2013; 16:133-140
- Fernandez ML, West KL. Mechanisms by which dietary fatty acids modulate plasma lipids. J Nutr 2005; 135:2075-2078
- Dietschy JM. Dietary fatty acids and the regulation of plasma low density lipoprotein cholesterol concentrations. J Nutr 1998; 128:444S-448S
- Horton JD, Goldstein JL, Brown MS. SREBPs: activators of the complete program of cholesterol and fatty acid synthesis in the liver. J Clin Invest 2002; 109:1125-1131
- Mente A, de Koning L, Shannon HS, Anand SS. A systematic review of the evidence supporting a causal link between dietary factors and coronary heart disease. Arch Intern Med 2009; 169:659-669
- Schwingshackl L, Hoffmann G. Monounsaturated fatty acids, olive oil and health status: a systematic review and meta-analysis of cohort studies. Lipids Health Dis 2014; 13:154
- Zong G, Li Y, Sampson L, Dougherty LW, Willett WC, Wanders AJ, Alssema M, Zock PL, Hu FB, Sun Q. Monounsaturated fats from plant and animal sources in relation to risk of coronary heart disease among US men and women. Am J Clin Nutr 2018; 107:445-453
- Estruch R, Ros E, Salas-Salvado J, Covas MI, Corella D, Aros F, Gomez-Gracia E, Ruiz-Gutierrez V, Fiol M, Lapetra J, Lamuela-Raventos RM, Serra-Majem L, Pinto X, Basora J, Munoz MA, Sorli JV, Martinez JA, Martinez-Gonzalez MA. Primary prevention of cardiovascular disease with a Mediterranean diet. N Engl J Med 2013; 368:1279-1290
- Estruch R, Ros E, Salas-Salvado J, Covas MI, Corella D, Aros F, Gomez-Gracia E, Ruiz-Gutierrez V, Fiol M, Lapetra J, Lamuela-Raventos RM, Serra-Majem L, Pinto X, Basora J, Munoz MA, Sorli JV, Martinez JA, Fito M, Gea A, Hernan MA, Martinez-Gonzalez MA. Primary Prevention of Cardiovascular Disease with a Mediterranean Diet Supplemented with Extra-Virgin Olive Oil or Nuts. N Engl J Med 2018; 378:e34
- Estruch R, Ros E, Salas-Salvado J, Covas MI, Corella D, Aros F, Gomez-Gracia E, Ruiz-Gutierrez V, Fiol M, Lapetra J, Lamuela-Raventos RM, Serra-Majem L, Pinto X, Basora J, Munoz MA, Sorli JV, Martinez JA, Martinez-Gonzalez MA. Retraction and Republication: Primary Prevention of Cardiovascular Disease with a Mediterranean Diet. N Engl J Med 2013;368:1279-90. N Engl J Med 2018; 378:2441-2442
- Estruch R, Martinez-Gonzalez MA, Corella D, Salas-Salvado J, Ruiz-Gutierrez V, Covas MI, Fiol M, Gomez-Gracia E, Lopez-Sabater MC, Vinyoles E, Aros F, Conde M, Lahoz C, Lapetra J, Saez G, Ros E. Effects of a Mediterranean-style diet on cardiovascular risk factors: a randomized trial. Ann Intern Med 2006; 145:1-11
- de Lorgeril M, Renaud S, Mamelle N, Salen P, Martin JL, Monjaud I, Guidollet J, Touboul P, Delaye J. Mediterranean alpha-linolenic acid-rich diet in secondary prevention of coronary heart disease. Lancet 1994; 343:1454-1459
- de Lorgeril M, Salen P, Martin JL, Monjaud I, Delaye J, Mamelle N. Mediterranean diet, traditional risk factors, and the rate of cardiovascular complications after myocardial infarction: final report of the Lyon Diet Heart Study. Circulation 1999; 99:779-785
- Delgado-Lista J, Alcala-Diaz JF, Torres-Pena JD, Quintana-Navarro GM, Fuentes F, Garcia-Rios A, Ortiz-Morales AM, Gonzalez-Requero AI, Perez-Caballero AI, Yubero-Serrano EM, Rangel-Zuniga OA, Camargo A, Rodriguez-Cantalejo F, Lopez-Segura F, Badimon L, Ordovas JM, Perez-Jimenez F, Perez-Martinez P, Lopez-Miranda J. Long-term secondary prevention of cardiovascular disease with a Mediterranean diet and a low-fat diet (CORDIOPREV): a randomised controlled trial. Lancet 2022; 399:1876-1885
- Li J, Guasch-Ferre M, Li Y, Hu FB. Dietary intake and biomarkers of linoleic acid and mortality: systematic review and meta-analysis of prospective cohort studies. Am J Clin Nutr 2020; 112:150-167
- Marklund M, Wu JHY, Imamura F, Del Gobbo LC, Fretts A, de Goede J, Shi P, Tintle N, Wennberg M, Aslibekyan S, Chen TA, de Oliveira Otto MC, Hirakawa Y, Eriksen HH, Kroger J, Laguzzi F, Lankinen M, Murphy RA, Prem K, Samieri C, Virtanen J, Wood AC, Wong K, Yang WS, Zhou X, Baylin A, Boer JMA, Brouwer IA, Campos H, Chaves PHM, Chien KL, de Faire U, Djousse L, Eiriksdottir G, El-Abbadi N, Forouhi NG, Michael Gaziano J, Geleijnse JM, Gigante B, Giles G, Guallar E, Gudnason V, Harris T, Harris WS, Helmer C, Hellenius ML, Hodge A, Hu FB, Jacques PF, Jansson JH, Kalsbeek A, Khaw KT, Koh WP, Laakso M, Leander K, Lin HJ, Lind L, Luben R, Luo J, McKnight B, Mursu J, Ninomiya T, Overvad K, Psaty BM, Rimm E, Schulze MB, Siscovick D, Skjelbo Nielsen M, Smith AV, Steffen BT, Steffen L, Sun Q, Sundstrom J, Tsai MY, Tunstall-Pedoe H, Uusitupa MIJ, van Dam RM, Veenstra J, Monique Verschuren WM, Wareham N, Willett W, Woodward M, Yuan JM, Micha R, Lemaitre RN, Mozaffarian D, Riserus U. Biomarkers of Dietary Omega-6 Fatty Acids and Incident Cardiovascular Disease and Mortality. Circulation 2019; 139:2422-2436
- Zhu Y, Bo Y, Liu Y. Dietary total fat, fatty acids intake, and risk of cardiovascular disease: a dose-response meta-analysis of cohort studies. Lipids Health Dis 2019; 18:91
- Hooper L, Al-Khudairy L, Abdelhamid AS, Rees K, Brainard JS, Brown TJ, Ajabnoor SM, O'Brien AT, Winstanley LE, Donaldson DH, Song F, Deane KH. Omega-6 fats for the primary and secondary prevention of cardiovascular disease. Cochrane Database Syst Rev 2018; 11:CD011094
- Abdelhamid AS, Martin N, Bridges C, Brainard JS, Wang X, Brown TJ, Hanson S, Jimoh OF, Ajabnoor SM, Deane KH, Song F, Hooper L. Polyunsaturated fatty acids for the primary and secondary prevention of cardiovascular disease. Cochrane Database Syst Rev 2018; 11:CD012345
- Feingold KR. Triglyceride Lowering Drugs. In: Feingold KR, Anawalt B, Boyce A, Chrousos G, de Herder WW, Dungan K, Grossman A, Hershman JM, Hofland J, Kaltsas G, Koch C, Kopp P, Korbonits M, McLachlan R, Morley JE, New M, Purnell J, Singer F, Stratakis CA, Trence DL, Wilson DP, eds. Endotext. South Dartmouth (MA) 2024.
- Gardner CD, Kraemer HC. Monounsaturated versus polyunsaturated dietary fat and serum lipids. A meta-analysis. Arterioscler Thromb Vasc Biol 1995; 15:1917-1927
- Lichtenstein AH. Dietary trans fatty acids and cardiovascular disease risk: past and present. Curr Atheroscler Rep 2014; 16:433
- Mozaffarian D, Katan MB, Ascherio A, Stampfer MJ, Willett WC. Trans fatty acids and cardiovascular disease. N Engl J Med 2006; 354:1601-1613
- Nestel P. Trans fatty acids: are its cardiovascular risks fully appreciated? Clin Ther 2014; 36:315-321
- Mauger JF, Lichtenstein AH, Ausman LM, Jalbert SM, Jauhiainen M, Ehnholm C, Lamarche B. Effect of different forms of dietary hydrogenated fats on LDL particle size. Am J Clin Nutr 2003; 78:370-375
- Brouwer IA, Wanders AJ, Katan MB. Effect of animal and industrial trans fatty acids on HDL and LDL cholesterol levels in humans--a quantitative review. PLoS One 2010; 5:e9434
- Bendsen NT, Christensen R, Bartels EM, Astrup A. Consumption of industrial and ruminant trans fatty acids and risk of coronary heart disease: a systematic review and meta-analysis of cohort studies. Eur J Clin Nutr 2011; 65:773-783
- Matthan NR, Welty FK, Barrett PH, Harausz C, Dolnikowski GG, Parks JS, Eckel RH, Schaefer EJ, Lichtenstein AH. Dietary hydrogenated fat increases high-density lipoprotein apoA-I catabolism and decreases low-density lipoprotein apoB-100 catabolism in hypercholesterolemic women. Arterioscler Thromb Vasc Biol 2004; 24:1092-1097
- van Tol A, Zock PL, van Gent T, Scheek LM, Katan MB. Dietary trans fatty acids increase serum cholesterylester transfer protein activity in man. Atherosclerosis 1995; 115:129-134
- Soliman GA. Dietary Cholesterol and the Lack of Evidence in Cardiovascular Disease. Nutrients 2018; 10
- Xu Z, McClure ST, Appel LJ. Dietary Cholesterol Intake and Sources among U.S Adults: Results from National Health and Nutrition Examination Surveys (NHANES), 2001(-)2014. Nutrients 2018; 10
- Berger S, Raman G, Vishwanathan R, Jacques PF, Johnson EJ. Dietary cholesterol and cardiovascular disease: a systematic review and meta-analysis. Am J Clin Nutr 2015; 102:276-294
- Carson JAS, Lichtenstein AH, Anderson CAM, Appel LJ, Kris-Etherton PM, Meyer KA, Petersen K, Polonsky T, Van Horn L. Dietary Cholesterol and Cardiovascular Risk: A Science Advisory From the American Heart Association. Circulation 2020; 141:e39-e53
- Hu FB, Stampfer MJ, Manson JE, Rimm E, Colditz GA, Rosner BA, Hennekens CH, Willett WC. Dietary fat intake and the risk of coronary heart disease in women. N Engl J Med 1997; 337:1491-1499
- Ascherio A, Rimm EB, Giovannucci EL, Spiegelman D, Stampfer M, Willett WC. Dietary fat and risk of coronary heart disease in men: cohort follow up study in the United States. BMJ 1996; 313:84-90
- Alexander DD, Miller PE, Vargas AJ, Weed DL, Cohen SS. Meta-analysis of Egg Consumption and Risk of Coronary Heart Disease and Stroke. J Am Coll Nutr 2016; 35:704-716
- Krittanawong C, Narasimhan B, Wang Z, Virk HUH, Farrell AM, Zhang H, Tang WHW. Association Between Egg Consumption and Risk of Cardiovascular Outcomes: A Systematic Review and Meta-Analysis. Am J Med 2021; 134:76-83 e72
- Drouin-Chartier JP, Schwab AL, Chen S, Li Y, Sacks FM, Rosner B, Manson JE, Willett WC, Stampfer MJ, Hu FB, Bhupathiraju SN. Egg consumption and risk of type 2 diabetes: findings from 3 large US cohort studies of men and women and a systematic review and meta-analysis of prospective cohort studies. Am J Clin Nutr 2020; 112:619-630
- Mazidi M, Katsiki N, Mikhailidis DP, Pencina MJ, Banach M. Egg Consumption and Risk of Total and Cause-Specific Mortality: An Individual-Based Cohort Study and Pooling Prospective Studies on Behalf of the Lipid and Blood Pressure Meta-analysis Collaboration (LBPMC) Group. J Am Coll Nutr 2019; 38:552-563
- Xu L, Lam TH, Jiang CQ, Zhang WS, Zhu F, Jin YL, Woo J, Cheng KK, Thomas GN. Egg consumption and the risk of cardiovascular disease and all-cause mortality: Guangzhou Biobank Cohort Study and meta-analyses. Eur J Nutr 2019; 58:785-796
- Zhao B, Gan L, Graubard BI, Mannisto S, Albanes D, Huang J. Associations of Dietary Cholesterol, Serum Cholesterol, and Egg Consumption With Overall and Cause-Specific Mortality: Systematic Review and Updated Meta-Analysis. Circulation 2022; 145:1506-1520
- Vincent MJ, Allen B, Palacios OM, Haber LT, Maki KC. Meta-regression analysis of the effects of dietary cholesterol intake on LDL and HDL cholesterol. Am J Clin Nutr 2019; 109:7-16
- Herron KL, Lofgren IE, Sharman M, Volek JS, Fernandez ML. High intake of cholesterol results in less atherogenic low-density lipoprotein particles in men and women independent of response classification. Metabolism 2004; 53:823-830
- Djousse L, Gaziano JM. Dietary cholesterol and coronary artery disease: a systematic review. Curr Atheroscler Rep 2009; 11:418-422
- McNamara DJ. The impact of egg limitations on coronary heart disease risk: do the numbers add up? J Am Coll Nutr 2000; 19:540S-548S
- Grundy SM. Does Dietary Cholesterol Matter? Curr Atheroscler Rep 2016; 18:68
- Tannock LR, O'Brien KD, Knopp RH, Retzlaff B, Fish B, Wener MH, Kahn SE, Chait A. Cholesterol feeding increases C-reactive protein and serum amyloid A levels in lean insulin-sensitive subjects. Circulation 2005; 111:3058-3062
- Jakobsen MU, Dethlefsen C, Joensen AM, Stegger J, Tjonneland A, Schmidt EB, Overvad K. Intake of carbohydrates compared with intake of saturated fatty acids and risk of myocardial infarction: importance of the glycemic index. Am J Clin Nutr 2010; 91:1764-1768
- Lambadiari V, Korakas E, Tsimihodimos V. The Impact of Dietary Glycemic Index and Glycemic Load on Postprandial Lipid Kinetics, Dyslipidemia and Cardiovascular Risk. Nutrients 2020; 12
- Vega-Lopez S, Venn BJ, Slavin JL. Relevance of the Glycemic Index and Glycemic Load for Body Weight, Diabetes, and Cardiovascular Disease. Nutrients 2018; 10
- Jenkins DJA, Dehghan M, Mente A, Bangdiwala SI, Rangarajan S, Srichaikul K, Mohan V, Avezum A, Diaz R, Rosengren A, Lanas F, Lopez-Jaramillo P, Li W, Oguz A, Khatib R, Poirier P, Mohammadifard N, Pepe A, Alhabib KF, Chifamba J, Yusufali AH, Iqbal R, Yeates K, Yusoff K, Ismail N, Teo K, Swaminathan S, Liu X, Zatonska K, Yusuf R, Yusuf S. Glycemic Index, Glycemic Load, and Cardiovascular Disease and Mortality. N Engl J Med 2021; 384:1312-1322
- Jenkins DJA, Willett WC, Yusuf S, Hu FB, Glenn AJ, Liu S, Mente A, Miller V, Bangdiwala SI, Gerstein HC, Sieri S, Ferrari P, Patel AV, McCullough ML, Le Marchand L, Freedman ND, Loftfield E, Sinha R, Shu XO, Touvier M, Sawada N, Tsugane S, van den Brandt PA, Shuval K, Khan TA, Paquette M, Sahye-Pudaruth S, Patel D, Siu TFY, Srichaikul K, Kendall CWC, Sievenpiper JL. Association of glycaemic index and glycaemic load with type 2 diabetes, cardiovascular disease, cancer, and all-cause mortality: a meta-analysis of mega cohorts of more than 100 000 participants. Lancet Diabetes Endocrinol 2024; 12:107-118
- Yang Q, Zhang Z, Gregg EW, Flanders WD, Merritt R, Hu FB. Added sugar intake and cardiovascular diseases mortality among US adults. JAMA Intern Med 2014; 174:516-524
- Narain A, Kwok CS, Mamas MA. Soft drinks and sweetened beverages and the risk of cardiovascular disease and mortality: a systematic review and meta-analysis. Int J Clin Pract 2016; 70:791-805
- Ho FK, Gray SR, Welsh P, Petermann-Rocha F, Foster H, Waddell H, Anderson J, Lyall D, Sattar N, Gill JMR, Mathers JC, Pell JP, Celis-Morales C. Associations of fat and carbohydrate intake with cardiovascular disease and mortality: prospective cohort study of UK Biobank participants. BMJ 2020; 368:m688
- Malik VS, Li Y, Pan A, De Koning L, Schernhammer E, Willett WC, Hu FB. Long-Term Consumption of Sugar-Sweetened and Artificially Sweetened Beverages and Risk of Mortality in US Adults. Circulation 2019; 139:2113-2125
- de Koning L, Malik VS, Kellogg MD, Rimm EB, Willett WC, Hu FB. Sweetened beverage consumption, incident coronary heart disease, and biomarkers of risk in men. Circulation 2012; 125:1735-1741, S1731
- Gerber PA, Berneis K. Regulation of low-density lipoprotein subfractions by carbohydrates. Curr Opin Clin Nutr Metab Care 2012; 15:381-385
- Aeberli I, Gerber PA, Hochuli M, Kohler S, Haile SR, Gouni-Berthold I, Berthold HK, Spinas GA, Berneis K. Low to moderate sugar-sweetened beverage consumption impairs glucose and lipid metabolism and promotes inflammation in healthy young men: a randomized controlled trial. Am J Clin Nutr 2011; 94:479-485
- Fechner E, Smeets E, Schrauwen P, Mensink RP. The Effects of Different Degrees of Carbohydrate Restriction and Carbohydrate Replacement on Cardiometabolic Risk Markers in Humans-A Systematic Review and Meta-Analysis. Nutrients 2020; 12
- Mansoor N, Vinknes KJ, Veierod MB, Retterstol K. Effects of low-carbohydrate diets v. low-fat diets on body weight and cardiovascular risk factors: a meta-analysis of randomised controlled trials. Br J Nutr 2016; 115:466-479
- Hu T, Mills KT, Yao L, Demanelis K, Eloustaz M, Yancy WS, Jr., Kelly TN, He J, Bazzano LA. Effects of low-carbohydrate diets versus low-fat diets on metabolic risk factors: a meta-analysis of randomized controlled clinical trials. Am J Epidemiol 2012; 176 Suppl 7:S44-54
- Goff LM, Cowland DE, Hooper L, Frost GS. Low glycaemic index diets and blood lipids: a systematic review and meta-analysis of randomised controlled trials. Nutr Metab Cardiovasc Dis 2013; 23:1-10
- Schwingshackl L, Neuenschwander M, Hoffmann G, Buyken AE, Schlesinger S. Dietary sugars and cardiometabolic risk factors: a network meta-analysis on isocaloric substitution interventions. Am J Clin Nutr 2020; 111:187-196
- Te Morenga LA, Howatson AJ, Jones RM, Mann J. Dietary sugars and cardiometabolic risk: systematic review and meta-analyses of randomized controlled trials of the effects on blood pressure and lipids. Am J Clin Nutr 2014; 100:65-79
- Fattore E, Botta F, Agostoni C, Bosetti C. Effects of free sugars on blood pressure and lipids: a systematic review and meta-analysis of nutritional isoenergetic intervention trials. Am J Clin Nutr 2017; 105:42-56
- Livesey G, Taylor R. Fructose consumption and consequences for glycation, plasma triacylglycerol, and body weight: meta-analyses and meta-regression models of intervention studies. Am J Clin Nutr 2008; 88:1419-1437
- Stanhope KL, Bremer AA, Medici V, Nakajima K, Ito Y, Nakano T, Chen G, Fong TH, Lee V, Menorca RI, Keim NL, Havel PJ. Consumption of fructose and high fructose corn syrup increase postprandial triglycerides, LDL-cholesterol, and apolipoprotein-B in young men and women. J Clin Endocrinol Metab 2011; 96:E1596-1605
- Kroemer G, Lopez-Otin C, Madeo F, de Cabo R. Carbotoxicity-Noxious Effects of Carbohydrates. Cell 2018; 175:605-614
- Softic S, Meyer JG, Wang GX, Gupta MK, Batista TM, Lauritzen H, Fujisaka S, Serra D, Herrero L, Willoughby J, Fitzgerald K, Ilkayeva O, Newgard CB, Gibson BW, Schilling B, Cohen DE, Kahn CR. Dietary Sugars Alter Hepatic Fatty Acid Oxidation via Transcriptional and Post-translational Modifications of Mitochondrial Proteins. Cell Metab 2019; 30:735-753 e734
- Naghshi S, Sadeghi O, Willett WC, Esmaillzadeh A. Dietary intake of total, animal, and plant proteins and risk of all cause, cardiovascular, and cancer mortality: systematic review and dose-response meta-analysis of prospective cohort studies. BMJ 2020; 370:m2412
- Chen Z, Glisic M, Song M, Aliahmad HA, Zhang X, Moumdjian AC, Gonzalez-Jaramillo V, van der Schaft N, Bramer WM, Ikram MA, Voortman T. Dietary protein intake and all-cause and cause-specific mortality: results from the Rotterdam Study and a meta-analysis of prospective cohort studies. Eur J Epidemiol 2020; 35:411-429
- Budhathoki S, Sawada N, Iwasaki M, Yamaji T, Goto A, Kotemori A, Ishihara J, Takachi R, Charvat H, Mizoue T, Iso H, Tsugane S. Association of Animal and Plant Protein Intake With All-Cause and Cause-Specific Mortality in a Japanese Cohort. JAMA Intern Med 2019; 179:1509-1518
- Qi XX, Shen P. Associations of dietary protein intake with all-cause, cardiovascular disease, and cancer mortality: A systematic review and meta-analysis of cohort studies. Nutr Metab Cardiovasc Dis 2020; 30:1094-1105
- Schwingshackl L, Hoffmann G. Long-term effects of low-fat diets either low or high in protein on cardiovascular and metabolic risk factors: a systematic review and meta-analysis. Nutr J 2013; 12:48
- Wycherley TP, Moran LJ, Clifton PM, Noakes M, Brinkworth GD. Effects of energy-restricted high-protein, low-fat compared with standard-protein, low-fat diets: a meta-analysis of randomized controlled trials. Am J Clin Nutr 2012; 96:1281-1298
- Santesso N, Akl EA, Bianchi M, Mente A, Mustafa R, Heels-Ansdell D, Schunemann HJ. Effects of higher- versus lower-protein diets on health outcomes: a systematic review and meta-analysis. Eur J Clin Nutr 2012; 66:780-788
- Threapleton DE, Greenwood DC, Evans CE, Cleghorn CL, Nykjaer C, Woodhead C, Cade JE, Gale CP, Burley VJ. Dietary fibre intake and risk of cardiovascular disease: systematic review and meta-analysis. BMJ 2013; 347:f6879
- Pereira MA, O'Reilly E, Augustsson K, Fraser GE, Goldbourt U, Heitmann BL, Hallmans G, Knekt P, Liu S, Pietinen P, Spiegelman D, Stevens J, Virtamo J, Willett WC, Ascherio A. Dietary fiber and risk of coronary heart disease: a pooled analysis of cohort studies. Arch Intern Med 2004; 164:370-376
- Kim Y, Je Y. Dietary fibre intake and mortality from cardiovascular disease and all cancers: A meta-analysis of prospective cohort studies. Arch Cardiovasc Dis 2016; 109:39-54
- Reynolds A, Mann J, Cummings J, Winter N, Mete E, Te Morenga L. Carbohydrate quality and human health: a series of systematic reviews and meta-analyses. Lancet 2019; 393:434-445
- Liu L, Wang S, Liu J. Fiber consumption and all-cause, cardiovascular, and cancer mortalities: a systematic review and meta-analysis of cohort studies. Mol Nutr Food Res 2015; 59:139-146
- Wu Y, Qian Y, Pan Y, Li P, Yang J, Ye X, Xu G. Association between dietary fiber intake and risk of coronary heart disease: A meta-analysis. Clin Nutr 2015; 34:603-611
- Loveman E, Colquitt J, Rees K. Cochrane corner: does increasing intake of dietary fibre help prevent cardiovascular disease? Heart 2016; 102:1607-1609
- Hartley L, May MD, Loveman E, Colquitt JL, Rees K. Dietary fibre for the primary prevention of cardiovascular disease. Cochrane Database Syst Rev 2016:CD011472
- Hollaender PL, Ross AB, Kristensen M. Whole-grain and blood lipid changes in apparently healthy adults: a systematic review and meta-analysis of randomized controlled studies. Am J Clin Nutr 2015; 102:556-572
- Bell LP, Hectorne K, Reynolds H, Balm TK, Hunninghake DB. Cholesterol-lowering effects of psyllium hydrophilic mucilloid. Adjunct therapy to a prudent diet for patients with mild to moderate hypercholesterolemia. JAMA 1989; 261:3419-3423
- Anderson JW, Zettwoch N, Feldman T, Tietyen-Clark J, Oeltgen P, Bishop CW. Cholesterol-lowering effects of psyllium hydrophilic mucilloid for hypercholesterolemic men. Arch Intern Med 1988; 148:292-296
- Jovanovski E, Yashpal S, Komishon A, Zurbau A, Blanco Mejia S, Ho HVT, Li D, Sievenpiper J, Duvnjak L, Vuksan V. Effect of psyllium (Plantago ovata) fiber on LDL cholesterol and alternative lipid targets, non-HDL cholesterol and apolipoprotein B: a systematic review and meta-analysis of randomized controlled trials. Am J Clin Nutr 2018; 108:922-932
- Brown L, Rosner B, Willett WW, Sacks FM. Cholesterol-lowering effects of dietary fiber: a meta-analysis. Am J Clin Nutr 1999; 69:30-42
- Ghavami A, Ziaei R, Talebi S, Barghchi H, Nattagh-Eshtivani E, Moradi S, Rahbarinejad P, Mohammadi H, Ghasemi-Tehrani H, Marx W, Askari G. Soluble Fiber Supplementation and Serum Lipid Profile: A Systematic Review and Dose-Response Meta-Analysis of Randomized Controlled Trials. Adv Nutr 2023; 14:465-474
- Cohn JS, Kamili A, Wat E, Chung RW, Tandy S. Reduction in intestinal cholesterol absorption by various food components: mechanisms and implications. Atheroscler Suppl 2010; 11:45-48
- Jesch ED, Carr TP. Food Ingredients That Inhibit Cholesterol Absorption. Prev Nutr Food Sci 2017; 22:67-80
- Gunness P, Gidley MJ. Mechanisms underlying the cholesterol-lowering properties of soluble dietary fibre polysaccharides. Food Funct 2010; 1:149-155
- Piironeen V, Lampi A. Occurrence and levels of phytosterols in foods. New York Marcel Dekker, Inc.
- Ras RT, Geleijnse JM, Trautwein EA. LDL-cholesterol-lowering effect of plant sterols and stanols across different dose ranges: a meta-analysis of randomised controlled studies. Br J Nutr 2014; 112:214-219
- Demonty I, Ras RT, van der Knaap HC, Meijer L, Zock PL, Geleijnse JM, Trautwein EA. The effect of plant sterols on serum triglyceride concentrations is dependent on baseline concentrations: a pooled analysis of 12 randomised controlled trials. Eur J Nutr 2013; 52:153-160
- Calpe-Berdiel L, Escola-Gil JC, Blanco-Vaca F. New insights into the molecular actions of plant sterols and stanols in cholesterol metabolism. Atherosclerosis 2009; 203:18-31
- Micha R, Shulkin ML, Penalvo JL, Khatibzadeh S, Singh GM, Rao M, Fahimi S, Powles J, Mozaffarian D. Etiologic effects and optimal intakes of foods and nutrients for risk of cardiovascular diseases and diabetes: Systematic reviews and meta-analyses from the Nutrition and Chronic Diseases Expert Group (NutriCoDE). PLoS One 2017; 12:e0175149
- Bechthold A, Boeing H, Schwedhelm C, Hoffmann G, Knuppel S, Iqbal K, De Henauw S, Michels N, Devleesschauwer B, Schlesinger S, Schwingshackl L. Food groups and risk of coronary heart disease, stroke and heart failure: A systematic review and dose-response meta-analysis of prospective studies. Crit Rev Food Sci Nutr 2019; 59:1071-1090
- Vernooij RWM, Zeraatkar D, Han MA, El Dib R, Zworth M, Milio K, Sit D, Lee Y, Gomaa H, Valli C, Swierz MJ, Chang Y, Hanna SE, Brauer PM, Sievenpiper J, de Souza R, Alonso-Coello P, Bala MM, Guyatt GH, Johnston BC. Patterns of Red and Processed Meat Consumption and Risk for Cardiometabolic and Cancer Outcomes: A Systematic Review and Meta-analysis of Cohort Studies. Ann Intern Med 2019; 171:732-741
- Fraser GE, Sabate J, Beeson WL, Strahan TM. A possible protective effect of nut consumption on risk of coronary heart disease. The Adventist Health Study. Arch Intern Med 1992; 152:1416-1424
- Ros E. Nuts and novel biomarkers of cardiovascular disease. Am J Clin Nutr 2009; 89:1649S-1656S
- Ros E. Health benefits of nut consumption. Nutrients 2010; 2:652-682
- Sabate J, Haddad E, Tanzman JS, Jambazian P, Rajaram S. Serum lipid response to the graduated enrichment of a Step I diet with almonds: a randomized feeding trial. Am J Clin Nutr 2003; 77:1379-1384
- Sabate J, Oda K, Ros E. Nut consumption and blood lipid levels: a pooled analysis of 25 intervention trials. Arch Intern Med 2010; 170:821-827
- Sabate J, Wien M. Nuts, blood lipids and cardiovascular disease. Asia Pac J Clin Nutr 2010; 19:131-136
- Banel DK, Hu FB. Effects of walnut consumption on blood lipids and other cardiovascular risk factors: a meta-analysis and systematic review. Am J Clin Nutr 2009; 90:56-63
- Guasch-Ferre M, Li J, Hu FB, Salas-Salvado J, Tobias DK. Effects of walnut consumption on blood lipids and other cardiovascular risk factors: an updated meta-analysis and systematic review of controlled trials. Am J Clin Nutr 2018; 108:174-187
- Lee-Bravatti MA, Wang J, Avendano EE, King L, Johnson EJ, Raman G. Almond Consumption and Risk Factors for Cardiovascular Disease: A Systematic Review and Meta-analysis of Randomized Controlled Trials. Adv Nutr 2019; 10:1076-1088
- Hadi A, Asbaghi O, Kazemi M, Haghighian HK, Pantovic A, Ghaedi E, Abolhasani Zadeh F. Consumption of pistachio nuts positively affects lipid profiles: A systematic review and meta-analysis of randomized controlled trials. Crit Rev Food Sci Nutr 2023; 63:5358-5371
- Houston L, Probst YC, Chandra Singh M, Neale EP. Tree Nut and Peanut Consumption and Risk of Cardiovascular Disease: A Systematic Review and Meta-Analysis of Randomized Controlled Trials. Adv Nutr 2023; 14:1029-1049
- Pan A, Yu D, Demark-Wahnefried W, Franco OH, Lin X. Meta-analysis of the effects of flaxseed interventions on blood lipids. Am J Clin Nutr 2009; 90:288-297
- Hui S, Liu K, Lang H, Liu Y, Wang X, Zhu X, Doucette S, Yi L, Mi M. Comparative effects of different whole grains and brans on blood lipid: a network meta-analysis. Eur J Nutr 2019; 58:2779-2787
- Marshall S, Petocz P, Duve E, Abbott K, Cassettari T, Blumfield M, Fayet-Moore F. The Effect of Replacing Refined Grains with Whole Grains on Cardiovascular Risk Factors: A Systematic Review and Meta-Analysis of Randomized Controlled Trials with GRADE Clinical Recommendation. J Acad Nutr Diet 2020; 120:1859-1883 e1831
- Jenkins DJA, Blanco Mejia S, Chiavaroli L, Viguiliouk E, Li SS, Kendall CWC, Vuksan V, Sievenpiper JL. Cumulative Meta-Analysis of the Soy Effect Over Time. J Am Heart Assoc 2019; 8:e012458
- Moradi M, Daneshzad E, Azadbakht L. The effects of isolated soy protein, isolated soy isoflavones and soy protein containing isoflavones on serum lipids in postmenopausal women: A systematic review and meta-analysis. Crit Rev Food Sci Nutr 2020; 60:3414-3428
- Tokede OA, Onabanjo TA, Yansane A, Gaziano JM, Djousse L. Soya products and serum lipids: a meta-analysis of randomised controlled trials. Br J Nutr 2015; 114:831-843
- Anderson JW, Bush HM. Soy protein effects on serum lipoproteins: a quality assessment and meta-analysis of randomized, controlled studies. J Am Coll Nutr 2011; 30:79-91
- Anderson JW, Johnstone BM, Cook-Newell ME. Meta-analysis of the effects of soy protein intake on serum lipids. N Engl J Med 1995; 333:276-282
- Reynolds K, Chin A, Lees KA, Nguyen A, Bujnowski D, He J. A meta-analysis of the effect of soy protein supplementation on serum lipids. Am J Cardiol 2006; 98:633-640
- Zhan S, Ho SC. Meta-analysis of the effects of soy protein containing isoflavones on the lipid profile. Am J Clin Nutr 2005; 81:397-408
- Simental-Mendia LE, Gotto AM, Jr., Atkin SL, Banach M, Pirro M, Sahebkar A. Effect of soy isoflavone supplementation on plasma lipoprotein(a) concentrations: A meta-analysis. J Clin Lipidol 2018; 12:16-24
- Hunter PM, Hegele RA. Functional foods and dietary supplements for the management of dyslipidaemia. Nat Rev Endocrinol 2017; 13:278-288
- Jenkins DJ, Mirrahimi A, Srichaikul K, Berryman CE, Wang L, Carleton A, Abdulnour S, Sievenpiper JL, Kendall CW, Kris-Etherton PM. Soy protein reduces serum cholesterol by both intrinsic and food displacement mechanisms. J Nutr 2010; 140:2302S-2311S
- Ried K, Toben C, Fakler P. Effect of garlic on serum lipids: an updated meta-analysis. Nutr Rev 2013; 71:282-299
- Zeng T GF, Zhang C,Song F, Zhao X, Xi K. A meta‐analysis of randomized, double‐blind, placebo‐controlled trials for the effects of garlic on serum lipid profiles. Journal of the Science of Food and Agricultue 2012; 92:1892-1902
- Reinhart KM, Talati R, White CM, Coleman CI. The impact of garlic on lipid parameters: a systematic review and meta-analysis. Nutr Res Rev 2009; 22:39-48
- Stevinson C, Pittler MH, Ernst E. Garlic for treating hypercholesterolemia. A meta-analysis of randomized clinical trials. Ann Intern Med 2000; 133:420-429
- Kwak JS, Kim JY, Paek JE, Lee YJ, Kim HR, Park DS, Kwon O. Garlic powder intake and cardiovascular risk factors: a meta-analysis of randomized controlled clinical trials. Nutr Res Pract 2014; 8:644-654
- Schwingshackl L, Missbach B, Hoffmann G. An umbrella review of garlic intake and risk of cardiovascular disease. Phytomedicine 2016; 23:1127-1133
- Sun YE, Wang W, Qin J. Anti-hyperlipidemia of garlic by reducing the level of total cholesterol and low-density lipoprotein: A meta-analysis. Medicine (Baltimore) 2018; 97:e0255
- Li S, Guo W, Lau W, Zhang H, Zhan Z, Wang X, Wang H. The association of garlic intake and cardiovascular risk factors: A systematic review and meta-analysis. Crit Rev Food Sci Nutr 2023; 63:8013-8031
- Shabani E, Sayemiri K, Mohammadpour M. The effect of garlic on lipid profile and glucose parameters in diabetic patients: A systematic review and meta-analysis. Prim Care Diabetes 2019; 13:28-42
- Kitamura K, Okada Y, Okada K, Kawaguchi Y, Nagaoka S. Epigallocatechin gallate induces an up-regulation of LDL receptor accompanied by a reduction of PCSK9 via the annexin A2-independent pathway in HepG2 cells. Mol Nutr Food Res 2017; 61
- Cui CJ, Jin JL, Guo LN, Sun J, Wu NQ, Guo YL, Liu G, Dong Q, Li JJ. Beneficial impact of epigallocatechingallate on LDL-C through PCSK9/LDLR pathway by blocking HNF1alpha and activating FoxO3a. J Transl Med 2020; 18:195
- Koo SI, Noh SK. Green tea as inhibitor of the intestinal absorption of lipids: potential mechanism for its lipid-lowering effect. J Nutr Biochem 2007; 18:179-183
- Onakpoya I, Spencer E, Heneghan C, Thompson M. The effect of green tea on blood pressure and lipid profile: a systematic review and meta-analysis of randomized clinical trials. Nutr Metab Cardiovasc Dis 2014; 24:823-836
- Zheng XX, Xu YL, Li SH, Liu XX, Hui R, Huang XH. Green tea intake lowers fasting serum total and LDL cholesterol in adults: a meta-analysis of 14 randomized controlled trials. Am J Clin Nutr 2011; 94:601-610
- Hartley L, Flowers N, Holmes J, Clarke A, Stranges S, Hooper L, Rees K. Green and black tea for the primary prevention of cardiovascular disease. Cochrane Database Syst Rev 2013:CD009934
- Zhao Y, Asimi S, Wu K, Zheng J, Li D. Black tea consumption and serum cholesterol concentration: Systematic review and meta-analysis of randomized controlled trials. Clin Nutr 2015; 34:612-619
- Liu W, Wan C, Huang Y, Li M. Effects of tea consumption on metabolic syndrome: A systematic review and meta-analysis of randomized clinical trials. Phytother Res 2020; 34:2857-2866
- Yuan F, Dong H, Fang K, Gong J, Lu F. Effects of green tea on lipid metabolism in overweight or obese people: A meta-analysis of randomized controlled trials. Mol Nutr Food Res 2018; 62
- Igho-Osagie E, Cara K, Wang D, Yao Q, Penkert LP, Cassidy A, Ferruzzi M, Jacques PF, Johnson EJ, Chung M, Wallace T. Short-Term Tea Consumption Is Not Associated with a Reduction in Blood Lipids or Pressure: A Systematic Review and Meta-Analysis of Randomized Controlled Trials. J Nutr 2020; 150:3269-3279
- Momose Y, Maeda-Yamamoto M, Nabetani H. Systematic review of green tea epigallocatechin gallate in reducing low-density lipoprotein cholesterol levels of humans. Int J Food Sci Nutr 2016; 67:606-613
- Araya-Quintanilla F, Gutierrez-Espinoza H, Moyano-Galvez V, Munoz-Yanez MJ, Pavez L, Garcia K. Effectiveness of black tea versus placebo in subjects with hypercholesterolemia: A PRISMA systematic review and meta-analysis. Diabetes Metab Syndr 2019; 13:2250-2258
- Wang D, Chen C, Wang Y, Liu J, Lin R. Effect of black tea consumption on blood cholesterol: a meta-analysis of 15 randomized controlled trials. PLoS One 2014; 9:e107711
- Kim A, Chiu A, Barone MK, Avino D, Wang F, Coleman CI, Phung OJ. Green tea catechins decrease total and low-density lipoprotein cholesterol: a systematic review and meta-analysis. J Am Diet Assoc 2011; 111:1720-1729
- Xu R, Yang K, Li S, Dai M, Chen G. Effect of green tea consumption on blood lipids: a systematic review and meta-analysis of randomized controlled trials. Nutr J 2020; 19:48
- O'Keefe JH, Bhatti SK, Patil HR, DiNicolantonio JJ, Lucan SC, Lavie CJ. Effects of habitual coffee consumption on cardiometabolic disease, cardiovascular health, and all-cause mortality. J Am Coll Cardiol 2013; 62:1043-1051
- Urgert R, Katan MB. The cholesterol-raising factor from coffee beans. Annu Rev Nutr 1997; 17:305-324
- Jee SH, He J, Appel LJ, Whelton PK, Suh I, Klag MJ. Coffee consumption and serum lipids: a meta-analysis of randomized controlled clinical trials. Am J Epidemiol 2001; 153:353-362
- Latham LS, Hensen ZK, Minor DS. Chocolate--guilty pleasure or healthy supplement? J Clin Hypertens (Greenwich) 2014; 16:101-106
- Hooper L, Kay C, Abdelhamid A, Kroon PA, Cohn JS, Rimm EB, Cassidy A. Effects of chocolate, cocoa, and flavan-3-ols on cardiovascular health: a systematic review and meta-analysis of randomized trials. Am J Clin Nutr 2012; 95:740-751
- Lin X, Zhang I, Li A, Manson JE, Sesso HD, Wang L, Liu S. Cocoa Flavanol Intake and Biomarkers for Cardiometabolic Health: A Systematic Review and Meta-Analysis of Randomized Controlled Trials. J Nutr 2016; 146:2325-2333
- Shrime MG, Bauer SR, McDonald AC, Chowdhury NH, Coltart CE, Ding EL. Flavonoid-rich cocoa consumption affects multiple cardiovascular risk factors in a meta-analysis of short-term studies. J Nutr 2011; 141:1982-1988
- Tokede OA, Gaziano JM, Djousse L. Effects of cocoa products/dark chocolate on serum lipids: a meta-analysis. Eur J Clin Nutr 2011; 65:879-886
- Rimm EB, Williams P, Fosher K, Criqui M, Stampfer MJ. Moderate alcohol intake and lower risk of coronary heart disease: meta-analysis of effects on lipids and haemostatic factors. BMJ 1999; 319:1523-1528
- Brien SE, Ronksley PE, Turner BJ, Mukamal KJ, Ghali WA. Effect of alcohol consumption on biological markers associated with risk of coronary heart disease: systematic review and meta-analysis of interventional studies. BMJ 2011; 342:d636
- Spaggiari G, Cignarelli A, Sansone A, Baldi M, Santi D. To beer or not to beer: A meta-analysis of the effects of beer consumption on cardiovascular health. PLoS One 2020; 15:e0233619
- Brinton EA. Effects of ethanol intake on lipoproteins. Curr Atheroscler Rep 2012; 14:108-114
- Badia RR, Pradhan RV, Ayers CR, Chandra A, Rohatgi A. The Relationship of Alcohol Consumption and HDL Metabolism in the Multiethnic Dallas Heart Study. J Clin Lipidol 2023; 17:124-130
- Kovar J, Zemankova K. Moderate alcohol consumption and triglyceridemia. Physiol Res 2015; 64:S371-375
- Klop B, do Rego AT, Cabezas MC. Alcohol and plasma triglycerides. Curr Opin Lipidol 2013; 24:321-326
- Cesena FH, Coimbra SR, Andrade AC, da Luz PL. The relationship between body mass index and the variation in plasma levels of triglycerides after short-term red wine consumption. J Clin Lipidol 2011; 5:294-298
- Ginsberg H, Olefsky J, Farquhar JW, Reaven GM. Moderate ethanol ingestion and plasma triglyceride levels. A study in normal and hypertriglyceridemic persons. Ann Intern Med 1974; 80:143-149
- De Oliveira ESER, Foster D, McGee Harper M, Seidman CE, Smith JD, Breslow JL, Brinton EA. Alcohol consumption raises HDL cholesterol levels by increasing the transport rate of apolipoproteins A-I and A-II. Circulation 2000; 102:2347-2352
- You M, Arteel GE. Effect of ethanol on lipid metabolism. J Hepatol 2019; 70:237-248
- Sozio M, Crabb DW. Alcohol and lipid metabolism. Am J Physiol Endocrinol Metab 2008; 295:E10-16
- Rees K, Takeda A, Martin N, Ellis L, Wijesekara D, Vepa A, Das A, Hartley L, Stranges S. Mediterranean-style diet for the primary and secondary prevention of cardiovascular disease. Cochrane Database Syst Rev 2019; 3:CD009825
- Ge L, Sadeghirad B, Ball GDC, da Costa BR, Hitchcock CL, Svendrovski A, Kiflen R, Quadri K, Kwon HY, Karamouzian M, Adams-Webber T, Ahmed W, Damanhoury S, Zeraatkar D, Nikolakopoulou A, Tsuyuki RT, Tian J, Yang K, Guyatt GH, Johnston BC. Comparison of dietary macronutrient patterns of 14 popular named dietary programmes for weight and cardiovascular risk factor reduction in adults: systematic review and network meta-analysis of randomised trials. BMJ 2020; 369:m696
- Harsha DW, Sacks FM, Obarzanek E, Svetkey LP, Lin PH, Bray GA, Aickin M, Conlin PR, Miller ER, 3rd, Appel LJ. Effect of dietary sodium intake on blood lipids: results from the DASH-sodium trial. Hypertension 2004; 43:393-398
- Siervo M, Lara J, Chowdhury S, Ashor A, Oggioni C, Mathers JC. Effects of the Dietary Approach to Stop Hypertension (DASH) diet on cardiovascular risk factors: a systematic review and meta-analysis. Br J Nutr 2015; 113:1-15
- Chiavaroli L, Viguiliouk E, Nishi SK, Blanco Mejia S, Rahelic D, Kahleova H, Salas-Salvado J, Kendall CW, Sievenpiper JL. DASH Dietary Pattern and Cardiometabolic Outcomes: An Umbrella Review of Systematic Reviews and Meta-Analyses. Nutrients 2019; 11
- Lari A, Sohouli MH, Fatahi S, Cerqueira HS, Santos HO, Pourrajab B, Rezaei M, Saneie S, Rahideh ST. The effects of the Dietary Approaches to Stop Hypertension (DASH) diet on metabolic risk factors in patients with chronic disease: A systematic review and meta-analysis of randomized controlled trials. Nutr Metab Cardiovasc Dis 2021; 31:2766-2778
- Chiavaroli L, Nishi SK, Khan TA, Braunstein CR, Glenn AJ, Mejia SB, Rahelic D, Kahleova H, Salas-Salvado J, Jenkins DJA, Kendall CWC, Sievenpiper JL. Portfolio Dietary Pattern and Cardiovascular Disease: A Systematic Review and Meta-analysis of Controlled Trials. Prog Cardiovasc Dis 2018; 61:43-53
- Ramezani-Jolfaie N, Mohammadi M, Salehi-Abargouei A. The effect of healthy Nordic diet on cardio-metabolic markers: a systematic review and meta-analysis of randomized controlled clinical trials. Eur J Nutr 2019; 58:2159-2174
- Massara P, Zurbau A, Glenn AJ, Chiavaroli L, Khan TA, Viguiliouk E, Mejia SB, Comelli EM, Chen V, Schwab U, Riserus U, Uusitupa M, Aas AM, Hermansen K, Thorsdottir I, Rahelic D, Kahleova H, Salas-Salvado J, Kendall CWC, Sievenpiper JL. Nordic dietary patterns and cardiometabolic outcomes: a systematic review and meta-analysis of prospective cohort studies and randomised controlled trials. Diabetologia 2022; 65:2011-2031
- Kirkpatrick CF, Bolick JP, Kris-Etherton PM, Sikand G, Aspry KE, Soffer DE, Willard KE, Maki KC. Review of current evidence and clinical recommendations on the effects of low-carbohydrate and very-low-carbohydrate (including ketogenic) diets for the management of body weight and other cardiometabolic risk factors: A scientific statement from the National Lipid Association Nutrition and Lifestyle Task Force. J Clin Lipidol 2019; 13:689-711 e681
- Kwiterovich PO, Jr., Vining EP, Pyzik P, Skolasky R, Jr., Freeman JM. Effect of a high-fat ketogenic diet on plasma levels of lipids, lipoproteins, and apolipoproteins in children. JAMA 2003; 290:912-920
- Buga A, Welton GL, Scott KE, Atwell AD, Haley SJ, Esbenshade NJ, Abraham J, Buxton JD, Ault DL, Raabe AS, Noakes TD, Hyde PN, Volek JS, Prins PJ. The Effects of Carbohydrate versus Fat Restriction on Lipid Profiles in Highly Trained, Recreational Distance Runners: A Randomized, Cross-Over Trial. Nutrients 2022; 14
- Buren J, Ericsson M, Damasceno NRT, Sjodin A. A Ketogenic Low-Carbohydrate High-Fat Diet Increases LDL Cholesterol in Healthy, Young, Normal-Weight Women: A Randomized Controlled Feeding Trial. Nutrients 2021; 13
- Creighton BC, Hyde PN, Maresh CM, Kraemer WJ, Phinney SD, Volek JS. Paradox of hypercholesterolaemia in highly trained, keto-adapted athletes. BMJ Open Sport Exerc Med 2018; 4:e000429
- Retterstol K, Svendsen M, Narverud I, Holven KB. Effect of low carbohydrate high fat diet on LDL cholesterol and gene expression in normal-weight, young adults: A randomized controlled study. Atherosclerosis 2018; 279:52-61
- Lima PA, de Brito Sampaio LP, Damasceno NR. Ketogenic diet in epileptic children: impact on lipoproteins and oxidative stress. Nutr Neurosci 2015; 18:337-344
- Joo M, Moon S, Lee YS, Kim MG. Effects of very low-carbohydrate ketogenic diets on lipid profiles in normal-weight (body mass index < 25 kg/m2) adults: a meta-analysis. Nutr Rev 2023; 81:1393-1401
- Castellana M, Conte E, Cignarelli A, Perrini S, Giustina A, Giovanella L, Giorgino F, Trimboli P. Efficacy and safety of very low calorie ketogenic diet (VLCKD) in patients with overweight and obesity: A systematic review and meta-analysis. Rev Endocr Metab Disord 2020; 21:5-16
- Norwitz NG, Feldman D, Soto-Mota A, Kalayjian T, Ludwig DS. Elevated LDL Cholesterol with a Carbohydrate-Restricted Diet: Evidence for a "Lean Mass Hyper-Responder" Phenotype. Curr Dev Nutr 2022; 6:nzab144
- Houttu V, Grefhorst A, Cohn DM, Levels JHM, Roeters van Lennep J, Stroes ESG, Groen AK, Tromp TR. Severe Dyslipidemia Mimicking Familial Hypercholesterolemia Induced by High-Fat, Low-Carbohydrate Diets: A Critical Review. Nutrients 2023; 15
- Norwitz NG, Mindrum MR, Giral P, Kontush A, Soto-Mota A, Wood TR, D'Agostino DP, Manubolu VS, Budoff M, Krauss RM. Elevated LDL-cholesterol levels among lean mass hyper-responders on low-carbohydrate ketogenic diets deserve urgent clinical attention and further research. J Clin Lipidol 2022; 16:765-768
- Goldberg IJ, Ibrahim N, Bredefeld C, Foo S, Lim V, Gutman D, Huggins LA, Hegele RA. Ketogenic diets, not for everyone. J Clin Lipidol 2021; 15:61-67
- Schaffer AE, D'Alessio DA, Guyton JR. Extreme elevations of low-density lipoprotein cholesterol with very low carbohydrate, high fat diets. J Clin Lipidol 2021; 15:525-526
- Schmidt T, Harmon DM, Kludtke E, Mickow A, Simha V, Kopecky S. Dramatic elevation of LDL cholesterol from ketogenic-dieting: A Case Series. Am J Prev Cardiol 2023; 14:100495
- Foster GD, Wyatt HR, Hill JO, Makris AP, Rosenbaum DL, Brill C, Stein RI, Mohammed BS, Miller B, Rader DJ, Zemel B, Wadden TA, Tenhave T, Newcomb CW, Klein S. Weight and metabolic outcomes after 2 years on a low-carbohydrate versus low-fat diet: a randomized trial. Ann Intern Med 2010; 153:147-157
- Yokoyama Y, Levin SM, Barnard ND. Association between plant-based diets and plasma lipids: a systematic review and meta-analysis. Nutr Rev 2017; 75:683-698
- Wang F, Zheng J, Yang B, Jiang J, Fu Y, Li D. Effects of Vegetarian Diets on Blood Lipids: A Systematic Review and Meta-Analysis of Randomized Controlled Trials. J Am Heart Assoc 2015; 4:e002408
- Dansinger ML, Gleason JA, Griffith JL, Selker HP, Schaefer EJ. Comparison of the Atkins, Ornish, Weight Watchers, and Zone diets for weight loss and heart disease risk reduction: a randomized trial. JAMA 2005; 293:43-53
- Ference BA, Ray KK, Catapano AL, Ference TB, Burgess S, Neff DR, Oliver-Williams C, Wood AM, Butterworth AS, Di Angelantonio E, Danesh J, Kastelein JJP, Nicholls SJ. Mendelian Randomization Study of ACLY and Cardiovascular Disease. N Engl J Med 2019; 380:1033-1042
- Ference BA, Kastelein JJP, Ray KK, Ginsberg HN, Chapman MJ, Packard CJ, Laufs U, Oliver-Williams C, Wood AM, Butterworth AS, Di Angelantonio E, Danesh J, Nicholls SJ, Bhatt DL, Sabatine MS, Catapano AL. Association of Triglyceride-Lowering LPL Variants and LDL-C-Lowering LDLR Variants With Risk of Coronary Heart Disease. JAMA 2019; 321:364-373
- Arnett DK, Blumenthal RS, Albert MA, Buroker AB, Goldberger ZD, Hahn EJ, Himmelfarb CD, Khera A, Lloyd-Jones D, McEvoy JW, Michos ED, Miedema MD, Munoz D, Smith SC, Jr., Virani SS, Williams KA, Sr., Yeboah J, Ziaeian B. 2019 ACC/AHA Guideline on the Primary Prevention of Cardiovascular Disease: A Report of the American College of Cardiology/American Heart Association Task Force on Clinical Practice Guidelines. Circulation 2019; 140:e596-e646
- Mach F, Baigent C, Catapano AL, Koskinas KC, Casula M, Badimon L, Chapman MJ, De Backer GG, Delgado V, Ference BA, Graham IM, Halliday A, Landmesser U, Mihaylova B, Pedersen TR, Riccardi G, Richter DJ, Sabatine MS, Taskinen MR, Tokgozoglu L, Wiklund O, Group ESCSD. 2019 ESC/EAS Guidelines for the management of dyslipidaemias: lipid modification to reduce cardiovascular risk. Eur Heart J 2020; 41:111-188
- Kirkpatrick CF, Sikand G, Petersen KS, Anderson CAM, Aspry KE, Bolick JP, Kris-Etherton PM, Maki KC. Nutrition interventions for adults with dyslipidemia: A Clinical Perspective from the National Lipid Association. J Clin Lipidol 2023; 17:428-451
- Hunninghake DB, Stein EA, Dujovne CA, Harris WS, Feldman EB, Miller VT, Tobert JA, Laskarzewski PM, Quiter E, Held J, et al. The efficacy of intensive dietary therapy alone or combined with lovastatin in outpatients with hypercholesterolemia. N Engl J Med 1993; 328:1213-1219
- Subramanian S. Hypertriglyceridemia: Pathophysiology, Role of Genetics, Consequences, and Treatment. In: Feingold KR, Anawalt B, Boyce A, Chrousos G, de Herder WW, Dungan K, Grossman A, Hershman JM, Hofland J, Kaltsas G, Koch C, Kopp P, Korbonits M, McLachlan R, Morley JE, New M, Purnell J, Singer F, Stratakis CA, Trence DL, Wilson DP, eds. Endotext. South Dartmouth (MA) 2024.
- Enkhmaa B, Anuurad E, Berglund L. Lipoprotein (a): impact by ethnicity and environmental and medical conditions. J Lipid Res 2016; 57:1111-1125
- Law HG, Meyers FJ, Berglund L, Enkhmaa B. Lipoprotein(a) and diet-a challenge for a role of saturated fat in cardiovascular disease risk reduction? Am J Clin Nutr 2023; 118:23-26
- Rees K, Dyakova M, Ward K, Thorogood M, Brunner E. Dietary advice for reducing cardiovascular risk. Cochrane Database Syst Rev 2013:CD002128
- Ross LJ, Barnes KA, Ball LE, Mitchell LJ, Sladdin I, Lee P, Williams LT. Effectiveness of dietetic consultation for lowering blood lipid levels in the management of cardiovascular disease risk: A systematic review and meta-analysis of randomised controlled trials. Nutr Diet 2019; 76:199-210
- Moller G, Andersen HK, Snorgaard O. A systematic review and meta-analysis of nutrition therapy compared with dietary advice in patients with type 2 diabetes. Am J Clin Nutr 2017; 106:1394-1400