ABSTRACT
Graves' disease includes thyrotoxicosis, goiter, exophthalmos, and pretibial myxedema when fully expressed, but can occur with one or more of these features.
Graves' disease is a disease of "autoimmunity", but the final cause of autoimmunity remains unclear. A strong hereditary tendency is present. Inheritance of HLA antigens DR3, DQ 2, and DQA1*0501 predispose to Graves' disease. The abnormal immune response is characterized by the presence of antibodies directed against thyroid tissue antigens, including antibodies that react with the thyrotrophin receptor by binding to the receptor. Some of these antibodies act as agonists and stimulate the thyroid. The best-known of the antibodies is the serum factor first reported as "LATS", now known as "TSAb".It has been reported in active Graves' disease that T- lymphocyte suppressor cell function is diminished and suppressor cell number is reduced. It is hypothesized that an abnormality in the control of autoimmune responses is present in this disease and leads to production of high levels of autoantibodies that may stimulate the thyroid or eventually cause thyroid damage and cell death.
The thyroid gland is hyperfunctioning in Graves' disease. The pituitary response to TRH is also suppressed. The gland is unusually responsive to small doses of iodide, which both block further hormone synthesis and inhibit release of hormone from the gland.
The incidence of Graves' disease is reported in recent studies to be 1 to 2 cases per 1,000 population per year in England. This rate is considerably higher than the rate of about 0.3 cases per 1,000 previously reported from this country. The frequency in women is much greater than it is in men.
The classic features of thyrotoxicosis are nervousness, diminished sleep, tremulousness, tachycardia, increased appetite, weight loss, and increased perspiration and signs are goiter, occasionally with exophthalmos, and rarely with pretibial myxedema. Physical findings include fine skin and hair, tremulousness, a hyperactive heart, Plummer's nails, muscle weakness, accelerated reflex relaxation, occasional splenomegaly, and often peripheral edema. Autoimmune vitiligo or hives may coexist in patients with Graves' disease.
The disease typically begins gradually in adult women and is progressive unless treated. Thyrotoxicosis can cause congestive heart failure. Mitral valve prolapse, atrial tachycardia and fibrillation are commonly caused by thyrotoxicosis.. Amenorrhea or anovulatory cycling is common in women, and fertility is reduced.Thyrotoxicosis in untreated cases leads to cardiovascular damage, bone loss and fractures, or inanition, and can be fatal. The long-term history also includes spontaneous remission in some cases and eventual spontaneous development of hypothyroidism, since autoimmune thyroiditis coexists and destroys the thyroid gland.
DEFINITIONS
Hyperthyroidism was first described in the English language by Caleb Perry (1755-1822), but it is the description by the Irish physician Robert Graves [1], to whom credit is usually attributed. The eponym Basedow's disease is often used on the European continent to recognize the description by Karl A. von Basedow (1799 - 1854).
GRAVES' DISEASE AS A DISEASE OF THYROID AUTOIMMUNITY
Graves' disease, Hashimoto's thyroiditis, and idiopathic thyroid failure are closely associated and in fact overlapping syndromes. Hashimoto's thyroiditis is typically characterized by thyroid enlargement and often underactivity. Idiopathic hypothyroidism is usually the result of Hashimoto's thyroiditis, and myxedema is the most advanced form of this illness. Of course hypothyroidism and myxedema can also be induced by other causes of thyroid hormone deficiency. These three syndromes of autoimmune thyroid disease (AITD) share immunological abnormalities, histological changes in the thyroid, and genetic predisposition. Patients can move from one or the other category, depending upon the stage of their illness. For example, an individual might first be observed with thyroid enlargement and positive antibody tests for anti-thyroglobulin or anti-TPO antibodies, and thus qualify as having Hashimoto's thyroiditis. At a later stage, this individual might become hyperthyroid and fit in the category of Graves' disease. Or, the patient with hyperthyroidism might have progressive destruction of the thyroid, or develope blocking antibodies, and become hypothyroid or ultimately develop myxedema.
The common features of the autoimmune thyroid diseases include the immune reactivity to specific thyroid antigens. We now know that patients with AITD have immune reactivity, both antibodies and cell-mediated immunity, directed to the TSH receptor, thyroid peroxidase (TPO), and thyroglobulin (TG) [2]. Antibodies also exist to megalin (the thyroid cell TG receptor)(3), to the thyroidal iodide symporter [4], and antibodies reacting to components of eye muscle and fibroblasts are present in sera of patients with Graves’ ophthalmopathy [5]. ( Table 10-1) The immune reactivity includes development of antibodies to these antigens, cell-mediated immune responses due to lymphocyte reactivity, and development of circulating antigen/antibody complexes [6], at least for some of the antigens. Patients with AITD also often develop other "organ specific" antibodies, including antibodies directed to gastric parietal cells in 50% of patients with Hashimoto’s thyroiditis [7]. Jenkins and Weetman have recently reviewed the evidence indicating an association of AITD with ACTH deficiency, Addison's disease, chronic hepatitis, celiac disease, DM-1, multiple sclerosis, myasthenia, PA, premature ovarian failure, primary biliary cirrhosis, vitiligo, RA, SLE, systemic sclerosis, urticaria, and angioedema(8). Patients with AITD may have antibodies, less frequently, to adrenal steroidogenic enzymes, ovarian steroidogenic enzymes, and components of the pituitary gland, thus qualifying for the Multiple Endocrine Autoimmune Syndrome [9]. In addition, up to 25% of patients with active Graves' disease have low level titers of antibodies to DNA, and occasionally have antibodies to liver mitochondria [10, 11]. Further evidence of ongoing autoimmunity in Graves' disease is the elevation of ICAM-1, and IL-6 and IL-8 cytokines seen in hyperthyroid patients [12, 13]. Anti-cardiolipin antibodies are present in increased incidence in patients with autoimmune thyroid disease, including Graves’ disease. However, these are not necessarily pathogenic and may be nonspecific markers of immune disregulation [14].
Table 1. Antibodies in Graves' Disease |
Elevated levels of TSAb, TBII, and (rarely) TSBAb • Elevated levels of anti-TPOAb ( 80%) • Elevated levels of anti-TGAb ( 50%) • Antibodies reacting to the Iodide Symporter and Pendrin protein • Antibodies recognizing components of eye muscle and/or fibroblasts • Antibodies to DNA • Antibodies to Parietal Cells (infrequent) • Antibodies binding to platelets |
Graves' disease is associated statistically with a group of autoimmune diseases including pernicious anemia, vitiligo [15], alopecia [16], angioedema [17], myasthenia gravis [18], and idiopathic thrombocytopenic purpura [13]. A weak association is probably present with rheumatoid arthritis and systemic lupus erythematosus [19]. Graves' disease is an example of an organ specific autoimmune disease, and appears not to be statistically more common among individuals who have, dermatomyositis, or scleroderma [20].
Up to 90% of patients with Graves' disease have antibodies directed to the "microsomal antigen" in the thyroid, known now to be thyroid peroxidase [21-25]. A lower proportion, approximately 50%, have antibodies directed against thyroglobulin [25]. Rarely patients have antibodies directed against T4 or T3 [23]. These antibodies are very similar to those present in Hashimoto's thyroiditis and idiopathic myxedema. Peripheral blood mononuclear cells [26], thyroid lymphocytes [27], and lymph node lymphocytes demonstrated cell-mediated immunity to TG, TPO, and TSH-R [28,29], and also to specific peptide epitopes of TSH-R,TPO and TG [30-35]. The functional consequence of having TG antibodies is uncertain, but they do not appear in general to cause thyroid cell destruction. TG/anti-TG immune complexes are rarely deposited in the kidney basement membrane of the glomeruli and can, in extremely rare circumstances, produce disease [36-38]. Anti-TPO antibodies are not known to play a role in Graves' disease, although they are thought possibly to be cytotoxic and function in the pathology of Hashimoto's thyroiditis [38]. Presumably TPO antibodies could similarly cause cytotoxicity in Graves' disease. About 1/3 of patients with autoimmune thyroid disease have ANA antibodies, and the ANA+ patients may also have antiRo, anti-La, anti-dsDNA , and anti-cardiolipin antibodies, and up to 10% have Sjogren's Syndrome.(27.1).
TSH-Receptor Antibodies
The antibodies of central importance in Graves' disease are those directed against the TSH receptor on the thyroid cell membrane. Protein factors in the circulation, thought to play a role in Graves' disease, have been described for more than five decades. Serum factors were described which produce exophthalmos in experimental models including fish and guinea pigs, and were given the eponym Exophthalmos Producing Substance [40,41]. For a time this material was thought to be a modified TSH molecule.
The first clear evidence of a circulating factor that could induce thyroid hyperactivity came from studies by Adams and Purves , who showed the presence of a factor in human serum that could stimulate the thyroid of guinea pigs [42]. Ultimately they found that infusion of this material into a human stimulated release of hormone from the thyroid [43](Figure 10-1). Because of the time course of its action being longer than TSH, this material was labelled Long Acting Thyroid Stimulator, or LATS. It was subsequently shown to be a gammaglobulin, and thus began the entirely new concept of an autoimmune disease due to stimulation of the thyroid by an antibody to an antigen in the thyroid, that mimicked the action of the natural stimulator, TSH. Nearly three decades later, the antigen to which this antibody was directed was identified as the thyroid cell surface protein receptor for TSH (TSH-R) [44,45].
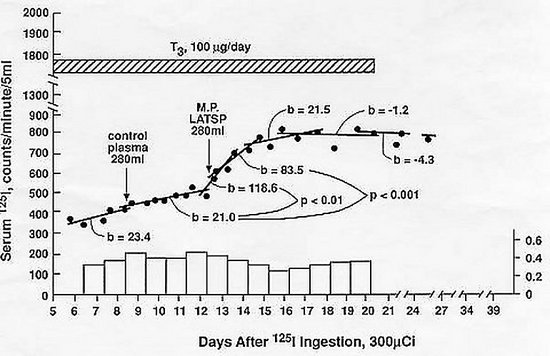
Figure 1. Stimulation of thyroid secretion by LATS-P, a form of TSAb. The subject's thyroid iodine was labeled by administration of 131I, and serial observations were made on the appearance of 131I-labeled hormone in blood (ordinate) over one month (abscissa). An infusion of 280 ml control plasma had no effect, but 280 ml plasma from a patient with Graves' disease caused a marked stimulation of secretion of hormone from the thyroid. (D.D. Adams et al., J. Clin. Endocrinol. Metab., 39:826, 1974. Used with permission of the authors). Of interest, the subjects initials were D.A.
Over the past three decades, an enormous literature has grown around the identification, quantitation, and pathophysiologic importance of these anti-receptor antibodies [46-48]. The antibodies can be classified into at least three general types. Thyroid stimulating antibodies (TSAb, sometimes TSI) interact with the TSH receptor in a positive functional manner and initiate the adenyl cyclase function and the phospholipase A2 function of the receptor, causing all aspects of thyroid stimulation [49-51]. Functionally, this is probably identical to the effects induced by TSH itself. Other antibodies can interact with the receptor in a slightly different manner, presumably by binding to different epitopes on the receptor, and can block the binding of TSH to the receptor while not themselves stimulating function. These antibodies are known as thyroid stimulation blocking antibodies, or TBAb [52-55]. A third set of antibodies can bind to the receptor but neither stimulate nor inhibit its function. These are known as thyrotrophin binding immunoglobulins. They are commonly recognized by assays which detect their ability to interfere with the binding of TSH to the receptor, and are identified as TRAb, or TBII [53]. Probably all patients with Graves' disease have a mixture of all of these antibodies. If TSAb predominate, thyroid stimulation occurs and, if the activity is sufficient, the patient may become hyperthyroid and be characterized as having Graves' disease. If the antibodies block the action of TSH, they may induce hypothyroidism, in which case the patient might be characterized as having Hashimoto's thyroiditis or idiopathic myxedema
TSAb, TBAb, and TRAb
TSAb are usually identified by an assay which quantitates the ability of the antibodies to stimulate the adenyl cyclase function of the TSHR membrane receptor. Either thyroid cells or thyroid cell membranes can be used, and the cyclic AMP produced by this stimulation is quantitated by a radioimmune assay [49]. A cyclic AMP responsive luciferase construct has been stably introduced into CHO cells, allowing a sensitive luminescent assay for thyroid stimulating antibodies with the capability of high throughput suitable for use in general laboratories [50].Assays which measure DNA synthesis of the thyroid cells, or some other aspect of cell growth such as incorporation of thymidine, may or may not measure the same type of antibodies. Antibodies having this action are called thyroid growth stimulating antibodies, to indicate a potential difference [52], and are reported to be present in sera of patients with multinodular goiter. TBAb are measured in the same kind of assay as are TSAb. However, in their assay, a basal level of stimulation is obtained by using bovine or human TSH, and then the ability of the TBAb antibody to interfere with this stimulation is quantitated as "blocking activity" [53]. TRAb are typically measured by their ability to interfere with the binding of radiolabelled TSH to thyroid cells or thyroid cell membranes. These IgGs can be of several subclasses, and have a restricted clonal but not monoclonal origin [56]. They can bind to certain animal TSH-R molecules and to TSH-R (apparently) present on fat cells [57]. Although some studies suggest limited clonality of the anti-receptor antibodies, several studies indicate that both types of light chains are present, and the antibodies may be of a mixture of IgG1, IgG2, IgG3 and IgG4. Antibodies with lower affinity can be found in normal patient sera.
TSAb mediate the thyroid hyperactivity and hypersecretion characteristic of Graves' disease. Presumably low levels of TSAb can stimulate the thyroid in a way that replaces TSH stimulation, and can make the thyroid non-suppressible by administered thyroid hormone, but not cause overproduction of hormone. However, when TSAb reach a certain level of function, they cause an increased secretion of thyroid hormone and produce hyperthyroidism.
The TSH receptor is formed as one polypeptide chain and inserted into the thyroid cell plasma membrane. It undergoes a processing that is reminiscent of that occurring with insulin. A segment of 30 or more amino acids is cut out of the receptor at approximately residue 320, forming a two peptide structure with the chains held together by disulfide bonds. It is thought that both the intact and the processed receptor are functional. The processing of the receptor is thought to involve a matrix metalloprotease-like enzyme cleaving the 120 kDa precursor to form the heterodimeric receptor. Subsequently, reduction of the disulfide bonds by a protein disulfide isomerase may separate the two molecules and lead to shedding of the “alpha” subunit. It is an interesting concept that shedding of the alpha subunit might be intimately related to onset of autoimmunity against the TSH receptor. Shedding of the receptor is augmented by TSH stimulation of thyroid cells (58). The amino-terminal ectodomain of the human TSH receptor has been expressed on the surface of CHO cells as a glycosylphosphatidylinositol-anchored molecule. This material can be released from the cells and is biologically active in that it binds immunoglobulins from serum of patients with Graves’ disease, and displays saturable binding of TSH (46), indicating that all of the "immunologic information" related to production of antibodies resides in the extracellular portion of TSH-R.
The initial bioassay for TSH developed by Adams [42], and then by MacKenzie [59], could quantitate TSAb (or LATS as it was then known) in up to 60% of patients with active Graves' disease. Recent assays measuring thyroid stimulation by cyclic-AMP formation can detect TSAb in over 90% of patients with active thyrotoxicosis [60]. Newer assays being described may increase this to near 100%. The presence of TSAb is thus presumed to be characteristic of active Graves' disease, and if the thyroid can respond, induces hyperthyroidism. The natural course of such antibody action on the thyroid in the untreated state is not usually observed at present. However, the stimulating antibodies are typically associated with other antibodies, and cell-mediated immunity, which damage the thyroid. In time, if the patient survives, the thyroid may be destroyed, or develop blocking antibodies, and the patient may become hypothyroid. TSH-R antibodies are found in patients with Hashimoto's thyroiditis, infrequently in patients with toxic multinodular goiter, and rarely in "normal" subjects [61].
During antithyroid therapy TSABs tend to decline, and if present in significant concentration at the end of a period of therapy, the patient rarely enters remission [62]. Similarly, after surgery, TSAb tend to decline if the patient enters a euthyroid state [63]. After radioactive iodide therapy, TSAb are stimulated to increased levels during several months or a year, probably because of release of antigens [64,65]. TSAbs gradually return to lower levels during the subsequent years. It is also possible that, during antithyroid drug therapy, some sort of immune modulation occurs and the predominant stimulating antibodies are replaced by antibodies which have binding or blocking activity. The specific epitopes to which the thyroid stimulating antibodies bind on the TSH receptor have not been identified. There is evidence that TSAb bind to sequences in the amino terminal portion of the extracellular domain, while those with blocking activity tend to bind to sequences at the carboxy terminal portion nearer the plasma membrane [66].
Lymphocyte reactivity to TSH-R
Lymphocytes of patients with Graves' disease are reactive to TSH-R , as shown by their proliferation during in vitro incubation with TSH-R in vitro [67], and to peptides derived from the TSH receptor. We have shown that patients' lymphocytes react to peptide sequences in TPO (AA110-129, 211-223, 842-861, and 882-901), as well as from TSH-R (AA44-62, 158-176, 237-252, and 248-263)[ 32,68-70]. Thyroid stimulating hormone receptor specific T cells have also been developed from thyroid tissue of patients with Graves’ disease [71]. TSHR peptides (GLKMFPDLTKVYST) and (ISRIYVSIDVTLQQLES) that have aspartic or glutamic acid in in position four of their binding motif are partiularly active inducing Tcell responses in Graves’ disease and immunized animal T cells, and are probably important in developing the immune response to TSHR (72).
It is probable that immunity to TSH receptor plays a direct role in the development of Graves’ ophthalmopathy, probably mediated by immune cell secretion of imflammatory cytokines.. Crisp et al observed immunoreactive TSH-R in samples of normal and orbital fat. Up to 5% of orbital preadipocytes displayed TSH-R reactivity. Differentiation of preadipocytes into adipocytes was induced by TSH stimulation, and on differentiation, more of the adipocytes displayed TSH-R reactivity, and also cyclic-AMP production after TSH stimulation [73-74].Bell et al also found TSH-R mRNA in both orbital and abdominal adipose tissue samples, and TSHR protein in these tissues. TSH activated preadipocytes. In addition to the relation to Graves’ ophthalmopathy, these authors suggest that TSHR signalling may be important in adipose tissue development [75]. Haraguchi et al report that TSH causes proliferation and inhibits differentiation of rat preadipocytes, again supporting the idea that TSH-R may be an important regulator of this process in animals and possibly in man , at least in adipocytes in the orbit[75]. Immunity to TSH-R is believed to lead to production of cytokines in the orbital tissue, which actually mediate the inflammatory process. Hiromatsu et al investigated cytokine profiles in eye tissue and found that Th1 cytokines such as IFNγ, TNFα, IL-1β, and IL-6 were primarily present in eye muscle whereas IL-4 and IL-10 were detected in many samples of orbital fat. Thus both Th1-like and Th2-like immune reactivity may play a role, although it may differ in the involved tissues [76]. Wakelkamp et al found that serum concentrations of sIL-2R, IL-6, sIL-6R, TNFα, and sCD30 were elevated in Graves’ ophthalmopathy patients compared to controls. They also conclude that both Th1 and Th2 type cytokines are elevated in these patients [77].
The role of TSH-R antigenic stimulation is also suggested by triggering of Graves’ disease by treatment of non-toxic goiter with large doses of 131I, which releases antigens from the thyroid, with some patients developing typical ophthalmopathy [78].
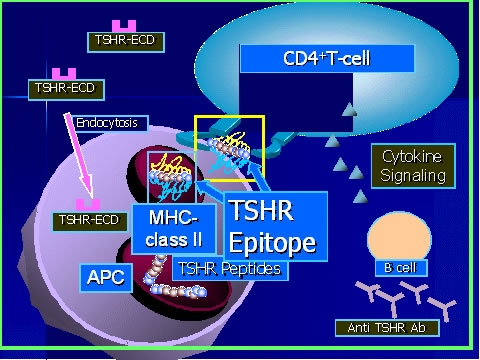
Figure 1a-Schematic representation of TSH-R endocytosis by antigen presenting cells, subsequent proteolysis of the receptor into 9-20 amino acid fragments, their “presentation” in DR molecules to T cells, which help B cells produce antibodies, leading to production of TSAb. (Figure courtesy of Hidefumi Inaba, MD)
The importance of immunity to the TSH-R as the basic effector in Graves' Disease is strongly supported by the development of animal models. Ludgate and coworkers immunized outbred mice by injection of a plasmid expressing TSH-R, and the mice developed some features of Graves’ disease [79]. Nagayama and associates and others have developed this model immunizing mice with an adnovirus or plasmid expressing the A-subunit of TSHR, developed TSAb and elevated T4 levels, and in some studies evidence of ocular pathology(81,82). In line with the role of HLA DRB1*0301 fostering Graves in humans(see above), mice made transgenic for expression of this human HLA protein are more susceptible to this induction of "Graves' disease" than are mice transgenic for other HLA proteins(72,80).
Auto-antibodies to IGF-1 Receeptor-??
A role for antibodies binding to and stimulating the IGF-1 receptor has been proposed. IgG isolated from the sera of patients with Graves' disease (GD-IgG) provoked the synthesis of hyaluronan in orbital fibroblasts. The effect of GD-IgG was reproduced by IGF-I, and appeared to be mediated through the IGF-I receptor. In contrast to the effects in GD fibroblasts, cultures derived from donors without known thyroid disease fail to respond to GD-IgG or IGF-I. The observation that hyaluronan production is induced by GD-IgG in fibroblasts suggests that the IGF-I receptor and its activating antibodies could represent a pathway through which important pathogenic events in thyroid-associated ophthalmopathy are mediated (83). B-cells from patients with Graves” are reported to aberrantly express the IGF-1-R, suggesting that these antibodies could stimulate their own production by B cells (84 ). IGF1R antibodies exist in sera from about 10% of “normal” subjects, and a similar percent of GD patients, and are stable over time, indicating they have no unique role in GD(85 ). Gershengorn et al demonstrated that the monoclonal hTSHR Ab, M22, stimulated HA secretion by GO fibroblasts/preadipocytes and this involved cross talk between TSHR and IGF-1R(86). This cross talk releys on TSHR activation rather than direct activation of IGF-1R and leads to synergistic stimulation of HA secretion. It currently seems unlikely that specific anti-IGFR antibodies play an important role in GO. However IGF1,PDGF-AB and BB augment the action of TSH and TSHR antibodies to produce hyaluronan by orbital fibroblasts (87), supporting the idea that coincident stimulation of TSHR and IGFR on fibroblasts of adipocytes may be important.
CAUSES OF GRAVES' DISEASE
Since Graves' disease is accepted as a disease syndrome induced by autoimmunity to the thyroid, the question of cause resolves into why autoimmunity to the thyroid is present. It is not clear that any other "cause" of Graves' disease is present, other than disordered immunity. There is, for example, no evidence that the thyroid or its protein antigens are initially abnormal [88]. Contemporary understanding is that the process involves a variety of factors allowing self-reactivity to occur. ( Table 10-2) While our immune system is designed to prevent self-reactivity, to some extent, very low levels of self-reactity are normally present [2]. Presumably genetic and environmental factors interact to augment this immunity, from a low and physiologically unimportant level, to a degree that causes a disease state. Several such factors can be identified with some certainty, and others have been suggested and will be noted. It always remains possible, when a specific individual cause is not known, that ultimately one single cause of Graves' disease will be discovered. Current ideas suggest this is not the case.
Table 2. Possible Factors in the Immunological Etiology of Graves' Disease |
Persistence of some autoreactive T cells and B cells (failure of negative selection) • Inheritance of specific HLA, CTLA-4, and many other immune-response related genes • Re-exposure of antigens by thyroid cell damage • Reduced or dysfunctional regulatory T cells • Cross-reacting epitopes on environmental and thyroid antigens • Inappropriate HLA-DR expression • Mutated T or B cell clones • Activation of T cells by polyclonal stimuli • Stimulation of the Thyroid by Cytokines |
Environmental Factors- Recognised “Causes” of Graves’ disease
Considerable information has accumulated about factors in the environment that can induce Graves' disease. Damage to the thyroid, by radiation or ethanol injection, with liberation of antigens, has been noted above. Induction of thyroid autoimmunity, including Graves disease, following therapeutic use of IL-1alpha, IL-2, IFN alpha and gamma, CAMPATH, and drugs used in HIV treatment, are noted below. Cigarette smoking increases risk for GD about 2 fold, while alcohol use and physical activity seem unrelated, and obesity decreases risk(89).
Thymic Selection of Lymphocytes
Lymphocytes develop from precursors present in the bone marrow and mature in the thymus, where they undergo progressive maturation and selection. Lymphocytes which fail to recognize endogenous HLA molecules undergo negative selection, as do those which strongly react with endogenous epitopes presented by HLA molecules [90,91]. In this process, 95 - 98% of all lymphocytes developing in the thymus undergo apoptosis. Spitzweg et al report that NIS, TSH-R, TPO, and Tg-RNAs are present and processed to immunoreactive peptides in the human thymus, and other groups have reported similar findings (92,93). These data suggest that pre-T lymphocytes may be educated in the thymus to recognize thyroid-related epitopes, and thus to generate self-tolerance against these thyroid-related antigens. Expression of these thyroid antigens in the fetal thymus is under control of the AIRE gene, and absence of this gene leads to a fatal poly-autoimmune disease. In Downs syndrome , despite having 3 copies of the AIRE gene, expression of thyroid antigens in the thymus is reduced, and this is thought to cause the elevated incidence of autoimmunity in Down’s (94 ).Clearly this process in imperfect, since cells reacting with these antigens are present in the peripheral blood of normal patients and those with autoimmune thyroid disease [92]. Presumably, in a developmental process designed to provide the maximum repertoire of lymphocytes, some lymphocytes which weakly recognize autologous antigens in the context of autologous HLA are allowed to persist in the circulation. Whether this varies from person to person and is involved in the selection for Graves' disease is unknown.
Molecular Mimicry
A persistent theory on the etiology of autoimmune diseases is that exposure to a particular peptide epitope in an environmental antigen might develop immune reactivity to an amino acid sequence identical to that present in an human endogenous antigen such as TSH receptor, TPO, or TG. Through this molecular mimicry, exposure to a virus or bacteria could produce heightened immune reactivity to a component of the body. This sequence is believed to play a role in rheumatic fever and glomerulonephritis. No certain examples of this sequence have been shown for thyroid disease. However, there is some evidence that proteins present in a common intestinal parasite, Yersinia Enterocolitica, may induce antibody reactivity to TSH receptor [95,96]. These proteins actually appear to be coded for by plasmids which live within the pathogenic bacteria [97], and a specific sequence in the plasmid (DALYGNVTS) is similar to a sequence (198-DAFGGVYS-205) in TSHR. A higher proportion of patients with Graves' disease have been infected with this bacteria than people who do not have Graves' disease [95], exposure to the bacteria can induce TSH receptor antibodies [96], and TSH appears to bind to a molecule on the bacteria [98]. There is also evidence for infection of the thyroid by foamy viruses [99, (although this is disputed) , and there is clear evidence that autoimmunity to the thyroid is induced by infection with the HLTV1 virus, which causes lymphocytic leukemia [100]. Whether this is due to molecular mimicry of the virus, viral damage to the thyroid, or stimulation through another mechanism such as cytokine secretion, remains uncertain. While molecular mimicry remains tantalizing, the factual evidence for its role in the pathogenesis of Graves' disease is minimal.
Another environmental factor that may have an effect on the development of autoimmune thyroid disease is H. pylori infection of the gastric mucosa. One study reports that up to 85% of patients with autoimmune atrophic thyroiditis have H. pylori infection, and it has been suggested that H. pylori antigens may be involved in the development of autoimmune thyroid disease (101). Interestingly, rosacea is also associated with a high prevalence of H. pylori infection, and eradication of H. pylori leads to an improvement in symptoms of gastritis and of rosacea (102).
Thyroid Injury and Antigen Release
It is definite that certain types of injury to the thyroid are followed by the development of thyroid autoimmunity, including Graves' disease. In fact this is one of the few proven cause of Graves' disease. We recognized three decades ago that radiation to the thyroid in young people was followed by a higher incidence of positive thyroid antibody tests [103]. Hancock and associates have reported a significantly increased risk of Graves' disease, Hashimoto's thyroiditis, and Graves’ophthalmopathy associated with radiation to the neck for Hodgkins disease [104]. The incidence of thyroid autoimmunity is elevated among children and adolescents radiated by the Chernobyl explosion ( 105) Radioactive iodide treatment for toxic multinodular goiter and ethanol injection for cure of toxic thyroid nodules have both been followed by the development of autoimmune Graves' disease [106,107]. Over 1 % of patients treated with RAI for autonomous thyroid disease develop Graves hyperthyroidism after therapy, and the incidence is 10x greater if anti-TPO antibodies are present (108). Thus, in this marvelous but unintentional manner, it has been demonstrated that release of thyroid antigens may add a significant stimulation to a latent low level of thyroid autoimmunity, causing the development of clinically important Graves disease, including ophthalmopathy. Whether viral injury, as in the case of HLTV1, plays a similar role is uncertain, although it has been shown experimentally that Reo virus infection of a neonatal mouse can induce thyroiditis and thyroid autoimmunity [109].
Alterations in immune function and cytokines
(See discussion of Tregs below) Administration of cytokines IFN-alpha, IL-2, and GM-CSF in chemotherapy can augment AITD, or in some cases appear to induce it de novo. These agents may act to magnify latent immunity, and a direct action on the thyroid cell may also be involved [110-112]. Depletion of circulating lymphocytes is used in therapy of Multiple Sclerosis. In a group of 34 patients so treated (using CAMPATH),a humanized monoclonal antibody), one-third developed Graves' Disease within 6 months during recovery from T cell depletion. Patient’s stopping immunosuppressive treatment of TIDM may have “re-bound” development of Graves’ disease. These treatments may deviate the immune system from a TH1 to a TH2 type of response [113]. Most likely these therapies reduce the number of Tregs, or alter the balance between effector cells (Th17, Th 23) and regulatory T cells(114).
Cells expressing the pro-inflammatory cytokines IL-17, IL-22, and IL-23R are increased in blood and thyroid of patients with AITD, including Graves” (114).
Onset or worsening of antithyroid autoimmunity and thyroid dysfunction have been reported to occur during treatment with Interferon-α for chronic hepatitis, or Interferon-β for multiple sclerosis. Interferon-α treatment of patients with chronic hepatitis due to hepatitis C virus is associated with the development of primary hypothyroidism, Graves’ hyperthyroidism, and destructive thyroiditis, and is especially prevalent in women (relative risk of 4.4), and in the presence of existing TPO antibodies (relative risk of 3.9)(116). However, one study of this problem in a large group of patients treated for multiple sclerosis found no evidence of a trend to development of thyroid dysfunction during Interferon-β treatment (117).
Genetic Factors
The increased incidence of Graves' disease in certain families [118,119]and in identical twins [120,121] has for decades indicated a powerful genetic influence on development of the disease. Studies of pairs of twins suggest that the genetic factors account for 79% of the liability to the development of Graves’ disease, whereas environmental factors account presumably for the remainder (121).A representative sample of healthy twin pairs was identified through the Danish Twin Registry; 1372 individuals, divided into 283 monozygotic (MZ), 285 dizygotic same sex (DZ), and 118 opposite sex twin pairs were investigated. Serum TPOab and serum Tgab were measured. Proband-wise concordance and intraclass correlations were calculated, and quantitative genetic modelling was performed. Genetic components (with 95% confidence intervals) accounted for 73% (46-89%) of the liability of being thyroid antibody positive. Adjusting for covariates (age, TSH and others), the estimate for genetic influence on serum TPOab concentrations was 61% (49-70%) in males and 72% (64-79%) in females. For serum TGAb concentrations, the estimates were 39% (24-51%) and 75% (66-81%) respectively (122). Early markers of thyroid autoimmunity appear to be under strong genetic influence.
The first genetic factor to be associated with Graves' disease was HLA-B8 [123], a Class I major histocompatibility component (MHC). Inheritance of this gene, expressed on the surface of antigen presenting cells, was found to confer increased risk of getting Graves' disease. Subsequently, this relation was found to be more specifically with an MHC Class II molecule, HLA-DR3 [124,125]. Inheritance of this gene increases the risk of Graves' disease up to 5.7-fold. Our laboratory demonstrated that the HLA molecule DQA1*0501 was also closely and independently associated with the risk of getting Graves' disease [126,127]. In contrast, inheritance of HLA DRbeta 1*07 appears to be protective [128].
The reason HLA genes are associated with Graves' disease seems now to be clear, although the exact pathway is less certain. These molecules exist as dimers on the surface of antigen presenting cells. In the initiation of an immune response, the antigen presenting cell displays a specific epitope complexed in a DR protein. Recognition of this bi-molecular complex by the T cell receptor leads to stimulation of the T cell. In contrast to the possible 10-7 or higher specificities present on individual T cell receptors, the spectrum of HLA molecules is much more restricted. There are between 50 and 100 different DR molecules, and a much smaller number of DQ and DP molecules, all coded on Chromosome 6, in the human genome [129]. Each human has genes coding for two DR, two DQ, and two DP molecules. Of these, the DR are most abundantly expressed and most important. The HLA molecules exist as dimers on the surface of antigen presenting cells, and their extracellular domains form a structure that can be compared to a hot dog bun, into which the peptide epitope is cradled much like a hot dog in a hot dog bun. (Figure 10-1.1, below) This combination, the DR molecule and its enclosed amino acid epitope of 9 - 20 amino acids, constitutes the structure which is seen and recognized, or not, by the receptor on a T cell receptor.
The amino acid sequences of the DR molecule determine the shape of the antigen presenting cleft, and peptides formed from protein antigens fit into the cleft with greater or lesser affinity, depending upon how well their three dimensional structure fits into the three dimensional structure of the antigen presenting cleft of the HLA molecule [130]. The net effect of this is that certain DR molecules are better able to present certain epitopes. DR molecules which best fit epitopes derived from the TSH receptor, for example, are most effective in presenting the epitope to the T cell to induce immunity. This same recognition sequence is involved in selection of T cells in the thymus and determines whether the T cells are destroyed or allowed to mature(90). Thus the matching of the DR molecule with the structure of the TSHR epitopes, or other thyroid epitopes, plays an important role in determining the development of autoimmunity. Sawai and DeGroot studied the binding of TSH receptor peptide epitopes to the DRB1*0301 molecule known to be associated with Graves’ disease. Epitopes which induce reactivity of T cells from patients with Graves’ disease bound with medium affinity, whereas epitopes which did not stimulate T cells bound with very low affinity(131). De Groot and Inaba provided a specific molecular explanation for this role of DR3 is promoting Graves’ disease. They found that TSH-R-ECD epitopes with aspartic or glutamic acid (D/E) in positions 71 and 74 in the DR sequence (important in defining the shape and charge of pocket 4 on the surface of the DR molecule) bind more strongly to DRB1*0301 than epitopes that are D/E- and are more stimulatory to GD patients' peripheral blood mononuclear cells and to splenocytes from mice immunized to hTSH-R (72). This effect is due to the presence of arginine at position 74 in the β-chain of the HLA-DR molecule, giving a strong positive charge to pocket 4. Thus epitopes with D or E at specific positions in their motif are important in immunogenicity to TSH-R due to their favored binding to HLA-DR3, thus increasing presentation to T cells.
These data support the concept that the ability of specific peptides to bind in the antigen binding cleft of the HLA molecule is the reason for the association between the HLA Class II DR3 or DR5 molecules and the development of Graves’ disease [2]. Individuals who have DR3 antigen, or HLA-DQA*0501, tend to develop Graves' disease [124-126]. Those who have DR5 tend to develop Hashimoto's thyroiditis [132]. This correlation does not necessarily hold perfectly true from one human race to another, but there is a general similarity that suggests great importance for this relationship. However, the relationship between DR gene inheritance and Graves' disease is such that it can account for about a 2 - 5-fold increment in risk, which is certainly not enough to explain the marked increase in risk for Graves' disease seen in many families.
The importance of the HLA-DRβ1*0301 gene in autoimmune thyroid disease has been demonstrated by using transgenic mice. Transgenic mice expressing DR3 were susceptible to induction of thyroiditis following immunization with thyroglobulin, whereas animals transgenic with DRβ1*1502 (DR2) were resistant(133). Pichurin et al found that transgenic mice that express HLA-DR3 without their own murine MHC are prone to develop TSH receptor antibodies after vaccination with TSHR-DNA in a plasmid, whereas control mice that were HLA DQ6b transgenic did not develop the antibodies. Some of the sera recognized a linear peptide sequence present in the amino terminus of the TSHR (134).
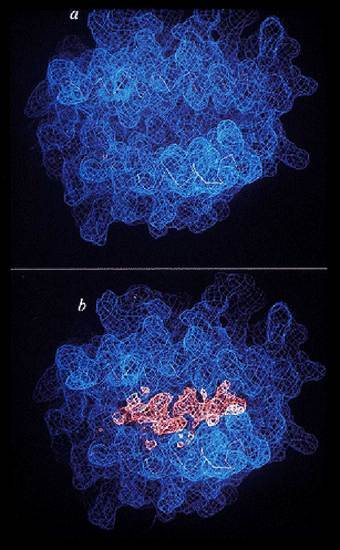
Figure 1.1. In this remarkable x-ray crystallographic study, an HLA Class I molecule is seen from above. The two interlocking subunits form an antigen binding cleft into which the peptide epitope must fit and remain if it is to be recognized by the T cell receptor(a). In (b), fortuitously, a peptide epitope is found occupying the cleft, fitting like the hot-dog in a bun.
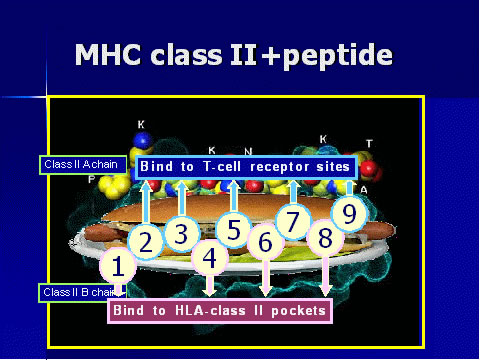
Figure 1.2- Schematic diagram of how a peptide, such as one derived from TSH-R, can fit into the 9 “pockets” formed on the surface of a DR molecule by the peptides in the alpha and beta chains. The amino acids fit into a groove with one aspect of their structure facing the DR molecule, and the other facing outward and becoming, with the surface of DR, the structure that is recognized by specific T cells.
CTLA4:
A second gene was found to be related to the propensity to develop Graves' disease, and this gene also is involved in immune responses [135,136]. When an immune reaction begins, the "first signal" is through the recognition by the T cell receptor of its cognate epitope presented in an HLA molecule. However, if only the first signal is received by a T cell, the T cell tends to be turned off or “anergized”. In order for a progressive immune response to recur, there must be a "second signal" provided by one of several adhesion molecules which exist on the APC and T cell, and which tend to augment the affinity of the interaction [137]. Of these, one of the most important is "B7", which exists in two forms, B7.1 and B7.2, present on the surface of APCs. These molecules interact with their cognate receptors on the T cell, CD28 for B7.1 and CTLA4 for B7.2. In many situations interaction between B7.1 and CD28 give a positive stimulus to growth of the T cell, whereas interaction with CTLA4 provides a negative signal [138]. CTLA4 exists as a gene with several isoforms. These are due to a polymorphism in the leader sequence, and an AT dimer polymorphisms in the 3’-untranslated region of exon 3, which are also closely linked with the polymorphism in exon 1 [139]. It has been found that inheritance of the 106 base pair AT polymorphism is associated with a greater incidence of Graves' disease, especially in males [135,136]. The G (alanine ) position 49 allele was found to be linked to Graves' Disease by Heward et al [140] and Vaidya et al found in a linkage study that inheritance of a specific CTLA-4 allele along with MHC allele was responsible for 50% of the genetic influence in Graves' disease [141] CTLA gene polymorphism studies indicate that the G allele, associated with the development of Graves’ disease, also influences higher production of TPO and Tg antibodies [142].
Kouki and De Groot investigated the relation of the CTLA-4 alleles to proliferation of T cells in patients with Graves’ disease and Hashimoto’s thyroiditis. They found that T cells from all subjects that have the G polymorphism, including normal controls, proliferate to a greater degree than do lymphocytes bearing the CTLA-4 A polymorphism. This is presumed to give individuals carrying the G polymorphism a mild but important greater propensity for development of a functional auto-reactive lymphocyte clone [143].
Interestingly, the HLA association suggests a relationship to disease specificity, since it has to do with the presentation of specific antigen epitopes, whereas the CTLA-4 polymorphism appears to be a general phenomenon, allowing one population group to have augmented lymphocyte proliferation, but is not specifically related to the disease. These observations also fit with the concept that development of Graves’ disease is mediated by a set of genes rather than one specific gene. It is reasonable that a variation in the function of the CTLA-4 gene makes it less effective, as a suppressive signal controlling autoimmunity. The genetic effects of DR genes and CTLA-4 interact. Specifically, it has been found that the positive effect of CTLA-4 predisposition mitigates in part the negative effect of DRB1*0701, but does not interact with the positive influence of DRB1*0301(33)
Numerous other gene polymorphisms have been reported to be associated with GD. Very likely these genes all provide a real but small increment in the chance of developing GD. It has been indicated [144], and denied [145], that an association exists between a specific polymorphism of the TSH receptor (PRO52THR), and susceptibility to Graves' disease. Alleles of intron 7 of the TSH-R gene were found associated with GD in Japanese patients(146). Linkage to the TSH-R gene has recently been confirmed by Dechairo et al [147]. The TG gene has been linked to Graves and other AITD, but to date evidence for this relation is uncertain. A Vitamin D receptor exon 2 initiation codon polymorphism has been associated with Graves’ disease in a Japanese population. A similar association has been reported with IDDM and multiple sclerosis [149]. Vitamin D and its receptor are involved in control of autoimmunity, so an association is not surprising, but the mechanism remains unknown. Inheritance of specific V genes coding for immunoglobulins may carry the same kind of risk. Several possible genes linked to Graves’ disease or autoimmune thyroid disease have been found by linkage studies, including one recently described at a locus on chromosome 18q21 that is also associated with IDDM. (148).
Linkage studies of Graves’ family members have suggested the susceptibility locus on chromosome 20q11, which has been named GD-2 by Davies et al, is related to the gene CD-40, expressed on B cells and other immune cells. Recently this group identified a polymorphism in the Kozak sequence of the CD40 gene at position –1 from the translational start site. The CC genotype was associated with Graves’ disease and gave a relative risk of 1.6. Previous studies using a mixed population of patients had not supported such a linkage, but in a Caucasian population, this association and linkage were shown(150). The frequency of C/C genotype of CD40 was increased in GD compared to controls, but the difference was not significant (60.5% versus 55.8%, p = 0.062, odds ratio [OR] = 1.21, 95% confidence interval [CI]: 0.96-1.53). In a meta-analysis with the data from previous studies, the combined OR for the C/C genotype as a risk factor for GD was 1.22 (95% CI: 1.08-1.38, p = 0.001). There was no interaction between CD40 genotypes and other GD susceptibility alleles. No significant genotype-phenotype associations were found. The CD40 C-T polymorphism appears to have a modest effect on genetic susceptibility to sporadic GD(151).
A promoter polymorphism of the CYP27B1 gene has been associated with Graves' Disease and other autoimmune diseases (152). Interestingly, this gene catalyzes the conversion of 25-OH-D to 1,25-OH-D, the active metabolite of D. A possible relation of Vit D receptor to Graves' was noted above. A B cell specific gene ("ZFAT") has been linked to autoimmune thyroid disease, though not specifically to Graves (153). IL-13 gene polymorphisms were studied in Japanese GD patients and healthy control subjects without antithyroid autoantibodies or a family history of autoimmune disorders. A C/T polymorphism at position -1112 of the promoter region was measured using the direct sequencing method, and an Arg-Gln (G2044A) polymorphism in exon 4 was examined using the PCR-restriction fragment length polymorphism method. IL-13 gene polymorphisms are associated with GD susceptibility in Japan(154).
The lymphoid tyrosine phosphatase, encoded by the protein tyrosine phosphatase-22 (PTPN22) gene, is a powerful inhibitor of T cell activation. Recently, a single-nucleotide polymorphism (SNP), encoding a functional arginine to tryptophan residue change at PTPN22 codon 620 in Caucasians has been shown to be associated with GD and other autoimmune diseases. This variation inhibits function of the gene, which is normally to down-modulate signaling via binding to Csk and phosphorylation of Lck. Using a polymerase chain reaction (PCR)-restriction fragment (XcmI) assay to examine genotypes at the codon 620 polymorphism in 334 unrelated patients with AITD and 179 controls, none of the patients with AITD and controls had the tryptophan allele. Of interest, knockout mice deficient in this gene do not develop signs of autoimmunity, suggesting it may not be important in etiology of AITD (155).
The +869T/C polymorphism in the TGF-beta1 gene has been associated with the severity and intractability of both Graves’ disease and thyroiditis(156). CD25 has been related to Graves’ disease, and IL-23R to ophthalmopathy(157). A significant association of the Interferon Inducible Helicase, Ala946Thr, IFIH1 polymorphism to organ-specific autoimmune diseases including Graves' disease.was reported by Sutherland et al (158). IL-1alpha and IL-1beta polymorphisma are associated with Graves’on Asian populations (159). Gene associations with GD or AITD, and a long list of othergenes-TNFAIP3, FoxP3, TBX21, HLX, BTNL2, Notch4, and CXCR4, has been reported.
Although linkage analysis has often been considered to be superior to gene association studies for determining genetic effects in autoimmune diseases, in fact linkage analysis may be limited in defining such loci, and large-scale association studies may prove to be more useful in identifying genetic susceptibility factors for AITD. A genome-wide screen was performed on affected relative pairs with autoimmune thyroid disease. 1119 Caucasian relative pairs affected with autoimmune thyroid disease (GD or AIH) were recruited into the study. The study aimed to identify regions of genetic linkage to AITD. Three regions of suggestive linkage were obtained on chromosomes 18p11 (maximum LOD score 2.5), 2q36 (maximum LOD score 2.2) and 11p15 (maximum LOD score 2.0). No linkage to HLA was found. The absence of significant evidence of linkage at any one locus in such a large dataset argues that genetic susceptibility to AITD reflects a number of loci each with a modest effect (78.17).
The final result of this kind of research is not clear at present. The most obvious conclusion is that there are several -- probably very many -- genes which augment the possibility of developing immunity to thyroid gland protein components. Thus inheritance is polygenic. Rather than inheriting one gene which, in a dominant fashion, induces Graves' disease, individuals inherit many different genes which are related to the development of thyroid autoimmunity. If a sufficient load of the positively acting genes is inherited, they support the development of Graves' disease or other AITD, especially if other factors are present such as injury to the thyroid. A dramatic illustration of the genetic influence is provided in a recent report of "adoptive" hyperthyroidism following allogenic stem cell transplant from an HLA-identical twin with Graves’.[161]
Gender
Perhaps the clearest association with autoimmune disease is being a member of the female sex, which carries a 10 - 20-fold risk compared to the male sex. Despite this obvious association, the mechanism has remained obscure. The association carries through not only for autoimmune thyroid disease but also for the development of multinodular goiter, and even differentiated thyroid carcinoma, but not undifferentiated thyroid carcinoma. Thus female gender may endow a generally greater reactivity of the thyroid gland, or may subject it to greater stress in some manner. It has been suggested that there may be specific receptors on the promoter for DR genes, which makes them responsive to the estrogen receptor. In a Polish population the ESR2 A allele is associated with GD with a strength comparable to polymorphisms of PTPN22 and CTLA4 CT60 loci (OR approximately 1.7). The association with ESR2 is found in both sexes and may be particularly strong among the DRB1*03-negative individuals(162)
Other Suggested Causative Factors
A variety of other ideas have been presented as the "cause of Graves' disease", but remain unlikely or unproven. ( Table 10-3) Mutation of T or B cells to produce a specific reactive clone has been suggested [163]. Of course somatic mutation is a known part of the development of B cell clones, and specific mutations of B cells or T cells can produce tumors rather than a disease producing autoimmunity.
Regulatory T cell abnormalities
Every immune response involves development of a set of effector T cells and at the same time a set of regulatory T cells designed to temper the response. Current research gives the regulatory cells a prominent role in controlling anti-self immunity, as seen in myasthenia, multiple sclerosis, thyroid autoimmunity, and Type 1 Diabetes. A common assumption has been that numbers or function of Tregs would be deficient in human autoimmunity, since mouse knock-out models and human mutations leading to deficient expression of FoxP3 (and CTLA-4) lead to broad and sometimes fatal autoimmunity(164).
An important class of Tregs is characterized by expression of CD4, CD25 (IL-2Rα subunit), FoxP3, GITR (glucocorticoid inducible TNF receptor), CTLA-4 (cytotoxic T lymphocyte associated antigen), GADD45 alpha and beta (growth arrest and damage inducible proteins), and a low level CD127 (IL-7-R subunit alpha chain) (165). The FoxP3 transcription factor is one hallmark (but not always reliable) for Treg suppressor function (166,167). CTLA-4 is a negative regulator of T cell proliferation. Triggering of the Stat signal pathway by IL-2 and IL-7 is essential for survival and proliferation of Tregs.
Tregs are comprised of several different cell types. “Natural” Tregs (nTreg) develop in utero within the thymus, recognize self-antigen, and are CD4+CD25+GITR+FoxP3+. They are IL-2 dependent and commonly anergic in vitro in the presence of antigen(168). Inducible Tregs (iTregs) develop in the periphery from CD 25lo cells (169). TGFbeta can promote the development of CD4+CD25- T cells into CD4+CD25+CTLA-4+, GITR+,FoxP3+ cells, unless exposure to IL-6 re-directs their development into Th17 effector T cells expressing RORgammaT (170) (Fig. 1.3). Tregs have been shown to function by cell-cell contact or in some systems by secretion of TGFbeta and/or IL10 and may directly suppress effector T cell reactivity, or act via induction of other Tregs (171). Natural Tregs also induce autoantigen-specific adaptive Tregs (172,173).Several other cell types such as CD8+ cells and CD69L+ cells (173) also have been shown to function as regulatory T cells, and their importance in Graves’ disease is unknown. The ability to induce non-antigen-specific bystander suppression is a hallmark of Tregs.
Tolerance can develop in the periphery when T cells are converted to an adaptive (aTreg) (or inducible (iTreg) Treg phenotype upon activation via their TCR in the presence of IL-10 and TGF-b. The role of these iTreg cells may be to dampen over-emphatic inflammatory immune responses. iTregs can suppress T helper responses, B cell responses (antibody) and CTL responses. iTreg induction also is associated with sustained tolerance (to autologous proteins) and probably requires the existence of Treg cells with the same antigen-specificity as the self-reactive (effector) T cells. Unfortunately the expression of FoxP3, while characteristic of regulatory T cells, is neither unique nor stable. CD4+FoxP3+ cells do not necessarily display a suppressive function, and more problematically, can be converted in the right milieu, into cells espressing Th17 with an effector function
The role of regulatory cells during development and progression of autoimmune disease has been widely studied. For example, the function of Treg cells appears to be impaired during development of diabetes in NOD mice (174,175). During development of EAE in mice, Tregs fail to suppress the immune response. (176). CD4+CD25High Tregs from patients with active SLE failed to suppress function of CD4+ effector cells in vitro, whereas Tregs from patients with inactive disease were effective(177). CD4+CD25+FoxP3+ cells are reported to be present in normal numbers but to have deficient suppressor function in patients with ITP (178). Tregs are reported to be decreased in number in myasthenia gravis, while CD8+CD122+ Tregs are normal (179). Many other studies on this topic cannot all be cited.
Several groups have investigated Tregs in AITD (Autoimmune thyroid disease) with conflicting results. Nakano et al. found that the proportion of Tregs among intra-thyroidal lymphocytes was lower than among PBMC in patients with GD and thyroiditis and that the Tregs present were apoptotic (180). Marazuela et al. (181) found an increased percent of CD4+ T cells expressing GITR, FoxP3, Il-10, TGF-beta and CD69 among PBMC from patients with autoimmune thyroiditis and that similar cells infiltrated Hashimoto’s thyroiditis tissue. The suppressive function of blood Tregs was defective. Gangi et al. found that administration of GM-CSF induced development of CD4+CD25+ Tregs that suppressed immunity to TG in mice (182). Molteni et al. reported that CD8+ T cells suppressed TSH-R specific CD4+ T cell clones (183). Watanabe et al. reported that relative proportions of CD25+ cells among CD8+ cells, and CD4+ cells were directly related to severity of Hashimoto’s thyroiditis (184). Vaidya et al. reported higher numbers of naïve activated T cells (CD4+ or CD45RA+CD4+) and lower memory T cells (CD45RO+ CD4+) in patients with GO as compared to controls(185). Glick et al found frequency of Tregs normal in 20 HT and GD children but impaired Treg suppresion of Teffectors(186). Klatka et al found lower numbers of Tregs and increased numbers of Th17 cells in adolescents with GD, returning towardnormalwith treatment (187). Our own data indicate that Tregs are normal as a percentage of cells and cell numbers in treated patients and those with active GD (188). In contrast, Mao et al (189) report that circulating FoxP3+Tregs are diminished in untreated GD, and that their function is further reduced by plasmacytoid dendritic cell secretion of IFNalpha and elevated thyroid hormones.
While there is much evidence for abnormalities in Treg number and/or function in GD, there remains some uncertainty. On the otherhand, it is a reasonable assumption that Tregs are at some time, or in some way, relatively inadequate, or patients would not develop GD(189-193).
It is probable that a relative deficiency of such cells explains the appearance of GD during immune system recovery following medical treatment of patients with advance HIV disease (78.3) and after therapy with the lymphocyte depleting antibody CAMPATH.
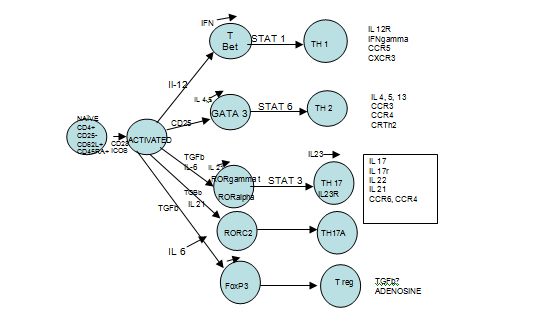
Figure 1.3. After activation, CD4+ T cells may enter several different pathways depending on APC co-stimulatory factors and cytokine milieu. TGF-beta directs T cells to become Tregs, unless IL-6 is present, in which case the cells may be acted on by IL-21 and IL-23, leading to expression of RORgammaT,and STAT 3, and becoming Th17 or IL-17a effector and inflammatory cells. FoxP3+ Tregs can be converted into Th17 cells. Pathways to Th1 and Th2 cells, and secretory products, are also shown.
Fetal cell microchimerism
Intrathyroidal fetal cell microchimerism has been suggested as a possible etiologic agent in autoimmunity. During pregnancy, fetal and maternal cells are transferred between mother and fetus. It has been shown that fetal cells from male infants can persist in the maternal circulation for up to 20 years. Male fetal origin cells were studied in human thyroids by identifying the male specific region of the SRY region of the Y chromosome, and were detected in 6 of 7 frozen thyroid tissue specimens from patients with Graves’ disease, and one of four with thyroid nodules. Fetal male cells are possible candidates for modulating autoimmune thyroid disease, since they might either induce an immune response, or develop a sort of graft-versus-host immune response to the mother (194).
Table 3. Other Factors Suggested in the Etiology of Graves' Disease |
Psychic Trauma • Sympathetic "Overactivity" • Weight Loss • Iodine • TSH • Female gender |
Development of expression of Class I or Class II MHC
Development of expression of Class I or Class II MHC molecules on the thyroid epithelial cell was suggested as a factor in the causation of Graves' disease by Bottazzo et al [195]. It is now apparent that exposure of thyroid epithelial cells to Interferon, presumably elaborated by infiltrating lymphocytes or other immune cells, can lead to the expression of Class II molecules on the thyroid cell surface [196]. Expression of these molecules does allow the thyroid epithelial cell to function as a weak antigen presenting cell [197]. Class II expression is secondary to the effect of an autoimmune lymphocyte attack and is induced by Interferon [198]. Culture of human thyroid cells from patients with Graves' disease in vitro shows that Class II expression disappears [199], as it does when the cells are transplanted into nude mice [200].
It is also possible that the Class II expression is a defensive response [201] Antigen presentation by Class II MHC expressed on a thyroid cell,to a T cell, in the absence of a second signal, could lead to anergy of the attacking T cell. Possibly Class II expression is secondary but may play a role in continuing or strengthening the autoimmune reactivity to thyroid antigens. Antithyroid drugs may have an immunosuppressive effect on autoimmune thyroid disease through inhibition of HLA-DR expression on thyrocytes. Follicular cells of patients with overt thyrotoxicosis express HLA-DR, while those in remission, or those under medication with antithyroid drugs, did not. Recently it was reported that transgenic mice expressing MHC Class II on their thyroid cells do not develop autoimmunity. This is a strong argument indicating that Class II MHC expression on Graves thyroid cells is secondary and not a causative event.
Stress
Psychic trauma or psychic stress has long been considered to be a possible etiology of Graves' disease. In Perry's original report, a crippled woman, who was injured when her nanny allowed her wheelchair to role down a flight of stairs, had rapid onset of thyrotoxicosis. This report, in 1820, has been followed by many more studies, some of which support the idea. The incidence of Graves' disease increased in Denmark during World War II [202], but did not in Ireland during the sustained civil war in that country during the period of 1980 through 1990. A recent study in Yugoslavia indicated that patients with Graves' disease had suffered on average more stressful episodes than control subjects, but previous similar studies have failed to show this relationship [203-205]. A recent article found increased numbers of stressful life events in patients with Graves’ disease prior to onset of the disease, compared to patients in a toxic nodular goiter group who had a similar number as control patients. (206). Stress induces a variety of physiologic responses including anxiety, tachycardia, restlessness, etc., which are not unlike symptoms of Graves' disease. Its role remains enigmatic in causation of Graves' disease to this date. A mechanistic route from stress to the development of Graves' disease is not obvious. Theoretically, stress might cause activation of the adrenal cortex or the sympathetic nervous system. Hypercortisolism would tend to suppress autoimmunity. Heightened sympathetic nervous system activity might theoretically cause stimulation of thyroid secretion, as has been shown in experimental animals [207. Other specific stressors have been reported. Aggressive weight loss programs have been reported to induce Graves' disease. Administration of thyroid hormone, sometimes given for induction of weight loss, also has been followed by mini-epidemics of Graves' disease [208].
Excess Iodide
Iodide itself has been thought to induce Graves' disease, thus leading to the term "Jod Basedow". This syndrome refers to the occurrence of thyrotoxicosis following supplementation of iodide in medicinals or by salt iodinization. Excess iodide clearly does induce hyperthyroidism in patients with multinodular goiter [209,210]. Presumably autonomous nodules in the goiter are unable to produce an excess of hormone when their synthesis is limited by iodide, but when this iodide supply is augmented many-fold, the nodules can process it to produce an excess of hormone. The best defined epidemic of iodide-induced thyrotoxicosis occurred in Tasmania after the government introduced iodization of salt, and was clearly associated with multinodular goiters rather than typical Graves' disease [211,212].
Possibly increased iodide intake can actually augment thyroid autoimmunity through other mechanisms. For example, increased iodide intake has been correlated with an increase in incidence of AITD [213This could in theory work by augmenting iodination of TG, and heavily iodinated TG is more immunogenic in animals than is poorly iodinated TG. Also, under special circumstances excess iodide can induce thyroid cell necrosis, and this might liberate antigens. Adding KI to the diet of the thyroiditis-prone BB strain rat and to NOD mice increases the severity of thyroiditis [214,215].
Whether an excess of iodide can induce true Graves' disease and autoimmunity remains unknown. In fact the addition of 2 - 6 mg per day of iodide to the intake of most patients with Graves' disease, raising plasma iodide levels above 5ug/dl, causes a dramatic reduction in hormone release by the "Wolff-Chaikoff" effect, which is an inhibition of hormone synthesis and of hormone release [216-219]. Iodide is one of the most rapid acting agents in suppressing thyrotoxicosis. While it has this effect in most individuals with Graves' disease, its action tends to be partial or transient, and thus is not relied upon as an effective antithyroid agent. Occasional contemporary reports attest to the ability of iodine treatment to induce remission of Graves” disease in a significant proportion of children over several years. A recent study suggests that the ability of iodide to suppress Graves' disease may be because iodide down-regulates MHC Class I and II expression on thyroid cells [220].
Smoking has been related to Graves' Disease, and more specifically to a greater propensity to develop ophthalmopathy, or to have worsening of the condition. Salvi et al also studied cytokines in patients with Graves’ disease and found serum IL-6 concentrations higher. Interestingly, smoking, which is associated with an adverse effect on Graves’ ophthalmopathy, appeared to have no interaction with the serum lymphokines [221].
Other older ideas on the cause of Graves' disease included a role for TSH, or some fragment of TSH [108], or the HCG molecule. None of these is thought to be involved in thyroid autoimmunity according to current formulations, although excess TSH production by a pituitary adenoma is an established cause of thyrotoxicosis [223, 224], and TSH produces the thyrotoxicosis seen in "Pituitary Resistance to Thyroid Hormone". Vassart and co-workers [225] have recently recognized the cause of non-autoimmune Hereditary Familial Hyperthyroidism inherited in an autosomal dominant manner. In two sibships, activating germline mutations were found in the transmembrane segments of the TSH-R. The mutations cause persistent basal hyperfunction of the receptor and early onset of thyrotoxicosis. Other causes of thyrotoxicosis (but not Graves' Disease) are described briefly in Chapter 11 and more fully in Chapter 13. Numerous other theories regarding the cause of Graves' disease have been proposed in the past. For a critical review of earlier speculations and a superb bibliography, the reader is referred to the monograph by Iversen [226].
THYROID GLAND FUNCTION IN GRAVES’ DISEASE
While the ultimate cause(s) remain uncertain, the patho-physiologic mechanisms are more clear. The thyroid gland is functioning at an accelerated rate. Cell membrane adenyl cyclase activity in tissue from Graves' patients is higher than in normal thyroids, and the increment coincides with TSAb in the serum [227], which acts on TSH receptors to cause the response. Clearance of plasma iodide by the gland is increased from the normal rate of 10-20 ml/min to 40 to several hundred ml per minute. We have estimated iodide clearance in one patient to exceed 2 liters/min. For this reason, the percentage of a tracer dose of 131I (the radioactive iodine uptake or RAIU) found in the gland at 24 hours is characteristically elevated. Thyroid peroxidase activity is increased. Because of rapid secretion, the period of retention of iodine within the thyroid is reduced, causing the characteristic drop in RAIU between 12 and 24 hours after administration of a tracer. The total quantity of iodine in the gland is variable. In previous years it tended to be reduced, but now it is often elevated because of increasing amounts of iodine in our diet. The volume of the gland is characteristically but not invariably increased. Thyroid hormones, TG, small amounts of an iodinated albumin-like protein [219,228], and iodotyrosines [229] are released into the blood at increased rates. The latter two components are normally either not secreted or are released in minute amounts.
Many studies with radioiodine have confirmed the accelerated physiologic activity of the thyroid in Graves' disease. Thus, labeled hormones appear as plasma Protein Bound 131I more rapidly and reach higher levels than in normal persons after administration of 131I. The rate of turnover of plasma hormones is also increased. Accelerated degradation is probably secondary to hypermetabolism and not a primary event [230], although it has been reported that accelerated T4 turnover persists after therapy for thyrotoxicosis [231].
With the general hyperactivity of the thyroid, excess TG is released and serum TG levels are elevated in active Graves' disease. After therapy the levels tend to fall, and normalization during antithyroid therapy is an excellent predictor of remission [232]. This excessive release of TG leads to formation of circulating immune complexes with anti-TG antibodies, and can lower the titer of these antibodies in the serum.
An important abnormality in thyroid function during Graves' disease is that the uptake of 131I by the thyroid is not suppressed by administration of exogenous T4 or T3 ( 233, 234). This fact holds true even if large amounts of hormone are given. Indeed, administration of T3 may cause, on average, a slight increase in the 24-hour radioiodine uptake. This abnormal response to the administration of thyroid hormone occurs in spite of responsiveness of the thyroid to administered exogenous TSH, as measured by augmented release of thyroid hormone. It may persist after thyrotoxicosis has been ameliorated by surgery, but typically suppressibility returns in time after treatment. Non-suppressibility is observed so regularly that it was used for many years as a criterion for diagnosis in doubtful cases. Non-suppressibility is caused by stimulation of the thyroid by TSAb, and independence of feedback control via TSH. There is a general, but not complete, correlation between T3 nonsuppressibility and positive assays for TSAb [235]. Even long after apparent remission of Graves' disease, some patients show some resistance to T3 suppression or TSH stimulation [236]. Also, some euthyroid relatives of Graves' disease patients show these abnormalities in the absence of overt thyroid disease.
The pituitary does not respond to TRH in the thyrotoxic state, either in hyperthyroid Graves' disease or when thyroid hormone is administered. This is because the function of the hypothalamic TRH neurons are down-regulated by excess thyroid hormone levels. Some patients with "euthyroid Graves' disease" respond to TRH and others do not; the responses do not correlate with the results of T3 suppression tests [237, 238]. For the diagnosis of Graves' disease, suppression of serum TSH is currently the most useful criterion, as it is more convenient than the TRH test and preferable to the T3 suppression test in patients with heart disease. Test results are not uniformly abnormal in patients with euthyroid Graves' disease.
IODINE SUPPRESSION OF THE GRAVES’ THYROID
Iodide affects the metabolism of the diffusely hyperplastic thyrotoxic gland in a way radically different from its action on the normal gland. Years ago, Plummer demonstrated that Graves' disease can be temporarily or permanently controlled by the administration of iodide [239]. The amount needed is 6 mg/day or more. Administration of large doses of iodide to laboratory animals causes a temporary inhibition of iodide organification, the Wolff-Chaikoff block. High intrathyroidal iodide concentration is the crucial factor inducing this response [240]. The same phenomenon occurs in humans, and thyrotoxic patients are especially sensitive to this effect. The thyroid uptake of 131I is acutely depressed in thyrotoxic patients by administration of 2 mg potassium iodide, whereas more than 5 mg is needed to depress uptake in normal subjects. Concentrations of serum iodide above 5 µg/dl block binding in the thyrotoxic gland [216-219].
The biochemical mechanism of the Wolff-Chaikoff block is not clear. Iodide does not prevent TSH or TSAb binding to the TSH membrane receptor, but does inhibit both TSH-stimulated adenyl cyclase production of cAMP, and cAMP actions. Since iodide inhibition of cAMP production and action is blocked by methimazole, it is hypothesized that an oxidized iodide intermediate is involved. Alternatively, the block of iodination may be caused by depression of H2O2 generation. Whether the inhibition of iodide transport and binding relate to the recognized changes in cAMP formation is not known (see also Chapter 5).
In animals the block of iodide binding is transient; during continuous iodide administration, binding recommences. This escape also occurs in most normal humans. Few individuals who take large doses of iodine continuously ever develop myxedema. Adaptation to excess iodine in animals involves a reduction of iodide transport into the thyroid, a lowering of intrathyroidal iodide content, and escape from the Wolff-Chaikoff block. This adaptation occurs independently of TSH action. Possibly because the Graves' gland is hyperactive under continued stimulation by TSAb, it may remain blocked by administered iodide, and hormone production may remain suppressed. (Actually in about 1/3 of patients the gland is only partially blocked, and in another third escape occurs after a few weeks.) A similar sensitivity to inhibition by iodide occurs in Hashimoto's thyroiditis, hyperfunctional adenomas, and possibly the normal gland when stimulated by exogenous TSH [241]. Myxedema can often be induced by administration of iodide to patients who have had a partial thyroidectomy for Graves' disease [242-243]. Thus, there is an inherent susceptibility of these glands to the action of iodine. Sensitivity of the gland in Graves' disease to iodide is also demonstrated with the iodide-perchlorate test [242]. In this test, it is seen that the dose of iodide required to block organification in Graves' disease is much smaller than that in the normal subject. The inhibition of binding by iodide is revealed by administration of perchlorate, which discharges the accumulated 131I present in the gland as free iodide.
Coincident with the block in uptake, iodide also causes a marked reduction in the release of previously formed hormone from the thyrotoxic gland. This phenomenon has been repeatedly observed and helps to explain the beneficial therapeutic effect of iodide in Graves' disease, as originally recognized by Plummer. Iodide administration blocks release of hormone from the gland but does not completely prevent hormone synthesis, for under these circumstances the gland gradually accumulates an increased store of organic iodine. Ochi et al [244] have shown that chronic administration of iodide in Graves' disease blocks the stimulating effect on hormone release of both TSH and TSAb. It is this block of release, rather than a block of hormone synthesis, that is responsible for the dramatic rapid beneficial therapeutic effects of iodide administration [245]. The block of hormone release that occurs in the thyroid of Graves' disease can be observed, although not uniformly, in the normal gland and in the normal gland made hyperactive by repeated administration of exogenous TSH. Iodide also inhibits the release of hormone from autonomous hyperfunctioning adenomas, presumably in the absence of endogenous TSH. This observation also indicates that iodide block is by a direct action on the thyroid gland.
Thus, the Graves' gland appears to be unusually sensitive to small amounts of iodide, as manifested by (1) a block of iodide uptake and binding and (2) a block of hormone release. Perhaps these are two parts of the same fundamental process. Sensitivity to iodide may be related to the TSAb dependent, hyperactive, iodide-concentrating mechanism of the Graves' gland.
A further abnormality in intrathyroid iodine metabolism is that the toxic gland continuously spills into the circulation large amounts of nonthyroxine iodide, in addition to hormone [246]. The iodide may be a product of the deiodination of iodotyrosines released from TG during its hydrolysis.
INCIDENCE AND DISTRIBUTION OF GRAVES' DISEASE
The incidence of Graves' disease in Olmstead County, MN was found to be 30 cases per 100,000 annually [247]. A thorough examination of an English town by Tunbridge and associates found an incidence of 100 - 200 cases per 100,000 per year, significantly higher than the previous estimates [248]. In this report, it was also found that 2.7% of women and .23% of men had either current Graves' disease or a history of Graves' disease. This survey also noted that goiter was present in 15% of women, antithyroid antibodies in 10.3% of women, and that hypothyroidism was about two-thirds as common as Graves' disease. A recent update in this area showed a continuing incidence of 80 cases/100,000 women/year [249]. The prevalence of hyperthyroidism was found in the “HANES” study to be 0.5% at the clinical level and 0.7% at the sub-clinical level (250). Data attest to a lifelong incidence of autoimmune thyroid disease of > 6%, comprised roughly equally by Graves' disease, Hashimoto's thyroiditis and idiopathic hypothyroidism.
The distribution of Graves' disease around the globe, so far as data is available, appears to be relatively equal, affecting all countries and races.
Graves' disease is most typically a disease of adult women in the age group between 30 and 60, and has an incidence roughly eight times greater in women than in men [248-249]. Aside from the infrequent occurrence of postnatal thyrotoxicosis due to maternal antibodies, the incidence of spontaneous Graves' disease in children before the age of ten is most unusual, but the incidence climbs with each decade until about age 60 [248-249, 250]. The greater incidence in women is typical of most thyroid diseases including multinodular goiter and differentiated thyroid carcinoma, and the mechanism for this association is unknown. One possibility is that female reproductive activity somehow stresses the thyroid. Another possibility is that the promoter for certain genes such as Class II HLA molecules may have estrogen receptor response elements and thus be activated more easily in women. The well known familial distribution of Graves' disease, recognized by all clinicians caring for patients, is thought to be explained by inheritance of specific genes, as detailed previously.
PATHOLOGY
It should be noted that only the abnormalities of the thyroid, orbital contents, lymphatic system, and skin can be considered specific for Graves' disease; the other lesions probably could be caused by thyrotoxicosis of any cause.
The Thyroid
It is known from observations made before the introduction of iodide or antithyroid drugs that the essential lesion of Graves' disease is parenchymatous hypertrophy and hyperplasia (Figure 10-2). The central features are increased height of the epithelium from cuboidal to columnar; and redundancy of the follicular wall, giving on section the picture of papillary infoldings, cytologic evidence of increased activity, hypertrophy of the Golgi apparatus, increased number of mitochondria, and increased vacuolization of colloid. There is probably nothing specific about the hyperplasia. Any stimulus that calls for sustained hyperfunction produces this picture, such as antithyroid drugs. There is also a characteristic lymphocyte and plasma cell infiltrate. This infiltrate may be mild and diffuse throughout the gland, but more typically occurs as aggregates of mononuclear cells and even lymphoid germinal centers, referred to as focal thyroiditis. Occasionally the histologic pattern completely overlaps that of Hashimoto's thyroidits.
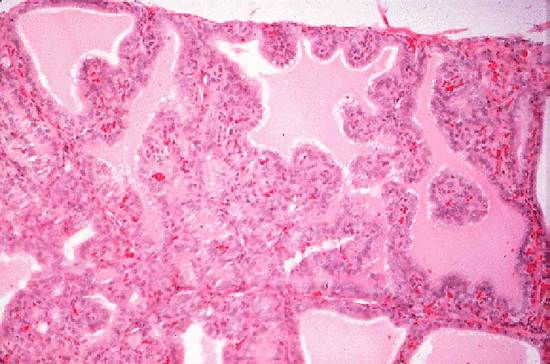
Figure 2. Extreme thyroid hyperplasia in Graves' Disease, with tall cells, small follicles, scant and "scalloped" colloid. Figure kindly provided by Dr. Francis Straus.
Fine structure examination discloses a rather widely varying size and shape of the follicles, with columnar cells and reduced homogeneous colloid [250, 251].The basement membrane is well demarcated and is 400 - 1,000 A thick. Between the follicles is a large array of capillaries, together with lymphocytes and fibrocytes. The apical end of the follicular cell often bulges into the lumen, and cuplike villi extend into the lumen. Vesicles and free ribosomes may be found in these villi. These microvilli vary in size and shape; some may enclose colloid. The nucleus is near the basal part of the cell. The mitochondria are numerous; they are mostly large, elongated structures, and some are branched. The endoplasmic reticulum is usually well developed. Ribosomes occur in great numbers. The Golgi apparatus is well developed. Vesicles are present in abundance, but vary in size and number from cell to cell. Multivesicular bodies are occasionally found near the Golgi apparatus. Droplets, globules, and dense bodies appear. Phagolysosomes are common. All these changes are quite like those of the normal thyroid chronically stimulated by TSH.
There are some data on the thyroid in persons who have recovered from Graves' disease [252]. Autopsy material from seven patients who had recovered from exophthalmic goiter and died later of other causes showed complete regression of the hyperplastic changes.
EXTRATHYROIDAL CHANGES
The ophthalmologic problem and pretibial myxedema, which are unique to Graves' disease, are described in chapter on Complications of Graves’ disease.
Abnormalities in striated muscle may be a part of Graves' disease [252-254 ]. Decades ago Askanazy and Rutishauser [255] studied four patients with hyperthyroidism on whom autopsies were performed. They found a diffuse process in all striated muscles, including the extrinsic muscles of the eyeball, consisting of degenerative atrophy of muscle cells, fatty infiltration, loss of striation and uniform appearance, vacuolization, and proliferation or degeneration of nuclei. Cardiac and smooth muscle were not involved in this process. Not all muscle groups were equally involved, nor were the same muscles involved in different patients.
Dudgeon and Urquhart [256], in studies of the muscles in nine postmortem cases of Graves' disease, found in various skeletal muscles interstitial myositis characterized by plasma cells, tissue macrophages, and atrophy of fibers. Ocular muscles were more affected and cardiac muscle less affected than skeletal muscle. The lesions were spotty and were observed in only a small fraction of the sections. On the other hand, Naffziger [257] examined biopsy muscle specimens from other parts of the body in patients with the ophthalmopathy of Graves' disease and found no abnormalities. The fat content of skeletal muscle may be increased, just as it is in the extraocular muscles [258]. Myocardial degenerative lesions have been reported in thyrotoxicosis, with foci of cell necrosis, mononuclear infiltrates, and mucopolysaccharide deposits similar to those described in extraocular and skeletal muscles [258],and severe damage has been found in patients dying of thyrotoxicosis [146]. (Figure 10-3).
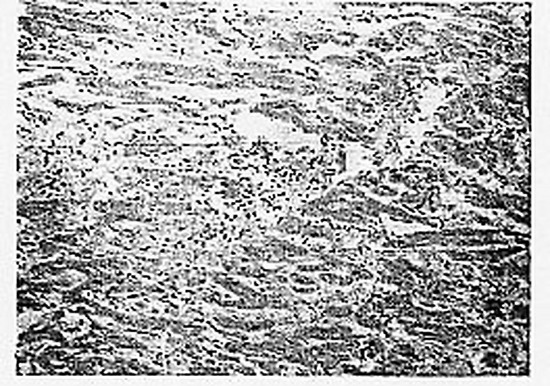
Figure 3. Myocardial tissue from a patient that died of cardiac failure with extreme thyrotoxicosis, showing degeneration of myocardial cells, mononuclear and polymorphonuclear infiltration, and edema.
The extraocular muscle lesions are probably specific for Graves' disease, whereas the remainder of the abnormalities may reflect the action of excess hormone.
The anterior pituitary demonstrates, not surprisingly, a dramatic decrease in identifiable thyrotropin containing cells in patients dying in thyroid storm. This loss is found entirely reversed in patients who come to autopsy after treatment to euthyroidism [260-261].
Lesions specific for Graves' disease do not appear in the parathyroids, gonads, or pancreas.
Studies based on autopsies of patients with Graves' disease made years ago demonstrated focal and even diffuse liver cell necrosis [262], atrophy, and cirrhosis, including a kind of peripheral fibrosis that was believed to be peculiar to this disease -- cirrhosis basedowiana. In more contemporary series of liver biopsy specimens obtained from thyrotoxic persons, the deviations from normal were minimal [263, 264]. Some decrease in glycogen and increase in fat, and some round cell infiltrates were noted. The differences among these studies are at least superficially explicable on the basis of lesser severity and duration of the disease in those patients studied during life.
Hyperplastic changes may be found in the spleen, thymus, and lymph nodes in Graves' disease. The thymus occasionally presents as an anterior mediastinal mass [265] and has been inadvertently resected. Persistence or enlargement of the thymus was once believed to be significant in Graves' disease, and early in the century thymectomies were performed for its treatment, with apparent benefit. The TSH-R is expressed in thymic tissue, suggesting that it might be the target of auto-immunity inducing hyperplasia.
Prolonged hyperthyroidism is known to produce the histologic picture of osteoporosis[266], but osteitis fibrosa also occurs [267]. Histomorphometric studies show clear evidence of excess bone formation and resorption. The high degree of exchangeability of calcium in the bones of patients with thyrotoxicosis and the high rate of loss of calcium in the urine are discussed later in this chapter. Serum 1,25-dihydroxychole-calciferol is decreased, probably in response to increased bone turnover [268].
Cytologic investigations of mitochondria using the electron microscope have revealed anatomic lesions not visible by the ordinary light microscope. Schulz et al [269] reported that the mitochondria from tissues of T4-treated animals appeared to be swollen.
DEVELOPMENT OF THE CLINICAL PICTURE AND THE COURSE OF THE DISEASE
Graves' disease displays an array of possible clinical patterns extending from that of goiter and thyrotoxicosis, but without ophthalmopathy, to that of ophthalmopathy without goiter or thyrotoxicosis.
In classic exophthalmic goiter, or Graves' disease, the most common onset is the simultaneous and gradual development, over a period of weeks or months, of the symptoms of thyrotoxicosis, enlargement of the thyroid, and prominence or related abnormality of the eyes (Figure 10-4). It is quite possible for classic Graves' disease to develop in a patient with preceding (probably unrelated) nontoxic goiter. This is common in a goitrous country. Often the onset of symptoms is so gradual that it is difficult or impossible for the patient or physician to fix its date. The more abrupt onset may sometimes be sufficiently rapid to justify the term fulminating. The picture of classic full- blown exophthalmic goiter has appeared in a person apparently previously well in as short a period as two to five days. Rare patients have first developed hypothyroidism and later thyrotoxicosis [54], probably because the initial development of TBAb is followed by some natural immune modulation and development of stimulatory antibodies. Continuous significantly elevated titers of anti-TSH-R antibodies in the months after diagnosis are positively correlated with serious ophthalmopathy (55).
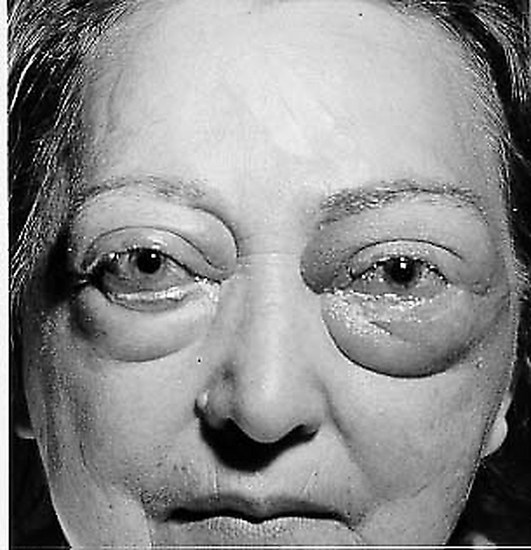
Figure 4. Classic severe Graves' ophthalmopathy demonstrating a widened palpebral fissure, periorbital edema, proptosis, chemosis, and conjunctival injection.
In many patients, the symptoms of Graves' disease are first noted after some emotional trauma. These associations are certainly of importance in understanding the patient's backgound, but, as noted above, whether or not they bear a causal relationship to the development of Graves' disease remains conjectural.
As noted in Chapter 14,Graves' disease is frequently partially or totally suppressed during pregnancy, and initial or recurrent manifestations can occur in the postpartum period. (Figure 10-10) Sometimes the "painless thyroiditis" characteristic of this period co-exists and masks the development of Graves' disease .
Weight reduction, as mentioned above, has also constituted an activating episode in Graves' disease.
At present, the natural history of the thyrotoxic process is usually altered by definitive therapy. Before the general availability of good treatment, hyperthyroidism tended to progress through periods of exacerbation and remission. In perhaps a quarter of the patients, especially those with a mild form of the disease, the process was self-limited to one year or more, as the patients returned spontaneously to a euthyroid state.
M.S., 27-Year-Old-Man: Thyrotoxicosis with Spontaneous Remission
This young physician developed tachycardia, hyperkinesis, decreased heat tolerance, slight tremor, and weight loss over three or four months. On examination, blood pressure (BP) was 150/50, pulse rate 86, and the skin was sweaty. There was a fine tremor. The eyes were entirely normal. There was a grade 1 precordial systolic murmur. The thyroid was about twice the normal size, diffusely enlarged, and firm. There were several cervical lymph nodes bilaterally. PBI was 11(nl 4--8ug/dl), and the rT3U level was elevated. RAIU was 57% and BMR-10. All tests were repeated once, and the results all remained as indicated.
The patient was given 100 mg PTU three times daily and was maintained on this program for 18 months. During this time, the T4 level was maintained in the range of 7.3 ug/dl and the FTI in the range of 6 (nl 4-10); the white cell count remained normal. The TGHA titer was 1/320, and there was a borderline positive TSAb bioassay response. During the course of therapy, the 20-minute technetium uptake test was repeatedly measured while the patient received both antithyroid drugs and suppressive doses of T3; suppressibility of the thyroid gradually fell to the normal range. Eighteen months after the initiation of therapy, the patient developed an acute gastroenteritis and was briefly hospitalized. At this time, because of the possible association of PTU with gastric irritation, the medication was discontinued.
He subsequently remained well for three months, but then developed symptoms of mild hyperthyroidism. The thyroid was again found to be two to three times the normal size, the T4 level to be 10.3 µg/dl, and the FTI to be 11.9. Since the symptoms were mild, it was elected to observe events without therapy for a period. Initially, the symptoms, signs, and laboratory test results remained abnormal, but over several months the mild tachycardia, increased sweating, and increased nervousness gradually dissipated. Six months later, the T4 level was 6.7 µg/dl and the FTI 8. The TSAb bioassay result remained positive. No further treatment was given, and the patient has remained entirely well with a moderate thyroid enlargement, normal thyroid function test results, and no symptoms over the subsequent 30 years.
In one of the few documented reports of untreated thyrotoxicosis, White [270] found that of 12 patients, 7 died in an average of three and a half years and the remainder lived on without therapy. From a large series, Sattler estimated that in the past mortality was up to 11% [271]. Fortunately, death due to hyperthyroidism is now rare, but we are aware of two patients who died of severe undiagnosed and untreated thyrotoxicosis in Chicago within the past few years (259). Deaths most frequently are attributed to cardiovascular complications such as myocardial infarction, arrhythmia, or heart failure, or infections secondary to debility. Some patients become spontaneously hypothyroid, and in fact most individuals apparently cured of Graves' thyrotoxicosis demonstrate evidence of hypothyroidism decades later. Coincident autoimmune thyroiditis presumably plays a role in such thyroid atrophy. Since in some patients treatment of thyrotoxicosis is associated with the spontaneous reestablishment of thyroid homeostasis after a period of enforced reduction in hormone formation (by drugs, surgery, or 131I treatment), it is obvious that the thyrotoxic phase of the disease can be self-limiting.
Toxic crisis, or thyroid storm, was also a frequent feature of Graves' disease in the past. This serious and often fatal development was a marked accentuation of the thyrotoxicosis, with hyperthermia, uncontrolled tachycardia, weakness, and delirium. This situation, now rarely encountered, is discussed in Chapter 12.
The ophthalmopathy of Graves' disease may follow a course quite different from that of thyrotoxicosis. This topic is also discussed in Chapter 12.
SYMPTOMS AND SIGNS OF GRAVES’ DISEASE AND THYROTOXICOSIS
In patients with Graves' disease, the ocular changes, lymphoid hyperplasia, localized abnormalities of skin and connective tissue (e.g., acropachy) and the goiter itself represent parts of the autoimmune syndrome. The remainder of the changes appear to be entirely attributable to an excess of thyroid hormone. Certain systems or organs (e.g., the muscles and cardiovascular system) play paramount roles in the disease, but as far as can be determined, these changes are all fundamentally related to and dependent on the excessive serum concentration of thyroid hormones.
Often the presenting symptoms are weight loss, weakness, dyspnea, palpitations, increased thirst or appetite, hyperdefecation, irritability, profuse sweating, sensitivity to heat or increased tolerance to cold, or tremor. Occasionally, prominence of the eyes or diplopia is the apparent symptom, and goiter may long antedate all other manifestations. Often a relative or friend notices eye signs, goiter, or nervous phenomena before the patient is conscious of any departure from his or her usual status. This asymptomatic phase of thyrotoxicosis is more commonly found in men and children. The excess of thyroid hormone produces an intoxication that in some persons takes the form of exhilaration. They may feel not only healthy but healthier than usual at a time when they are displaying unmistakable objective evidence of thyrotoxicosis. In older patients particularly, the symptom or symptoms may point to the heart more than to any other part of the body, "thyrotoxicosis masquerading as heart disease."
The habitus in Graves' disease shows nothing characteristic. In childhood, those afflicted are tall for their age. This association is an effect of the disease, not an etiologically related variable.
The nutritional state varies greatly. Sometimes the patient is severely emaciated, but on average the weight loss is 5 - 20 lbs. Infrequently, perhaps in 1 out of 10 instances, the patient actually gains weight while thyrotoxic.
The face may may instantly provide the diagnosis. An expression of fright or extreme anxiousness is common, largely because of the peculiar eye signs that may be present. Marked flushing is often noted. A drawn or sunken appearance may result from emaciation or dehydration. It is possible, especially in older patients, to find a considerable degree of thyrotoxicosis without any distinguishing evidence in the facies.
A change in reaction to external temperature is a very classic symptom. The development of a preference for cold weather, of a desire for less clothing and less bed covering, and of decreased ability to tolerate hot weather is highly suggestive of hyperthyroidism.
The tongue tends to be red and smooth; it may also exhibit a definite tremor. The tonsils, if present, are usually rather large, as is the postpharyngeal lymphoid tissue.
The neck is usually conspicuous due to the goiter. It is possible, although rare, for thyrotoxicosis to exist without a visible or palpable goiter. We note reports in the literature that up to a quarter of patients may not have a goiter [272], but this is not our experience. In the neck, the carotids will often be seen to throb violently; this condition may contribute to the anxiety of the patient.
The eye signs characteristic of Graves' disease often constitute the most striking feature (Fig. 10-4 above, Fig. 10-5, Fig. 10-6 below). Prominence of the eyes is the most important sign. A wild or staring expression is often observed. Lag of the lids behind the globes on downward rotation and lag of the globes behind the lids in upward rotation, infrequent blinking, failure to wrinkle the forehead on looking upward, and decreased ability to converge are also cardinal manifestations. Swelling of the lids is a characteristic and frequent eye sign. The bulbar conjunctiva may be edematous (chemosis). The insertions of the medial and lateral rectus muscles are often enlarged, inflamed, and quite obvious . The lacrimal gland can become infiltrated by lymphocytes and enlarged, and may protrude below the orbital boney margin.
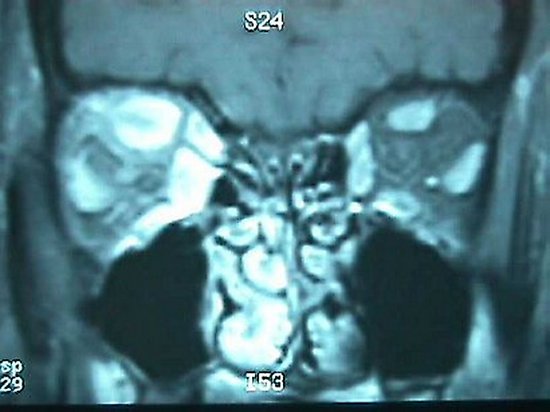
Figure 5a. This MRI image from a patient with Graves' ophthalmopathy provides a coronal view of the eyes. In this depiction the muscles appear white, and are enormously enlarged, especially in the left eye.
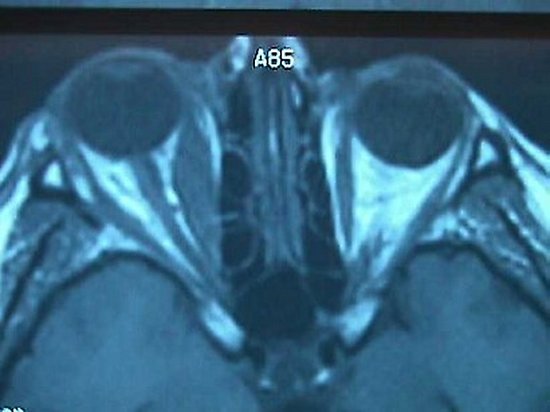
Figure 5b. In this transverse view the enlarged muscles are seen (appearing dark against the light fat signal) and the exophthalmos is apparent.
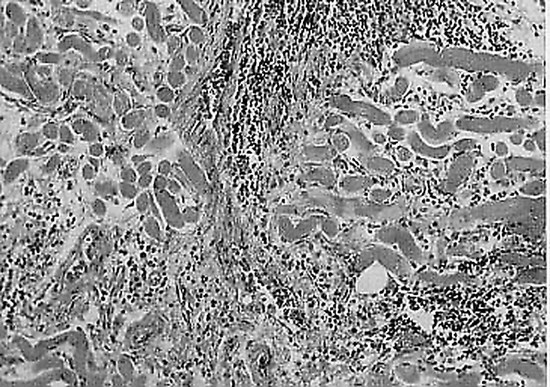
Figure 6a. Histologic appearance of extraocular muscle.
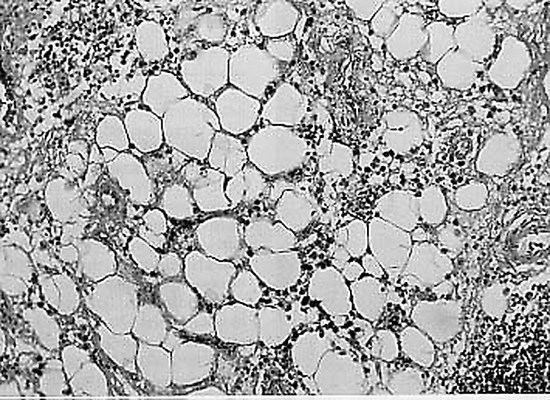
Figure 6b. Histologic appearance of retrobulbar fat.
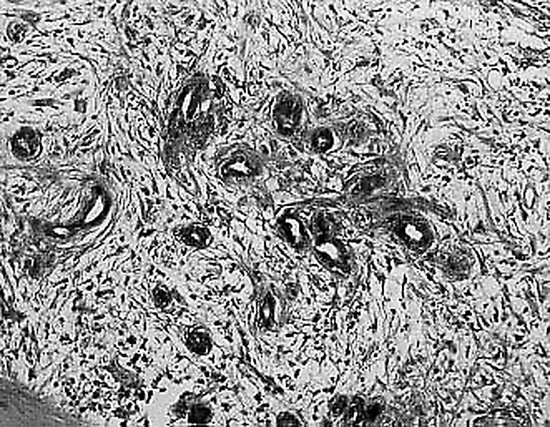
Figure 6c. Histologic appearance of lacrimal gland removed during a Kronlein procedure on a patient with severe exophthalmos.
For convenience, the ophthalmic phenomena may be grouped as in Table 10-4. A classification of the eye changes and a system of grading of their severity have been adopted by the American Thyroid Association [273] and is given in Chapter 12.
Table 4. Ocular Signs and Symptoms in Graves' Disease |
Ophthalmic phenomena reflecting thyrotoxicosis per se and apparently resulting from sympathetic overactivity: Lid reaction • Wide palpebral aperture (Dalrymple's sign) • Lid lag (von Graefe's sign) • Staring or frightened expression • Infrequent blinking (Stellwag's sign) • Absence of forehead wrinkling on upward gaze (Joffroy's sign)
Ophthalmic phenomena unique for Graves' disease and caused by specific pathologic changes in the orbit and its contents: • Inability to keep the eyeballs converged (Mobius' sign) • Limitation of movement of the eyeballs, especially upward • Diplopia • Blurred vision due to inadequate convergence and accommodation • Swelling of orbital contents and puffiness of the lids • Chemosis, corneal injection, or ulceration • Irritation of the eye or pain in the globe • Exophthalmos (also produces mechanically a wide palpebral fissure) • Visible and palpable enlargement of the lacrimal glands • Visible swelling of lateral rectus muscles as they insert into the globe, and injection of the overlying vessels • Decreased visual acuity due to papilledema, retinal edema, retinal hemorrhages, or optic nerve damage |
The eye signs may vary independently of the intensity of the thyrotoxicosis. Although it is true that in most patients with Graves' disease, eye signs, goiter, and symptoms of thyrotoxicosis appear more or less coincidentally, it is also true that in certain cases eye signs may appear long before thyrotoxicosis is evident, or become worse when the thyrotoxicosis is subsiding. Indeed, in some patients, serious exophthalmos may develop at a time when the thyrotoxicosis has been controlled by treatment.
The eye symptoms are extremely distressing. Diplopia is common; decreased visual acuity and other visual disturbances are less common. More frequent are symptoms due to conjunctival or corneal irritation. These symptoms include burning, photophobia, tearing, pain, and a gritty or sandy sensation.
Horner's syndrome on one side is occasionally encountered when the goiter has pressed upon the trunk of the cervical sympathetic chain. This syndrome consists of unilateral enophthalmos, ptosis of the lid, and miosis, as well as decreased sweating on the homolateral face.
THYROID GLAND
The thyroid may be smooth, lobulated, or rarely nodular. In thyrotoxicosis associated with nodular goiters, the hyperfunctioning tissue may reside between the nodules [274], which would constitute Graves' disease in a nodular goiter. Often the surface is lobulated, and the upper poles may seem to contain nodules above the site of entry of the superior thyroid artery. The diffuse toxic goiter is usually more or less symmetric. The size is related, but not closely, to the severity of the disease. It varies from the barely palpable normal (15 - 20 g) to an enlargement of six times normal (100 g) or, rarely, even more, but averages about 45 g. The near symmetry and usually moderate size of the diffuse goiter of Graves' disease make it somewhat less unsightly than many of the nodular goiters. It is commonly stated that the gland is not palpable in 1% of cases, either because the thyroid is actually smaller than ususal or because it is beneath the manubrium. However, in the presence of thyrotoxicosis, a small or normal-sized thyroid should alert the physician to the possiblity of some other cause of the illness.
The consistency of diffuse toxic glands is firm but elastic, or very firm if iodide has been given. The borders are easily demarcated by palpation. The pyramidal lobe should always be searched for since enlargement indicates the presence of diffuse disease of the thyroid. Also, if left behind at operation, it may be the site of recurrence of the disease.
Thrills and bruits are important but often absent. Their presence usually denotes hyperfunction. A thrill is less common than a bruit. It is more likely to be felt as a systolic purr in the region of the superior poles over the superior thyroid arteries. Bruits may be continuous or systolic in time, similar to a blowing cardiac murmur. Usually they are audible over the entire thyroid, often being louder on one side than on the other. Either a thrill or a bruit is highly suggestive, but not pathognomonic, of thyrotoxicosis. If local examination of a goiter discloses either of these signs, even though other evidence of hyperfunction may be lacking, especially careful investigation into the possibility of thyrotoxicosis is indicated. Both thrills and bruits tend to decrease in intensity as thyrotoxicosis subsides. They completely disappear in a few days under treatment with iodide.
The thrill is the palpable and the bruit the audible sign of turbulence associated with an increased rate of flow through rather tortuous vessels. The location of the thrill suggests that the larger thyroid vessels are chiefly responsible. Bruits may be distinguished from venous hums by occlusion of venous return caused by gentle pressure above the thyroid. A carotid or innominate thrill or bruit may be difficult to distinguish from sounds originating in the thyroid gland. Their localization over the vessel and distal transmission usually allow a distinction to be made.
Neighborhood symptoms, including dysphagia and the sensation of a lump in the neck, may be produced by toxic as well as nontoxic varieties of goiter. Sometimes the supraclavicular lymph nodes become enlarged and tender [275].
Vocal cord palsy is encountered, but is found chiefly in cancer of the thyroid, occasionally in nodular goiter, and only rarely in Graves' disease. Occasionally it is found on routine preoperative laryngoscopic examination, having produced no symptoms such as dysphonia or hoarseness.
The Skin
Cutaneous manifestations are nearly always present when hypermetabolism is significant. The patient feels hot and prefers a cold environment. Active sweating occurs under circumstances that would provoke no response in normal persons. Hand shaking gives a nearly diagnostic impression. The hand of the thyrotoxic person is erythematous, hot, and moist (sometimes actually dripping wet), in a state of hot hyperhydrosis. Although such hands may occasionally be found in other conditions, the finding of a cold hand, dry or moist -- almost excludes hyperfunction of the thyroid. Flushing is also very common, more in younger patients than in older ones. There may be more or less continuous erythema of the face and neck, with superimposed transient blushing. Occasionally diffuse pruritis or urticaria occurs. Urticaria appears to be linked to Graves hyperthyriodisim by some immune mechanism, but so far the causal relation is unknown (276).
The vasomotor system is overactive. Many of these cutaneous manifestations may be considered expressions of or incidental to increased heat elimination.
Redness of the elbows, first noted by Plummer, is frequently present. It is probably the result of the combination of increased activity, an exposed part, and a hyperirritable vasomotor system.
Although the integument is thinned, manifestations due to alteration in the growth of the tissue are less evident. It is possible that the type of fingernail described by Plummer (onycholysis) belongs in this category (Figure 10-7). The process may involve all fingers and toes, but typically begins on the fourth digit of each hand. The free margin of the nail leaves the nail bed, producing a concave or wavy margin at the line of contact. The hyponychium may be ragged and dirty, despite the best efforts at personal hygiene. Plummer's nails are a frequent and interesting clinical finding in Graves' disease. Occasionally the spoon-shaped fingernails of hypochromic anemia are encountered.
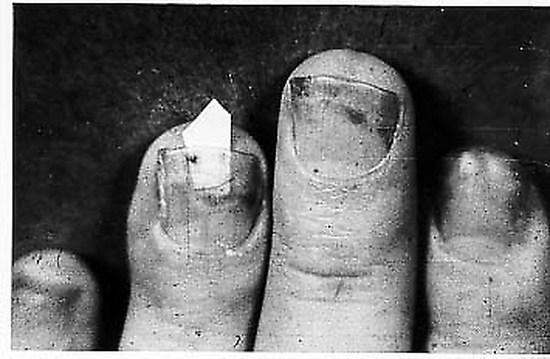
Figure 7. Plummer's nail changes, showing thinning of the nail and marked posterior erosion of the hyponychium.
Patchy hyperpigmentation, especially of the face and neck, is frequently seen, and occasionally there is a general increase in pigmentation. Most dark-skinned persons detect a definite increase in pigmentation during the onset of thyrotoxicosis, which may be dramatically localized around the eyes.
Patchy vitiligo is found in 7% of patients with Graves' disease, and we have observed several instances of complete loss of pigmentation in association with thyrotoxicosis. These changes are manifestations of associated autoimmunity directed toward melanocytes. The vitiligo, often of the hands and feet, may precede the onset of Graves' disease by years or even decades. Observation of this change is a useful clinical sign when attempting to establish the cause of thyrotoxicosis or exophthalmos.
Hair tends to be fine, soft, and straight. Women may complain that it will not retain a curl. (This complaint is also typical of patients with myxedema.) Temporary thinning of the hair is common, but alopecia is rare. Hair loss is often extreme after marked changes in metabolic rate are induced during therapy. We have seen complete or partial alopecia develop in a few patients with Graves' disease, sometimes in association with urticaria. These changes are believed to be manifestations of autoimmunity directed against the hair follicles.
Peripheral edema, unrelated to congestive heart failure or renal disease, is very common.
Pretibial myxedema (Figure 10-8) and the other remarkable abnormalities of "thyroid acropachy" are discussed in Chapter 12.
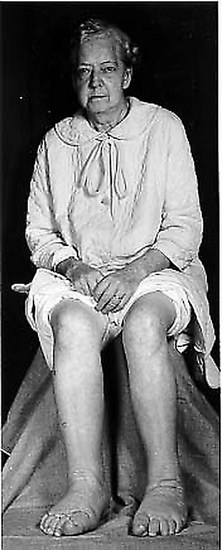
Figure 8. Remarkable "pretibial myxedema", also present on feet and hands, of a patient with Graves' disease and exophthalmos.
NEURAL AND MENTAL CHANGES
Neural and mental findings are varied and striking. The patient complains of nervousness or irritability and appears to be restless and fidgety. It sometimes seems impossible for the thyrotoxic patient to remain still for an instant. The tendon reflexes tend to be brisk, and the reflex relaxation time is shortened. The reaction to all sorts of stimuli is distinctly excessive. When asked to sit up, the patient jumps into an upright position. He or she may wish to cooperate but rather overdoes it. The patient is, so to speak, "hypercooperative." Such behavior constitutes an almost pathognomonic pattern. In the clinic, we are familiar with what we call the "thyrotoxic entrance and exit." The thyrotoxic patient hops into the clinic room like a jack-in-the-box, often with staring eyes, sits very quickly in the clinic chair, bolt upright, looks rapidly about the room, and does whatever is asked with pathologic alacrity. His or her exit is equally precipitate. Often emotional instability is combined with this pattern, perhaps to the point of a significant change in personality. The patient is often given to fits of crying, but may have sufficient insight to realize that the crying is pathologic. Some patients become hyperirritable and combative, and this can precipitate accidents or even assaultive behavior.
In some patients, the emotional pattern is that of hypomania or pathologic well-being (euphoria). In others, hyperactivity seems to produce a state of exhaustion, and profound fatigue or asthenia chiefly characterizes the picture. The mind is often very active, and the patient is troubled with insomnia. Rarely, patients develop visual or auditory hallucinations or a frank psychosis. The latter may not completely clear up after thyrotoxicosis has been treated. It is probable that thyrotoxicosis makes manifest an abnormality already present rather than inducing a psychosis de novo. Brownlie et al reported 18 cases of patients with thyrotoxicosis who had coincident psychotic disorder and concluded that, usually this was an affective psychosis, and that the incidence was above chance co-occurrence. Thyrotoxicosis appeared to be a precipitant effect of psychosis [277].
Impairment of intellectual function has been found in patients with untreated hyperthyroidism. It is usually assumed that such abnormalities return to normal with therapy. However, Perild et al. [166]report that ten years after successful therapy of thyrotoxicosis a group of patients manifested abnormal neuropsychological tests, and half had significant intellectual impairment which was apparently permanent. This surprising observation awaits confirmation. Marked increase in fatigability, or asthenia, is often prominent. This increased weariness may be combined with hyperactivity. Patients remark that they are impelled to incessant activity, which, however, causes great fatigue.
A fine, rapid tremor of the outstretched fingers is classically found, and a generalized tremulousness, involving also the tongue, may be evident. Muscle fibrillations are not a usual part of the syndrome, but they may occur in chronic thyrotoxic myopathy. Polyneuropathy has also been reported. [279]
More severe neurologic problems also occur during Graves' disease ( Table 10-5). Patients who are known to have a convulsive disorder may become more difficult to control with the usual medications, and seizures may appear in patients who have never previously manifested such symptoms [280]. Electroencephalography [280] reveals increased fast wave activity, and occasionally bursts of tall spike waves. Several reports describe a severe steroid responsive encephalopathy (Hasshimoto’s Encephalopathy) in patients with Hashimoto's thyroiditis ( 281). The same syndrome has been described in Graves' Disease [282]. A direct relation to Graves, or thyroiditis, seems probable, but is un-proven. In animals, excess T4 decreases the threshold to convulsive stimuli [169].
Table 5. Neuromuscular Manifestations of Thyrotoxicosis |
Tremor • Hyperactive reflexes • Accelerated reflex relaxation • Anxiety • Disorientation • Psychosis • Thyrotoxic neuropathy (rare) • Acute thyrotoxic encephalopathy ( rare) • Seizures (with or without an underlying abnormality) • Neuropathy secondary to nerve entrapment by lesions of pretibial myxedema • Corticospinal tract disease with pyramidal tract damage (rare) • Chorea and athetoid movements (rare) • Hypokalemic periodic paralysis • Myopathy • (Myasthenia gravis -- associated)
|
C.H., 52-Year Old Woman: Psychosis with Thyrotoxicosis
This woman appeared in the emergency room in a confused and agitated state. She refused to talk, but would on occasion answer questions. She appeared to be extremely paranoid and was resistant to offers of help.
She came to the emergency room alone, and after one interview disappeared. She returned a few hours later, again in the same agitated, confused, paranoid, and semimute condition. She stated that she heard voices quoting the Scripture and denied that these voices directed her to harm herself or others, but indicated that she was responsible for the bad problems of the world.
Relatives were contacted and indicated that the patient had been entirely well up to the previous few days, when she had become confused and agitated. It was determined that the patient had worked for more than 20 years and had lost her position about four years previously. She was married and had been separated from her husband intermittently during the past four years. She knew her address, was aware of the month and year but not the date, and was confused about current events.
The BP was 150/80 and the pulse rate 140. The patient was disheveled, thin, and hyperactive. The eyes were normal. Results of routine blood chemistry tests, complete blood count, and urinalysis were negative.
The patient was treated initially with haloperidol (Haldol), 1 mg twice a day, and gradually calmed. The diagnosis of hyperthyroidism was considered and confirmed by an FTI of 19. Antithyroid antibodies were absent.
During treatment, the patient's paranoia and anxiety subsided. She subsequently indicated that there had been a gradual increase in tiredness and weakness, weight loss of 10 lbs, heat intolerance, palpitations, and tremor over one to two years. Previous medical problems included a hysterectomy for fibroids and mild hypertension treated by diuretics. There was no history of previous psychiatric illness in the patient or her family.
On further examination, the thyroid was seen to be enlarged to about 35 g and was diffusely increased in size, without nodules; there was no bruit. Propranolol was added to the therapy, and Haldol was continued. The patient rapidly became psychologically normal and entirely cooperative, and regained control of her personal affairs.
An RAIU test was 49.7%. The patient received 4 mCi of radioactive 131I. After radioactive iodine therapy, the patient was given an antithyroid drug that brought her thyroid hormone levels back to normal. When this drug was discontinued, her FTI returned to 15.7. She was given 3.4 mCi of radioactive iodine again, and PTU was restarted. When last examined, her FTI was in the normal range.
There has been no return of any abnormal psychologic function, and the patient has received no further psychiatric care.
This episode appeared to be an acute psychotic reaction associated with severe hyperthyroidism, occurring in a patient with no previously known psychologic disease. It cleared promptly with medical therapy, including treatment of the hyperthyroidism, and the patient is now apparently well.
C.J., 43-Year-Old Woman: Thyrotoxic Neuropathy
This woman was referred for evaluation with a history of obesity, hypertension for two years, prominence of the right eye for two years, and thyroid overactivity known for six months. She had gained 50 lbs during the interval preceding the examination because of excess eating. Increasing dyspnea and shortness of breath, present for the previous two years, had become worse in the previous two months. She came to the emergency room because of symptoms of asthma. Examination revealed a pulse rate of 120 and an enlarged heart. There was LVH and strain on the electrocardiogram, and on echocardiogram an enlarged left atrium and a left ventricle with decreased function, especially of the lateral and posterior inferior walls. Thyroid function tests showed a T4 level of 17 ug/dl, an FTI of 16.8, and a T3 level of 357 ng/dl. She received digoxin, 0.25 mg daily, furosemide, 40 mg daily, potassium chloride, and aminophylline.
On examination in the endocrine clinic, the BP was 170/100, and the patient was obese and hyperactive. There was moderate bilateral proptosis and inflamed insertions of the extraocular muscles. There was 22 mm proptosis bilaterally. The thyroid was diffusely enlarged to about 40 g. Neurologic examination showed weakness of ocular motility with diplopia on the left lateral gaze, bilateral nystagmus, marked proximal muscle weakness without fasciculations, and decreased touch, pinprick, and vibration sense in a glove distribution of both arms, the left greater than the right. There was no significant deficit in the feet. Weakness in the left upper extremity was marked. Deep tendon reflexes were absent. The differential diagnosis included Graves' disease, cardiomyopathy and peripheral neuropathy, congestive heart failure, and hypertension.
A neurologic consultant confirmed the neuropathy and noted mild choretic movements of the left hand and arm. Other known causes of neuropathy were excluded. The patient was treated for one month with antithyroid drugs and then given 2.7 mCi 131I. Because of continued hyperthyroidism, the patient was retreated with 3.2 mCi 131I seven months after the initial treatment. Three months later the FTI was normal at 10.4, and there were no symptoms or signs of congestive heart failure. Some decreased strength and clumsiness of the left hand persisted. The diplopia and proptosis were unchanged. The neuropathy in the hands had decreased, and the patient was euthyroid.
This patient exhibited profound cardiomyopathy and skeletal myopathy, choreiform movements, and peripheral neuropathy, all apparently related to severe thyrotoxicosis. She improved rapidly with appropriate treatment of the thyrotoxicosis.
The tremor of Parkinsonism is greatly intensified during thyrotoxicosis. Signs and symptoms of cerebellar disease or pyramidal tract lesions have been seen [170,171]. Rarely, patients manifest extreme restlessness, disorientation, aphasia, grimacing, chorioathetoid movements, symptoms suggestive of encephalitis [286], or episodes of hemiparesis or bulbar paralysis. These symptoms clear up completely after restoration of metabolism to normal. No definite lesions have been found in the brain. Rarely, polyneuropathy has been severe enough to cause paraplegia [287].
Most of the biochemical actions of thyroid hormone on the brain are related to developmental functions rather than function in the adult. These actions have recently been reviewed by Bernal. All three forms of thyroid hormone receptor are expressed in the brain, especially in neurons. Genes regulated by thyroid hormone include myelin basic protein, mitochondrial genes such as cytochrome C oxidase, neurotrophins and their receptors, including NGF and trkA, cytoskeletal components such as tubulin, transcription factors such as NGF1a, extracellular matrix proteins, and adhesion molecules such as NCAM, genes involved in intracellular signaling such as RC3/neurogranin, and genes expressed in the cerebellum such as pcp-2. Interestingly, the brain of a thyrotoxic human subject does not have an elevated consumption of oxygen. Sensenbach et al. [174] found the cerebral blood flow to be increased, the cerebral vascular resistance decreased, arteriovenous (AV) oxygen difference decreased, and oxygen consumption unchanged in thyrotoxicosis. Reciprocal changes occurred in myxedema, and all reverted to normal after therapy. Curiously, brain size was shown to decrease significantly during treatment of the hyperthyroid patients, and ventricular size increased. This remarkable change is of uncertain cause but may involve osmotic regulation.
Although it is possible that some of the central nervous system irritability is a manifestation of elevated sensitivity to circulating epinephrine, this contention has not been proved. Epinephrine levels and catecholamine excretion are actually not elevated, but propranolol, presumably acting by inhibition of alpha-adrenergic sympathetic activity, certainly reduces anxiety and tremulousness in a very useful manner. The clinical applications of these findings are discussed in Chapters 11and 12.
MUSCLES
The muscular symptoms vary from mild myasthenia to profound muscular weakness and atrophy, especially of proximal muscle groups. This weakness forms the basis of a useful clinical test. If a thyrotoxic patient seated in a chair is asked to hold one leg out straight and in a horizontal position, he or she may be able to do so for 25 - 30 seconds only; normal persons can maintain such a position for 60 - 120 seconds. Toe standing and step climbing may also bring out muscle weakness that is otherwise not so apparent. In the more extreme forms of muscular involvement, there is not only weakness but also atrophy. Wasting of the temporals and interossei may be noted in a considerable number of patients, and in a few, wasting of all skeletal muscles. This wasting may go so far as to bear a close resemblance to progressive muscular atrophy; occasionally the myopathy may shade into the picture of a polymyositis. Muscle cell necrosis and lymphocyte infiltration may be visible histologically, but usually are not found even when the symptoms of weakness are severe [175]. Tremor, which is usually present, is ascribed to altered neural function. Fasciculations are unusual.
The speed of both tension development [290] and relaxation of the muscles is increased, so that the reflex time is shortened. The electromyogram is normal in most instances but may occasionally resemble that of muscular dystrophy [291]. Work efficiency, measured in terms of the calories of heat produced while performing a given amount of work, has been reported to be both decreased [292] and normal. The question of metabolic efficiency of hyperthyroid muscle has been revisited by Erkintalo et al, using phosphorus-31 MRI spectroscopy, finding that toxic muscle required more energy to function than normal, presumably because of additional ATP-consuming mechanisms [293]. Creatine excretion is increased. The muscles have decreased ability to take up creatine, produced in the liver, from the blood [294-295]. Creatinine excretion is initially increased by the general catabolism of hyperthyroidism, but as muscle mass diminishes, creatinine excretion in the urine is depressed.
Myasthenia gravis may simulate thyrotoxicosis, and vice versa [296]. It has been reported that neostigmine both strengthens the muscles in thyrotoxic myopathies and is without effect. Certainly, the response is small in comparison with the immediate and striking correction of weakness seen in myasthenia gravis. Thyrotoxicosis may rarely ameliorate myasthenia gravis, but typically it is accentuated by thyrotoxicosis and is also worsened by myxedema. The close relationship between these two diseases is apparent in the observation that thyrotoxicosis occurs in 3% of patients with myasthenia gravis. The pathogenic anti-acetylcholine receptor antibodies that occur in myasthenia gravis are clearly comparable to the anti-TSH receptor antibodies found in Graves' disease. In addition, it has been found that TG and acetyl- cholinesterase share epitopes recognized by B cells. It is, however, uncertain that this plays any role in the pathogenesis of muscle disease in Graves' patients.
Periodic paralysis is precipitated and worsened by thyrotoxicosis [297]. This relationship has been extensively studied in Japan, where it is a familiar syndrome, particularly in men. The paralysis is usually associated with and due to hypokalemia. While the exact mechanism is not known, the hypokalemia is believed to be caused by a shift to the intracellular compartment. It has been demonstrated that thyrotoxicosis augments K+ uptake and release from cells. Experimental T4 treatment augments synthesis of membrane Na+-K+ activated ATPase. The episodes of paralysis tend to be infrequent and sporadic, but most commonly occur after a meal, following exercise, or start during sleep, and can be induced by administration of glucose and insulin. The onset following meals or exercise presumably relates to rapid K+ uptake by cells. Episodes last from minutes to hours, usually involving peripheral muscles, but can cause paralysis of the diaphragm and affect the heart. Serious episodes can be associated with extensive muscle cell damage and necrosis, EKG abnormalities such as ST and T wave changes, PVCs, first degree heart block, prolonged Q, T intervals, and even ventricular fibrillation [298].
Potassium treatment has some protective effect, and quickens recovery from attacks. Propranolol, for reasons not entirely clear, has prophylactic action. Therapy of the thyrotoxicosis almost always causes the rapid and permanent disappearance of the syndrome.
Myotonia congenita and myotonia dystrophic do not occur with increased frequency with thyrotoxicosis.
SKELETON AND CALCIUM METABOLISM
Roentgenographic examination of the bones frequently discloses evidence of decalcification. Microdensitometry demonstrates this condition at all ages and in both sexes [299-301]. Patients with even mild increases in thyroid hormone lose bone mass [302], especially if postmenopausal and not receiving estrogen therapy. Those with a history of thyrotoxicosis extending over a number of years may have osteoporosis that is severe and premature9303-304. Fractures are uncommon, with the most frequent being collapsed vertebra in a chronically thyrotoxic postmenopausal woman. Skeletal mass is augmented after therapy [300-301]. Treatment restores the density in younger patients, but not usually in the elderly [300]. Although most attention has been made to the effects of thyroid hormone on bone density in women, it is not surprising to know that thyroid hormone excess also has a mild deleterious effect in males (303). A meta analysis of 289 published studies on the effect of hyperthyroidism causing bone fragility found that hyperthyroid patients had decreased bone mineral density and increased fracture risk. The bone mineral density tended to return to normal after therapy (302).
Periarthritis of the shoulder (subacromial bursitis) is occasionally associated with thyrotoxicosis. Linear bone growth may be accelerated in children. The time of epiphyseal closure may be accelerated in children, and bone age may exceed chronologic age.
Thyrotoxicosis results in an accelerated turnover of bone calcium and collagen [305-306]. TRα1, TRβ1, and TRβ2 proteins are expressed in human osteoblast cells and strongly in human bone marrow stromal cells. Endogenous receptors in these cells are functional in in vitro test systems. The specific function in vivo is unclear. As described in the section on pathology, the histologic picture of bones from the thyrotoxic patient may suggest osteitis fibrosa with increased osteoclastic activity, fibrosis, and an increased number of osteoblasts [267]. Histomorphometric evaluations with tetracycline labelling demonstrates accelerated bone resorption and formation, both in spontaneous hyperthyroidism and in women treated with excess thyroid hormone [266, 306,305]. In bone biopsy specimens the thin trabeculae of osteoporosis are seen. [267]The serum calcium level is usually normal, but may be elevated sufficiently to produce nausea and vomiting [307]and, rarely, renal damage [308, 309]. It may be made clinically evident when thyrotoxic patients become relatively immobile, for example at bed rest during illness. In contrast to what occurs in hyperparathyroidism, the hypercalcemia can usually be corrected partially or totally by the administration of glucocorticoids [310], but these have not been effective in all cases [311]. Phosphorus administration also lowers the concentration of calcium in serum and urine to normal [312]. The exchangeable calcium pool is remarkably increased [305]. Serum osteocalcin is increased in parallel with hormone levels [313]. The alkaline phosphatase level may be elevated, with a pattern showing the normal equal distribution of bone and liver isoenzymes. The changes in calcium and alkaline phosphatase correlate with serum T3 levels [314]. After therapy, the alkaline phosphatase level tends to increase, and bone isoenzyme becomes predominant, probably due to skeletal repair [314].
Fecal and urinary calcium excretion is greatly augmented, and it is remarkable that renal stones are rarely formed. This is because there is a concomitant increase in excretion of colloids that stabilize the calcium. Urinary hydroxyproline and pyridium cross-link excretion are increased and fall to normal after therapy [306]. Serum carboxy-terminal-1-telopeptide and serum osteocalcin levels and urinary osteocalcin secretion are increased and return to normal with therapy[268,313,315].
The serum phosphorus level is in the normal range or depressed. Renal phosphorus resorption is in the normal range or elevated [309, 310]. Although some of the observations suggest the presence of hyperparathyroidism, it is most likely that the changes actually reflect the direct metabolic effects of thyroid hormone. The parathyroid glands are histologically normal. In fact, parathyroid hormone (PTH) levels tend to be suppressed in hyperthyroidism, apparently in response to the elevated calcium levels [201]; 1,25-dihydroxyvitamin D3 levels are likewise about 40% below normal [202].
The increased fractional tubular phosphate reabsorption characteristic of hypoparathyroidism may also occur in thyrotoxicosis, probably because of reduced PTH levels. In one reported study [308], urinary phosphorus excretion was depressed after calcium infusion. Thus, a normal response was obtained rather than that found in hyperparathyroidism.
The hypercalcemia appears to be a direct manifestation of thyroid hormone action on bone metabolism [316,317], and calcium absorption from the intestine is usually reduced. [318]Both catabolism and anabolism of bone are accelerated. Negative calcium balance can sometimes be corrected by administration of calcium, an observation that perhaps should be given more attention in the management of thyrotoxic patients. Hypercalcemia can be corrected by propranolol therapy in some patients [319]. Bone turnover can be reduced by pamidronate and by calcitonin, which may therefore have a useful role in reducing thyrotoxicosis-induced osteopenia [320-321].
Two exceptional cases have been reported with coincident thyrotoxicosis and hypercalcemia with elevated PTH levels. Treatment of thyrotoxicosis eliminated all abnormalities, for reasons unknown. [322].
RESPIRATORY SYSTEM
Except for dyspnea, which may or may not represent abnormal respiratory function, symptoms deriving from the lungs are not prominent. Nevertheless, measurements show some reduction in vital capacity, expiratory reserve volume, pulmonary compliance, airway resistance, and weakness in both expiratory and inspiratory muscles [323,324]. Minute volume response to exercise is excessive for the amount of oxygen consumed [325]. Dyspnea on effort is present in a large majority of the patients. Pulmonary function in patients without coincident congestive heart failure has demonstrated reduction in vital capacity, decreased pulmonary compliance, weakness of the respiratory muscles, increase in respiratory dead space ventilation, and normal diffusion capacity. A combination of the four abnormalities may produce dyspnea [326]. Amelioration of intractable bronchial asthma has been reported after treatment of coincident thyrotoxicosis [209].
CIRCULATORY SYSTEM
(Table 10-6)
First and foremost of the symptoms deriving from the circulatory system are palpitations and tachycardia. The heart may beat with extreme violence, which may be distressing to the patient, particularly at night or on exercise. The pulse on palpation is rapid and bounding. The systolic blood pressure is frequently elevated. The diastolic blood pressure is characteristically decreased, and the pulse pressure is elevated, being usually between 50 and 80 mm Hg.
Left ventricular hypertrophy may be suggested on physical examination. A bounding precordium is so typically found that its absence is a point against the diagnosis of hyperthyroidism. However, in the majority of instances, roentgenograms show the heart to be normal in transverse diameter. A systolic murmur is usually heard over the precordium. One reason for this murmur is the development of mitral valve prolapse during thyrotoxicosis. [328]This can be detected by angiography, or more easily by echography. It is postulated that papillary muscle dysfunction due to inadequate ATP supplies may be responsible for the lesion. Prolapse is usually not clinically evident but rarely is a cause of symptomatic mitral valve insufficiency. The prolapse can revert to normal with therapy [328].
Interpretation of physical signs, especially systolic murmur and gallop rhythm, in the heart is difficult and uncertain in the presence of thyrotoxicosis. In evaluating heart status in thyrotoxicosis, one should concentrate chiefly on the presence or absence of signs of failure rather than on physical signs in the heart itself. If no signs of failure are present, the best procedure regarding abnormal cardiac findings is to ascertain whether they persist after thyrotoxicosis has been abolished.
A grating pulmonic systolic sound ("Lerman Scratch"), which has some of the characteristics of a pericardial friction rub, is occasionally heard over the sternum in the second left interspace. It is heard best at the end of full expiration. Its intensity subsides as thyrotoxicosis improves. The diagnosis of pericarditis may be suggested on the basis of this sound. The fact that it is superficial and tends to disappear on inspiration suggests a pleuropericardial origin. It may be related to the dilated pulmonary conus often seen on x-ray films in thyrotoxic patients. The sign has no prognostic significance.
Extrasystoles are frequent, and paroxysmal atrial tachycardia and atrial fibrillation, paroxysmal or continuous, occur in 6 - 12% of patients. Even subclinical hyperthyroidism is associated with a fivefold greater chance of developing atrial fibrillation, and it is effectively the same as the situation with overt hyperthyroidism (329). Precordial pain that seems distinct from angina pectoris occurs occasionally. Cardiac enlargement and congestive heart failure may occur with or without prior heart disease [331, 332]. (Figure 10-9). The electrocardiographic manifestations are confined to tachycardia, increased voltage, and sometimes a prolongation of the PR interval [332], unless there is a dysrhythmia or an accompanying but unrelated disorder of the heart.
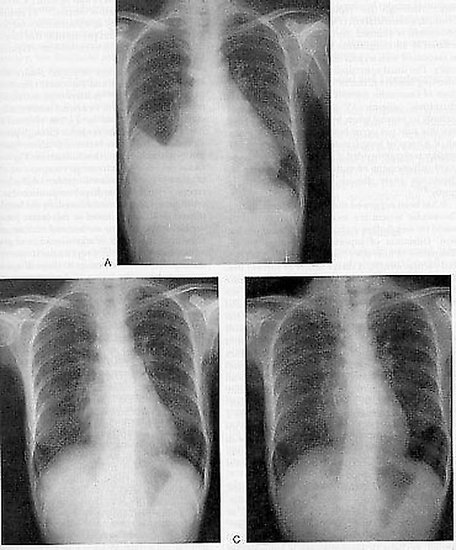
Figure 9. Congestive heart failure induced in an otherwise healthy young woman (a), which receded (b), and returned to normal (c), during and after therapy.
Patients with coronary atherosclerosis often develop angina during thyrotoxicosis. Occasionally angina develops de novo in young women with arteriography-proven normal coronary arteries. This condition has been ascribed to an imbalance between increased cardiac work and blood supply, so that a functionally deficient blood supply occurs even with a patent vessel [333]. Severe coronary vasospasm has been observed during angiography in patients with GD (334). Myocardial damage occurs in toxic patients with CHF (335) and myocardial infarction can occur in toxic patients with normal coronary vessels [336].
In thyrotoxicosis the heart rate, stroke volume, and cardiac output are increased. Circulation time is decreased. There is dilatation of superficial capillaries. Coronary blood flow and myocardial oxygen consumption in each stroke are increased [337]. Circulating plasma volume is increased. AV oxygen differences are variable but tend to be normal. Cardiac output in response to exercise is excessive in relation to oxygen consumed.
The relation of cardiac systolic time intervals to thyroid function has provided a valuable in vivo bioassay of hormone action. The pre-ejection period is shortened in thyrotoxicosis, and the left ventricular ejection time remains relatively normal. The interval from initiation of the QRS complex to arrival of the arterial pulse in the brachial artery is reduced [338]. Cardiac diastolic function as evaluated by echocardiography remains normal in the majority of patients [339].
Congestive heart failure and atrial fibrillation, when due to or associated with thyrotoxicosis, are relatively resistant to the action of digoxin. Accelerated metabolism of digoxin, plus the cardiac inefficiency and irritability produced by thyrotoxicosis, may be at least two of the factors producing this resistance. Although the response to the drug will be blunted, a beneficial effect will occur if a proper level of digoxin is attained.
Atrial fibrillation should be treated by anticoagulation if it is persistent, since it is associated with serious embolism in 10% of cases. The usual contraindications of old age, HBP, bleeding tendency, recent CVA, etc. apply, and the dose of Coumadin needed is lower than normal in thyrotoxic patients. AF tends to revert spontaneously to normal when hyperthyroidism is cured, but this may not occur before six months, or not at all, if AF was of long standing. Therapeutic cardio- version is recommended if AF persists six months beyond achievement of euthyroidism. Long term follow-up studies have revealed increased mortality from cardiovascular and cerebral vascular disease in patients with a past history of overt hyperthyroidism treated with radioiodine, and in patients with subclinical hyperthyroidism. Possibly development of atrial fibrillation and other supraventricular dysrhythmias may account for increased vascular mortality (340). Treatment of thyrotoxic heart disease has been reviewed recently [341]. A review of the impact of hyperthyroidism on cardiac function in older patients is available [342].
It has been suggested that the changes in the cardiovascular system are secondary to increased demand for metabolites and to increased heat production. Dilatation of superficial capillaries for the dissipation of heat does cause increased blood flow and cardiac output. However, a direct action of thyroid hormone on the heart is also increased, since the sinus node has higher intrinsic activity, the isolated thyrotoxic heart beats faster than normal, and isolated papillary muscle from a thyrotoxic heart has a shortened contraction time [343-345]. The heart shares in the general increase in respiratory quotient found in skeletal muscle. Adenosine transport into myocardial cells and its phosphorylation are increased [345]. Excess thyroid hormone increases cardiac Na+-K+ activated membrane ATPase, and sarcoplasmic reticulum Ca++-activated ATPase, both of which contribute to the heightened contractility of cardiac muscle. In addition excess thyroid hormone, at least in experimental animals, causes, by a direct effect on DNA transcription rates, an increased synthesis of alpha-myosin heavy chain with high ATPase activity, and decrease of beta myosin heavy chain synthesis. This alteration in alpha/beta ratio is associated with increased contractility [346]. In addition to the gene-mediated effects of thyroid hormone on the heart, triiodothyronine has direct effects that cause lower systemic vascular resistance and a higher cardiac output (347)
Whether an autoimmune process is involved in low output cardiac dysfunction in patients with Graves’ disease was investigated by myocardial biopsy of eleven patients in a study by Fatourechi and Edwards. Two of the group had lymphocytic infiltrates suggestive of an autoimmune process, whereas the others did not, indicating that this process may occur but would be an unusual cause of cardiac dysfunction [348].
Table 6. Cardiac Manifestation of Graves' Disease |
• Tachycardia • LVH and strain on EKG • Premature atrial and ventricular contractions • Atrial fibrillation • Congestive heart failure • Angina with (or without) coronary artery disease • Myocardial infarction • Systemic embolization • Death from cardiovascular collapse • Resistance to some drug effects (digoxin, coumadin) • Residual cardiomegaly |
The cardiovascular changes may be due in part to increased sensitivity to circulating epinephrine. Thyroid hormone administration may increase, or not alter, the catecholamine content of the heart. Guanethidine partially restores cardiac dynamics to normal, perhaps by releasing catecholamine from the heart and thus reducing the cardiac stimulation caused by this agent. Guanethidine and beta-adrenergic blockers slow the tachycardia of thyrotoxicosis. Concomitant with this slowing is an increase in stroke volume, and there may be either a decrease [223] or little change in cardiac output [350]. T4 can increase the heart rate directly in a manner not mediated by catecholamines [351], and presumably this direct chromatotropic effect adds to coincident sympathetic effects on the heart. Thyrotoxicosis causes increased beta-adrenergic receptors in the heart and increased responsiveness to isoproterenol [352, 353]. Alpha-adrenergic and cholinergic receptors are reduced. In animal studies, adenyl cyclase may be activated by T3, but reduced cardiac levels of ATP limit the response through protein kinase activation. The net effect is beta-adrenergic sensitization and cholinergic desensitization. Thyrotoxicosis also increases beta-adrenergic receptors on a variety of tissues [355]. An increased number of -adrenergic receptors could cause hyper-responsiveness to adrenergic agonists, and could mediate the heightened plasma cAMP levels noted in thyrotoxic patients in the basal state (in some studies) and after assumption of an upright posture or administration of glucagon and epinephrine [356, 356]. Propranolol treatment normalizes the cAMP responses to these drugs and, of course, inhibits the action of beta-adrenergic agonists on the heart. Possibly these actions of propranolol explain its ability to reduce somewhat the consumption of oxygen in thyrotoxicosis.
The impact of the interrelation of excess thyroid hormone and the sympathetic nervous system in humans is not finally settled [357]. There is clear evidence for increased beta- adrenergic receptors in the heart and elsewhere in thyrotoxicosis, as inferred from animal studies. Responses of the thyrotoxic human to adrenergic agonists are probably not excessive in relation to responses in normal subjects, although this question is much debated. Beta-adrenergic blockade clearly reduces some pathophysiologic responses, including the increased nitrogen and oxygen consumption, toward but not to normal. Metabolism of the heart and other organs is stimulated directly by thyroid hormone, and sympathetic effects are additive. It remains possible that the sympathetic responses are in fact exaggerated; it is also clear that sympathetic responses do not "mediate" thyroid hormone action. Left ventricular reserve is impaired in thyrotoxicosis, and beta adrenergic blockage can lead to increased pulmonary artery pressure in some circumstances and further impair cardiac function (358). This suggests caution in administering beta-blockers to patients with severe hyperthyroidism and any evidence of circulatory dysfunction. We have seen administration of propranolol to patients with severe hyperthyroidism on rare instances to cause cardiovacular collapse and shock.
While this discussion has concentrated on the cardiovascular effects of thyrotoxicosis, it is worth remembering that long-term mild excess of thyroid hormone causes impaired cardiac reserve and exercise capacity [358]. Subclinical thyrotoxicosis can alter cardiac function, with increased heart rate, increased left ventricular mass index, increased cardiac contractility, diastolic dysfunction, and induction of ectopic atrial beats or arrhythmias (359). Some of these changes are reversible when euthyroidism is restored.
Smit et al studied 25 patients with a history of differentiated thyroid carcinoma with more than 10 yr of TSH suppressive therapy with L-T4. Medication was titrated in a single-blinded fashion to establish continuation of TSH suppression (low-TSH group) or euthyroidism (euthyroid group). At baseline, diastolic function was impaired in all lopr-TSH patients as indicated by abnormal values for the peak flow of the early filling phase (E, 55.3 +/- 9.5 mm/sec), the ratio of E and the peak flow of the atrial filling phase (E/A ratio, 0.87 +/- 0.13), the early diastolic velocity obtained by tissue Doppler (E', 5.7 +/- 1.3 cm/sec), and the peak atrial filling velocity obtained by tissue Doppler (A', 6.8 +/- 1.4 cm/sec), prolonged E deceleration time (234 +/- 34 msec), and isovolumetric relaxation time (121 +/- 15 msec). After 6 months, significant improvements were observed in the euthyroid group in the E/A ratio (+41%; P < 0.001), E deceleration time (-18%; P = 0.006), isovolumetric relaxation time (-25%; P < 0.001), E' (+31%; P < 0.001), and the E'/A' ratio (+40%; P < 0.001). Prolonged subclinical hyperthyroidism is accompanied by diastolic dysfunction that is at least partly reversible after restoration of euthyroidism. Because isolated diastolic dysfunction may be associated with increased mortality, this finding is of clinical significance (359).
HEMATOLOGIC CHANGES
In most patients the hemoglobin and hematocrit are in the normal or low range [360]. Blood volume is increased, and the red cell mass is actually increased in some patients. In severe thyrotoxicosis normocytic anemia with hemoglobin concentrations as low as 8 - 9 g/dl may be observed. Hyperthyroid patients with anemia may show impaired iron use [361, 362]. Malnutrition may play a role in this decrease. These anemias are unresponsive to hematinic therapy, but the blood picture returns to normal when the thyrotoxicosis is controlled [262]. Iron deficiency or megaloblastic anemia is exceptional and requires a search for some explanation other than thyrotoxicosis. It is possible that thyrotoxicosis may increase the need for vitamin B12, as shown experimentally, and perhaps for folic acid. Also, there is an increased incidence of antigastric antibodies and mild pernicious anemia in patients with Graves' disease.The glucose-6-phosphate dehydrogenase activity of red cells is increased in thyrotoxicosis [363].
A relative lymphocytosis is frequently found in the peripheral blood due to neutropenia [364]. A relative and an absolute increase in the number of monocytes was noted years ago [365]. The monocyte count was between 10 and 15%; in only 2 of the 30 cases was it less than 10%. Relative lymphocytosis and relative monocytosis, with a normal or slightly low total white cell count, constitute the characteristic blood findings of Graves' disease. There is also an increase in the percentage and number of B lymphocytes and, as discussed previously, an altered ratio of T lymphocyte subsets. Significant pancytopenia with leukocyte counts under 3x109/l and neutrophiles under 2x109/l occasionally occurs, and if unrelated to drug therapy, tend to recover during treatment (366).
Graves' disease is often associated with mild thrombocytopenia, and occasionally with idiopathic thrombocytopenic purpura [367]. This co-occurrence is thought to reflect the autoimmune pathogenesis of both diseases. Fourteen percent of patients with ITP are reported to have coincident Graves' disease. Mild thrombocytopenia may disappear spontaneously or with treatment of hyperthyroidism, or if severe, may respond to glucocorticoid therapy [368]. Other more severe cases are managed as typical cases of ITP. Bone marrow examination may show normal or increased megakaryocytes [368]. Hyperthyroidism also induces a shortened platelet life span, believed to be due to more rapid clearing of normal platelets by an activated reticulo-endothelial system. Both anti-platelet antibodies and shortened platelet life span could contribute to the low or low-normal levels of platelets found in Graves' patients. It is reported that all patients with Graves' disease have evidence of IgG bound to platelets.
Coagulation is usually normal in spite of mild prolongation of the prothrombin time. Antihemophilic factor is often elevated in level and returns to normal with treatment. Hyperthyroidism can be associated with increased coagulability, in part through elevation of factor VIII. Cerebral venous thrombosis has been reported in association with thyrotoxicosis, suggesting that occasionally the propensity for coagulation can lead to serious consequences(369). Capillary fragility is increased. Severe liver damage caused by thyrotoxicosis and secondary congestive heart failure may be associated with a hemorrhagic tendency.
RETICULOENDOTHELIAL AND LYMPHATIC SYSTEMS
The reticuloendothelial and lymphocytic systems undergo hyperplasia. There may be generalized lymphadenopathy, and the thymus may be enlarged. Occasionally the thymus presents as an anterior mediastinal mass, but diminishes to normal size with control of the thyrotoxicosis [370]. Some authors have reported that the spleen tip can be felt in 20% of patients, but in our experience this finding is not common. The autoimmune responses in these patients have been detailed above. Several markers of augmented immune activity are elevated, including soluble CD30, a molecule released by T helper 2 cells, and IL-6 [371].
GASTROINTESTINAL FINDINGS
The appetite is characteristically increased. The effect of this increase is to offset, in part (sometimes completely), the loss of weight that might be expected from the increased catabolism. Indeed, the pattern of weight loss with increased appetite is nearly pathognomonic of thyrotoxicosis, although it may occur in diabetes mellitus and malabsorption or intestinal parasitism. A minority of patients complain of anorexia. Needless to say, they are likely to show the greatest weight loss. Nausea and vomiting are rare, but when they occur, they are serious. They are usually features of severe thyrotoxicosis but may also reflect hypercalcemia. In the presence of hypermetabolism, vomiting leads quickly to dehydration, ketosis, and perhaps avitaminosis.
The incidence of achlorhydria in exophthalmic goiter was found some years ago to be approximately 40% and slightly higher in myxedema [372]. The figures would be lower today. Berryhill and Williams [373]found that 73% showed a return of free hydrochloric acid after surgical thyroidectomy. Gastric enzyme production is decreased, and a mild chronic gastritis may be present [374]. Fasting serum gastrin levels, and responses to arginine, are increased [375].
Abdominal pain is an occasional symptom of thyrotoxicosis. Its nature and origin are obscure. Epigastric pain may suggest ulcer, gallbladder disease, or pancreatitis. Vomiting is sometimes associated with the pain.
The rate of absorption from the gastrointestinal tract is accelerated. The glucose tolerance curve may show an abnormally rapid rise and fall. Absorption of vitamin A is enhanced, and vitamin A formation from carotene is also increased.
Increased frequency of normal bowel movements is common, and occasionally diarrhea occurs. Transit time is decreased, and fat absorption may be impaired to the point of steatorrhea if fat intake is excessive [376].
The liver is frequently palpable in the absence of congestive heart failure, and is typically palpable with heart failure. Evidence of mild to severe liver disease may be found [377]. The plasma albumin level may be below 4 g/dl, and the globulin level above 3 g/dl. Galactose tolerance is impaired. There may be mild elevation of PT. The LFTs can give the impression of viral hepatitis. For example in one survey of 81 thyrotoxic patients, three-fourths had some LFT abnormality, including 31% with elevated bilirubin, 24% with elevated SGOT, 13% with elevated LDH, 26% with elevated SGPT, and 67% with elevated alkaline phosphatase (which of course may reflect bone metabolism). Cholesterol is often depressed. The abnormalities clear with treatment [378]. Bilirubin retention and jaundice are occasionally seen without evidence of congestive heart failure, but they are much more commonly found when this complication is also present. On hepatic biopsy the liver may be entirely normal histologically, even when there are abnormalities in chemical findings; alternatively, there may be evidence of focal collections of lymphocytes, decrease in glycogen, and occasionally death of cells. On electron microscopy, the mitochondria are increased in size and the smooth endoplasmic reticulum is hypertrophic. The glycogen level is decreased [379].The fine stellate scarrings seen in the livers of patients with severe thyrotoxicosis and reported in the earlier literature are rarely observed today. The cause of hepatic disease has been thought to be congestive heart failure, malnutrition, intercurrent infections, and a direct toxic effect of thyroid hormone. Malnutrition must play a role. Congestive heart failure by itself certainly can induce gross abnormalities in liver function, and presumably this insult is worsened by coincident thyrotoxicosis. The splanchnic blood flow is increased in thyrotoxicosis and the arteriovenous oxygen difference is greater than normal, but hepatic anoxia, at least in the portal areas, might occur even without circulatory failure if the metabolic demand for oxygen exceeds the supply.
Several patients who have been jaundiced without signs of congestive heart failure or other cause of hepatic dysfunction have been reported [380]. In two of these patients there was considerable elevation of the indirect-reacting bilirubin level. Studies in one patient showed that conjugation products of glucuronic acid were secreted into the urine in greatly increased quantities. This finding ruled out any absolute deficiency in the glucuronyl transferase enzyme in the liver. It was hypothesized that in certain thyrotoxic patients there is a great increase in metabolites that must be excreted via the glucuronyl transferase enzyme system. Since bilirubin competes relatively inefficiently in this enzyme system, it may be "crowded out" in the presence of an increased quantity of substrates. As a result, it may not be conjugated as rapidly as normally, and its concentration in the serum would therefore rise. All of these patients had residual abnormalities in bilirubin metabolism when euthyroid. This finding suggests that an underlying abnormality was present and was exacerbated by thyrotoxicosis. It is probable that the occasional thyrotoxic and jaundiced patient may actually suffer from an unrecognized separate abnormality, such as Gilbert's disease or posthepatitic liver dysfunction, brought to light by thyrotoxicosis.
URINARY TRACT
Polyuria and occasionally glycosuria are seen in uncomplicated thyrotoxicosis. Standard clinical renal function tests give normal results. Glycosuria may reflect accelerated absorption of sugar from the intestine and glucose intolerance.
In hyperthyroid animals and humans, the glomerular filtration rate and renal blood flow are on average increased, as are tubular transfer maxima for glucose and diodrast. The glomerular filtration rate and renal blood flow alterations probably are secondary to increased cardiac output, whereas the increased tubular activity may be a direct effect of thyroid hormone on renal function [381]. Polyuria does not indicate insensitivity to vasopressin, for the kidney responds normally to vasopressin with an increase in concentration of urine [382].
Hypercalcemia is a feature of severe thyrotoxicosis, but it rarely injures the kidneys. Occasionally hyposthenuria and uremia occur [307, 308], or more selective renal damage takes place. Huth et al [383] reported a patient with renal tubular acidosis coincident with hyperthyroidism. Circumstantial evidence suggested that hyperthyroidism led to hypercalcemia, which in turn had damaged the renal medulla and produced acidosis.
ENDOCRINE SYSTEM
( TABLE 10-7)
Table 7. Changes in Endocrine Function in Graves' Disease |
• FTI and T3 increased, TSH reduced • Prolactin normal • Growth hormone normal • Parathyroid hormone suppressed • Cortisol normal, urinary 17-OHCS increased, urinary free cortisol normal • Free testosterone reduced in males • Diabetic control worsened
|
FEMALE REPRODUCTIVE SYSTEM
Menstruation is characteristically decreased in volume. With severe thyrotoxicosis, the menstrual cycle may be either shortened or prolonged, and finally amenorrhea develops. The relative importance of a primary action of excess thyroid hormone on the ovary or uterus, and pituitary dysfunction, are unclear. In some cases, amenorrhea with a proliferative endometrium is found. This finding suggests failure of pituitary LH production and ovulation [384, 385].
Fertility is depressed, but pregnancy can develop. The incidence of miscarriage, premature delivery, pre-eclampsia and heart failure are increased by maternal hyperthyroidism [386, 387]. Evidence has been presented that high maternal thyroid hormone levels can lead to suppressed fetal TSH, lower fetal weight, and fetal death (388). Pregnancy, on the other hand, often ameliorates the symptoms of thyrotoxicosis due to Graves’ disease, but relapse is prone to occur in the 3-4 months following delivery (Figure 10-10). This topic is discussed in Chapter 14. Premature ovarian failure co-occurs with Graves' disease and thyroiditis in Multiple Endocrine Autoimmunity Type II [9]. Reduced fertility and increase miscarriage rates are associated with AITD and positive antibodies. Increased rates of thyroid dysfunction and positive antibody tests have been reported in infertile women (389). One study reports that treatment of euthyroid women with positive antibodies by administration of thyroxine reduced the incidence of miscarriage to the that found in anti body-negative women (390).
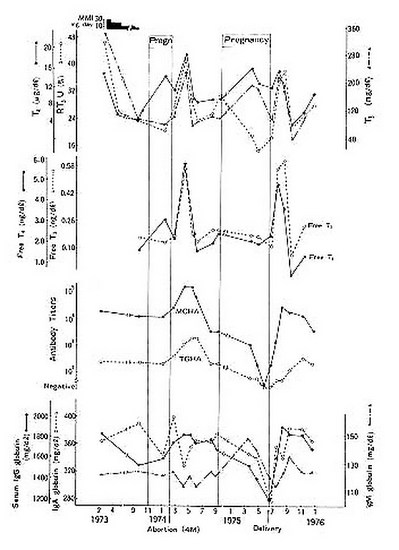
Figure 10. Clinical course of a patient who had transient exacerbation of Graves' disease on two occasions shortly following delivery.
Infants born to thyrotoxic mothers usually show no evidence of hyperthyroidism at birth. Fetal and neonatal thyrotoxicosis, fortunately infrequent events, are discussed in Chapter 14. Maternal thyrotoxicosis is associated with increased fetal loss (391), generally attributed to effects on the maternal system. However a recent study shows that elevated maternal thyroid hormone levels lead to elevated thyroid hormone levels in the fetus, and induce fetal loss. Surviving fetuses have lower birth weight (388).
MALE REPRODUCTIVE SYSTEM
Gynecomastia, with ductal elongation and epithelial hyperplasia, occurs occasionally. [392] The circulating level of free estradiol may be elevated in these men [393-395]. Peripheral conversion of testosterone and androstenedione to estrone and estradiol is increased in both sexes during hyperthyroidism [261]. This elevation probably accounts in part for the abnormality. In addition, the slightly elevated LH in men with gynecomastia suggests hypothalamic insensitivity to feedback control and some peripheral unresponsiveness to LH [393]. An imbalance between testosterone and estrogen may be related to gynecomastia.
Kidd et al [397] found impotence in half of a small group of thyrotoxic men and sperm counts below 40 million in four of five tested. In these studies, the total testosterone level was elevated, but because the testosterone-estrogen binding globulin level was also high, the free testosterone level was reduced and the response to hCG was blunted. In thyrotoxicosis, mean sperm density is lower, and fewer sperm have normal morphology. Motility is lower in thyrotoxic males. The abnormalities normalize when the patients become euthyroid (398). Thus, both Leydig cell and spermatogenic abnormalities may be present. Abalovich et al ( 399) reported similar findings, and in addition noted a high incidence of sperm abnormalities. All of the abnormalities returned to normal after therapy of the thyrotoxicosis. Radioiodine therapy can cause transient reductions in both sperm count and motility but do not seem to cause permanent effects with ordinary doses used in treatment under 14 mCi, equivalent to around 500 MBq (398).
PRL probably plays no role in these reproductive abnormalities, since in hyperthyroidism, its release tends to be inhibited both at the hypothalamic and pituitary level [400]. Surprisingly, galactorrhea, in women with normo-prolactinemia, is reported to occur in increased frequency [264].
ADRENAL CORTEX
There are no obvious signs or symptoms of altered adrenal cortical function in thyrotoxicosis, but distinct changes have been detected. In thyrotoxicosis the adrenal cortex is often hyperplastic. Administered adrenal steroids disappear from the plasma at an accelerated rate [265]. Their metabolism by reduction of the steroid nucleus is accelerated, and conjugation of the reduced steroids is proportionally increased. Since plasma corticoid levels are normal and their rate of metabolism is increased, total daily metabolism and excretion of 17-ketosteroids and 17 hydroxy-corticoids are usually increased [385,403].
Along with the accelerated plasma cortisol clearance of thyrotoxicosis, the pathways of metabolism are also altered. For example, thyrotoxicosis is associated with a relatively increased excretion of 11-oxycorticoid metabolites [267]. The 11-oxy compounds are biologically inactive. Because of the negative feedback control from the pituitary, this preferential channeling of steroids into the 11-oxy derivatives could be partly responsible for increased steroid production. There is increased production of steroids by the adrenal gland in order to maintain a normal concentration of active steroids in the peripheral blood and in the tissues [405]. Secretion of adrenocorticotropic hormone (ACTH) by the pituitary is reported to be increased [406]. There are increases in secretory episodes during the day, but the fall to zero secretion after midnight is retained.
A reduced response to exogenous ACTH [407] indicates that adrenal reserve is reduced. In fact, it has been hypothesized that in severe thyrotoxicosis and in thyroid storm there may be an element of adrenal insufficiency. This contention has not been proved. There is no reason to believe that T4 opposes the peripheral action of adrenal steroids.
An increase in the 5-alpha metabolite of testosterone (androsterone) and a relative decrease in the 5-beta metabolite (etiocholanolone) are seen in the urine of thyrotoxic patients [408], but no comparable change in adrenal corticoid metabolism has been observed. These interesting biochemical alterations could have physiologic significance, for the ketosteroid 5-alpha metabolites, such as androsterone, are biologically active. In hypothyroidism the reverse change occurs, that is, an increase in the biologically inactive 5-beta metabolites such as etiocholanolone. Because administration of large amounts of androsterone depresses the level of serum lipids, Hellman and co-workers [408] have hypothesized that this change in steroid metabolism may be a way in which T4 (or its lack) affects lipid metabolism and produces a depression or elevation in serum cholesterol concentration.
OTHER METABOLIC ASPECTS
The basal oxygen consumption in thyrotoxicosis, as measured by the BMR, is elevated. The increase is above the level of metabolism that the person would have if he or she were not thyrotoxic. In extreme thyrotoxicosis, the BMR may be double the standard, that is, according to the usual mode of expression, + 100. In moderately severe thyrotoxicosis it may be from +30 to +60, and in mild thyrotoxicosis from +10 to +30.
In addition to the BMR, one should consider the total metabolism, that is the basal plus the increments occasioned by work, food, or emotion. An increased cost of muscular work in thyrotoxicosis was reported many years ago by Plummer and Boothby [409] and Briard et al. [410] among others. These studies, whose results have been disputed, suggested that the thyrotoxic subject has less efficient coupling of oxidation and energy use than either the normal subject or, for example, the hypermetabolic patient with leukemia. Some recent studies indicate that the increase in energy expenditure caused by work is not altered by thyrotoxicosis [411], and other studies support the original observations.
CARBOHYDRATE METABOLISM
Absorption of carbohydrate from the intestine is accelerated, as is its removal from the plasma. After a standard oral glucose load is given, the thyrotoxic patient characteristically has an early and rapid rise in blood glucose concentration in 30 - 60 minutes (possibly to more than 200 mg/dl) and a rapid fall, so that by two hours the concentration is normal. The early peak may be associated with glycosuria. Intravenous administration does not usually elevate the blood glucose level beyond the rise found in normal subjects.
Thyrotoxicosis increases the demand for insulin, perhaps by accelerating its metabolism. In addition, resistance to the action of insulin is present, since in nondiabetic thyrotoxic patients normal fasting blood glucose levels are associated with double the normal insulin concentration [412], and resistance is found on incubation of adipocytes in vitro [413]. Diabetes may be activated or intensified, and is ameliorated or may disappear when the thyrotoxicosis is treated. Long ago experimental diabetes was shown to result from long-standing thyrotoxicosis in the presence of partial pancreatectomy [414]. The adverse effect of hyperthyroidism on glucose control in patients with non-insulin dependent diabetes is caused by increased basal hepatic glucose production and reduced ability of insulin and glucose to suppress hepatic glucose production [415, 416]. Although hyperthyroidism increases the requirement for insulin, the effectiveness of exogenous insulin on carbohydrate metabolism is actually enhanced. If glucose is administered intravenously and continuously to a thyrotoxic subject so that blood sugar is maintained at a high level, administered insulin has an effect on glucose removal from the blood [416] that is greater than that in the normal subject.
Type I diabetes mellitus co-occurs with increased frequency in autoimmune thyroid disease. Post-partum thyroid dysfunction is especially common in patients with diabetes [417]. In recent years many similarities in immunologic phenomena have been noted in the two diseases, including insulin receptor antibodies and islet cell antibodies.
LIPIDS
The serum cholesterol level is depressed in thyrotoxicosis. There is an increase both in synthesis and in degradation, but the balance results in a new lower steady-state concentration in the serum. The cholesterol pool in the body is altered by thyroid hormone in different directions, depending on the species involved, and does not necessarily parallel the serum cholesterol level. Hypocholesterolemia may be produced without a distinct decrease in total body or liver cholesterol [418-428]. Part of the cholesterol-lowering action of thyroid hormone may be due to the effects of malnutrition and weight loss, and part may be simply a manifestation of hypermetabolism, since agents that elevate metabolism, such as salicylates, also lower serum lipid levels.
Thyroid hormone directly enhances conversion of cholesterol to bile acids and their excretion in the bile. This metabolic route accounts for the disposal of 70-90% of the cholesterol formed in the body [421]. Thyroid hormone may also affect cholesterol metabolism by directly increasing the number of membrane surface low-density lipoprotein (LDL) receptors [422]. The increased cholesterol synthesis, and even more enhanced clearance rate in hyperthyroidism has been confirmed in a recent study. Hepatic lypogenesis is also strikingly increased, probably both by direct action of thyroid hormones and an increase in insulin. Triglyceride levels tend to be slightly elevated (423).
Levels of the other serum lipid components are lowered [424-429]. Plasma triglycerides are in the low normal range, and the clearance rate of infused triglycerides may be elevated. Postheparin lipolytic activity [425,426] may be low or normal. Hyperthyroidism causes lower levels of apo(a), HDL, and ratio of total/HDL cholesterol [420,426]. Plasma leptin levels are normal in hyperthyroid patients (427). The free tocopherol level of the plasma changes in parallel with the cholesterol alterations in thyrotoxicosis [429].
Rich et al. [430]found the level of nonesterified fatty acids elevated in thyrotoxic patients. This response can be seen within six hours after administration of L-T3 to normal subjects, and might be related to the observation that ketosis occurs more readily in thyrotoxic patients than in normal subjects.
PROTEIN METABOLISM
Protein formation and destruction are both accelerated in hyperthyroidism. Nitrogen excretion is increased, and nitrogen balance may be normal or negative, depending on whether intake meets the demands of increased catabolism. Testosterone is able to exert its anabolic effects in thyrotoxicosis.
Lewallen et al [431] found that administration of thyroid hormone increases albumin synthesis and degradation in normal subjects, increases the fractional rate of degradation, and reduces the quantity of exchangeable albumin.
Thyroid hormone in vivo [432] or in vitro [433], as reviewed in Chapter 2, exerts its basic action through stimulation of transcription and mRNA translation. Thyroid hormone stimulates incorporation of amino acids into protein by liver microsomes. This action is at least partially explained by increased production of mRNA and by an increased transfer of soluble tRNA- bound amino acids into microsomal protein [434]. Clinically, a great excess of thyroid hormone appears to have the opposite effect. Crispell et al. found that protein synthesis may be depressed by feeding thyroid hormone to normal humans [432]. Catabolism of collagen is increased, [435]and urinary hypodroxyproline excretion is characteristically increased. Gluconeogenesis from alanine is stimulated by thyroid hormone [436]. Probably the elevated somatomedin levels found in thyrotoxicosis [296] contribute to augmented protein synthesis.
L-carnitine feeding has been shown to reverse some of the metabolic abnormalities in Graves’ disease. In a randomized double-blind study, L-carnitine, in a dose of 2-4 grams/day also reversed or prevented some of the adverse effects of excess thyroid hormone on bone mineralization. Presumably this occurs because hyperthyroidism has depleted body stores of carnitine (437).
cAMP Metabolism
Basal cAMP levels are on average elevated in serum of thyrotoxic humans , and there is hyperresponsiveness to stimuli such as epinephrine and glucagon [438]. Urinary cAMP is likewise increased [439]. Treatment with propranolol (in some studies) lowers basal cAMP toward, but not to, normal [440]. Adrenergic receptors are increased in number, and responses are enhanced as compared to the normal state (see Chapter 2).
Vitamin Metabolism
The absorption of vitamin A is increased and conversion of carotene to vitamin A is accelerated in thyrotoxicosis. The requirements of the body are likewise increased, and low blood concentrations of vitamin A may be found.
Requirements for thiamine and vitamin B6 are increased [441]. Lack of the B vitamins has been implicated as a cause of liver damage in thyrotoxicosis. It has been demonstrated that vitamin B12 requirements are increased in experimental thyrotoxicosis.
Radiosensitivity
Radiosensitivity of animal and human tumors may be enhanced by administration of L-T3 [442].
DEATH IN THYROTOXICOSIS
Given current therapeutic resources, death from thyrotoxi cosis or from its treatment should be rare. Thyroid storm, when it occurs, can be a lethal event. In a study from Scottish hospitals, 20 of 33 hyperthyroid patients who died had congestive heart failure, 6 bronchial pneumonia, and 6 embolism in various sites. Nineteen had atrial fibrillation. Eight were considered to have thyroid storm [443]. One might suspect that several causes of death might be identified in any particular case, and that rarely if ever would pure thyrotoxicosis be the only assignable cause. In two recent deaths of which we are aware, in patients with fulminant thyrotoxicosis, the immediate cause was sudden cardiovascular collapse, with shock and arrhythmias including ventricular fibrillation, which was resistant to all usual resuscitative efforts.
DIFFERENCES BETWEEN THE SEXES
Over the years, we have become impressed that thyrotoxic men, considered collectively, present some striking differences from thyrotoxic women in their response to the disease. For a given rise in metabolism, the symptoms of women tend to be more conspicuous than do those of men. Of course, there are frequent exceptions. Men with moderately severe thyrotoxicosis often have few or minimal symptoms, whereas many women with much milder thyrotoxicosis often present an impressive array of symptoms.
A statistical study of symptoms and signs, chiefly circulatory, in a series of 184 thyrotoxic patients (52 men, 132 women), using 233 patients with nontoxic nodular goiter as controls [444], the male patients were somewhat older than the females, and there were more severe cases among men than among women. Cardiac symptoms were more common in women than in men, even though the men were older and more often had a severe form of the disease. Palpitations and dyspnea are both more common and more severe in the female than in the male group.
DIFFERENCES ATTRIBUTABLE TO AGE
Certain differences in symptoms are attributable to age. Classic exophthalmic goiter is relatively more frequent among younger patients, but severe infiltrative exophthalmos is virtually unknown before mid-adolescence. The character of the symptomatic response may be differentiated in another way [444]. In older patients circulatory symptoms are more in evidence. In older patients, emotional instability may be less evident, or depression may occur, and the symptoms and signs are manifestly circulatory. In many older patients with Graves' disease, the thyroid is not readily palpable. Anorexia in this group is fairly frequent, as is constipation. Devastating personality and emotional changes often appear in the child or adolescent with Graves' disease. Graves’ disease in very young children is associated problems in growth and development, including craniosynostosis. Children with thyrotoxicosis are tall for their age, and their bone ages are advanced at presentation. They tend to achieve final heights higher than their predicted target heights finally (445).
A study by Segni et al suggests that permanent brain damage can occur as a result of the illness (446).
REFERENCES
- Graves RJ: Clinical lectures. London Med Surg J (pt 2):516, 1835.
- DeGroot LJ, Quintans J. The causes of autoimmune thyroid disease. Endocrine Rev 10:537-562, 1989.
- Marino M, Chiovato L, Friedlander JA, Latrofa F, Pinchera A, McCluskey RT.1999Serum antibodies against megalin (GP330) in patients with autoimmune thyroiditis.J Clin Endocrinol Metab. 84:2468-2474.
- Morris JC, Bergert ER, Bryant WP: Binding of immunoglobulin G from patients with autoimmune thyroid disease to rat sodium-iodide symporter peptides: evidence for the iodide transporter as an autoantigen. Thyroid 7:527, 1997.
- Kubota S, Gunji K, Stolarski C, Kennerdell JS, Wall J: Reevaluation of the prevalences of serum autoantibodies reactive with "64-kd eye muscle proteins" in patients with thyroid-associated ophthalmopathy. Thyroid 8:175, 1998.
- Mariotti S, Kaplan EL, Medof ME, DeGroot LJ: Circulating thyroid antigen antibody immune complexes. Proceedings of the Eighth International Thyroid Congress, Sydney, Australia, February 3-8, 1980.
- Irvine WJ, Davies SH, Teitelbaum S et al: The clinical and pathological significance of gastric parietal cell antibody. Ann NY Acad Sci 124:657, 1965.
- Jenkins RC, Weetman AP. Disease associations with autoimmune thyroid disease.Thyroid. 2002 Nov;12(11):977-88
- Muir A, Schatz DA, MacLaren NK: Polyglandular failure syndromes. p. 3013. In DeGroot LJ (ed): Endocrinology, 3rd Ed. WB Saunders Co, Philadelphia, 1994.
- Katakura M, Yamada T, Aizawa T et al: Presence of antideoxyribonucleic acid antibody in patients with hyperthyroidism of Graves’ disease. J Clin Endocrinol Metab 64:405, 1987.
- Mackay IR, Taft LI, Cowling DC: Lupoid hepatitis. Lancet 2:1323, 1956.
- Siddiqi A, Monson JP, Wood DF, Besser GM, Burrin JM. Serum cytokines in thyrotoxicosis. J Clin Endocrinol Metab 84:435-439, 1999.
- Sonnet E, Massart C, Gibassier J, Allannic H, Maugendre D. 1999 Longitudinal study of soluble intercellular adhesion molecule-1 (ICAM-1) in sera of patients with Graves’ disease. J Endocrinol Invest. 22:430-435.
- Paggi A, Caccavo D, Ferri GM, Di Prima MA, Amoroso A, Vaccaro F, Bonomo L, Afeltra A. Anti-cardiolipin antibodies in autoimmune thyroid diseases. Clin Endocrinol 40:329-333, 1994.
- Ochi Y, DeGroot LJ: Vitiligo in Graves' disease. Ann Intern Med 71:935, 1969.
- Welti H: Skin manifestations associated with severe forms of Basedow’s disease. Bulletin der Schweirzerischen Akademie der Medizinischen Wissenschaften 23:476-482, 1968.
- Amoroso A, Garzia P, Pasquarelli C, Sportelli G, Afeltra A: Hashimoto’s thyroiditis associated with urticaria and angio-oedema: disappearance of cutaneous and mucosal manifestations after thyroidectomy. J Clin Pathol 50:254-256, 1997.
- Marino M, Ricciardi R, Pinchera A, Barbesino G, Manetti L, Chiovato L, Braverman LE, Rossi B, Muratorio A, Mariotti S: Mild clinical expression of myasthenia gravis associated with autoimmune thyroid diseases. J Clin Endocrinol Metab 82:438-443, 1997.
- Marshall J: ITP and Graves’ disease. Ann Intern Med 67:411, 1967.
- White RG, Bass BH, Williams E: Lymphadenoid goiter and the syndrome of systemic lupus erythematosus. Lancet 1:368, 1961.
- Mori T, Kriss JP: Measurements by competitive binding radioassay of serum antimicrosomal and antithyroglobulin antibodies in Graves’ disease and other thyroid disorders. J Clin Endocrinol Metab 33:688, 1971.
- Wang P-W, Huang M-J, Liu R-T, Chen CD: Triiodothyronine autoantibodies in Graves' disease: Their changes after antithyroid therapy and relationship with the thyroglobulin antibodies. Acta Endocrinol (Copenh) 122:22-28, 1990.
- Nakamura S, Sakata S, Shima H, Komaki T, Kojima N, Kamikubo K, Yasuda K, Miura K: Thyroid hormone autoantibodies (THAA) in two cases of Graves' disease: Effects of antithyroid drugs, prednisolone, and subtotal thyroidectomy. Endocrinol Japon 33:751-759, 1986.
- Kasagi K, Kousaka T, Higuchi K, Iida Y, Misaki T, Alam MS, Miyamoto S, Yamabe H, Konishi J: Clinical significance of measurements of antithyroid antibodies in the diagnosis of Hashimoto’s thyroiditis: Comparison with histological findings. Thyroid 6:445, 1996.
- Amino N, Hagen SR, Yamada N, Refetoff S: Measurement of circulating thyroid microsomal antibodies by the tanned red cell-hemagglutination technique. Its usefulness in the diagnosis of autoimmune thyroid disease. Clin Endocrinol 5:115, 1976.
- Mullins RJ, Cohen SBA, Webb LMC, Chernajovsky Y, Dayan CM, Londei M, Feldmann M: Identification of thyroid stimulating hormone receptor-specific T cells in Graves’ disease thyroid using autoantigen-transfected Epstein-Barr Virus-transformed B cell lines. J Clin Invest 96:30-37, 1995.
- Weetman AP, Gunn C, Hall R, McGregor AM: Thyroid autoantigen-induced lymphocyte proliferation in Graves’ disease and Hashimoto’s thyroiditis. J Clin Lab Immunol 17:1, 1985.
- Fisfalen M-E, DeGroot LJ, Quintans J, Franklin WA, Soltani K: Microsomal antigen reactive lymphocyte lines and clones derived from thyroid tissue of patients with Graves’ disease. J Clin Endocrinol Metab 66:776-784, 1988.
- Soliman M, Kaplan E, Fisfalen M-E, Okamoto Y, DeGroot LJ: T cell reactivity to recombinant human thyrotropin receptor extracellular domain and thyroglobulin in patients with autoimmune and non-autoimmune thyroid diseases. J Clin Endocrinol Metab 80:206-213, 1995.
- Aoki N, DeGroot LJ: Lymphocyte blastogenic response to human thyroglobulin in Graves' disease, Hashimoto's thyroiditis, and metastatic cancer. Clin Exp Immunol 38:523, 1979.
- DeGroot LJ, Kawakami Y, Fisfalen M-E, Okamoto Y, Yanagawa T. T cell epitopes in TPO and TSH receptor. Proceedings of the International Hashimoto Symposium--80th Anniversary of Hashimoto's Disease, Fukuoka, Japan, December 2-5, 1992.
- Soliman M, Kaplan EL, Yanagawa T, Hidaka Y, Fisfalen M-E, DeGroot LJ: T-cells recognize multiple epitopes in the human thyrotropin receptor extracellular domain. J Clin Endocrinol Metab 80:905-914, 1995.
- Kula D, Bednarczuk T, Jurecka-Lubieniecka B, Polanska J, Hasse-Lazar K, Jarzab M, Steinhof-Radwanska K, Hejduk B, Zebracka J, Kurylowicz A, Bar-Andziak E, Stechly T, Pawlaczek A, Gubala E, Krawczyk A, Szpak-Ulczok S, Nauman J, Jarzab B Interaction of HLA-DRB1 alleles with CTLA-4 in the predisposition to Graves' disease: the impact of DRB1*07. Thyroid.2006 May;16(5):447-53.
- Tandon N, Freeman M, Weetman AP: T cell responses to synthetic thyroid peroxidase peptides in autoimmune thyroid disease. Clin Exp Immunol 86:56-60, 1991.
- Fisfalen M-E, Soliman M, Okamoto Y, Soltani K, DeGroot LJ: Proliferative responses of T-cells to thyroid antigens and synthetic thyroid peroxidase peptides in autoimmune thyroid disease. J Clin Endocrinol Metab 80:1597-1604, 1995.
- Ploth DW, Fitz A, Schnetzker D et al: Thyroglobulin-anti-thyroglobulin immune complex glomerulone-phritis complicating radioiodine therapy, Clin Immunol Immunopathol 9:327, 1978.
- Matsuura M, Kikkawa Y, Akashi K et al: Thyroid antigen-antibody nephritis: Possible involvement of fucosyl-GMI as the antigen. Endocrinol Japon 34:587, 1987.
- Chiovato L, Bassi P, Santini F et al: Antibodies producing complement-mediated thyroid cytotoxicity in patients with atrophic or goitrous autoimmune thyroiditis. J Clin Endocrinol Metab 77:1700, 1993.
- Tektonidou MG, Anapliotou M, Vlachoyiannopoulos P, Moutsopoulos HM. Presence of systemic autoimmune disorders in patients with autoimmune thyroid diseases.Ann Rheum Dis. 2004 Sep;63(9):1159-61.
- der Kinderen PJ, Houstra-Lanz M, Schwarz F: Exophthalmos- producing substance in human serum. J Clin Endocrinol Metab 20:712, 1960.
- Dobyns BM, Steelman SL: The thyroid-stimulating hormone of the anterior pituitary as distinct from exophthalmos producing substance. Endocrinology 52:705, 1953.
- Adams DD: The presence of an abnormal thyroid-stimulating hormone in the serum of some thyrotoxic patients. J Clin Endocrinol Metab 18:699, 1958.
- Adams DD, Fastier FN, Howie JB, Kennedy TH, Kilpatrick JA, Stewart RDH: Stimulation of the human thyroid by infusions of plasma containing LATS protector. J Clin Endocrinol Metab 39:826, 1974.
- Smith BR, Hall R: Binding of thyroid stimulators to thyroid membranes. FEBS Lett 42:301, 1974.
- Huber GK, Safirstein R, Neufeld D, Davies TF: Thyrotropin receptor autoantibodies induce human thyroid cell growth and c-fos activation. J Clin Endocrinol Metab 72:1142-1147, 1991.
- Cornelis S, Uttenweiler-Joseph S, Panneels V, Vassart G, Costagliola S. Purification and characterization of a soluble bioactive amino-terminal extracellular domain of the human thyrotropin receptor. Biochemistry 40:9860-9869, 2001.
- Rees Smith B, McLachlan SM, Furmaniak J: Autoantibodies to the thyrotropin receptor. Endocr Rev 9:106-121, 1988.
- Ludgate ME, Vassart G: The thyrotropin receptor as a model to illustrate receptor and receptor antibody diseases. Baillieres Clin Endocrinol Metab 9:95-113, 1995.
- Orgiazzi J, Williams DE, Chopra IJ, Solomon DH: Human thyroid adenyl cyclase-stimulating activity in immunoglobulin G of patients with Graves' disease. J Clin Endocrinol Metab 42:341, 1976.
- Evans C, Morgenthaler NG, Lee S, Llewellyn DH, Clifton-Bligh R, John R, Lazarus JH, Chatterjee VKK, Ludgate M. Development of a luminescent bioassay for thyroid stimulating antibodies. J Clin Endocrinol Metab 84:374, 1999.
- Di Cerbo A, Di Paola R, Menzaghi C, Filippis VD, Tahara K, Corda D, et al. 1999 Graves' immunoglobulins activate phospholipase A2 by recognizing specific epitopes on thyrotropin receptor. J Clin Endocrinol Metab 84:3283-3292.
- Drexhage HA, Bottazzo GF, Doniach D, Bitensky L, Chayen J: Evidence for thyroid growth-stimulating immunoglobulins in some goitrous thyroid diseases. Lancet 2:287, 1980
- Endo K, Kasagi K, Konishi J, Ikekubo K, Okuno T, Takeda Y, Mori T, Torizuka K: Detection and properties of TSH-binding inhibitor immunoglobulins in patients with Graves' disease and Hashimoto's thyroiditis. J Clin Endocrinol Metab 46:734, 1978.
- Irvine WJ, Lamberg B-A, Cullen DR, Gordin R: Primary hypothyroidism preceding thyrotoxicosis: A report of 2 cases and a review of the literature. J Clin Lab Immunol 2:349, 1979.
- Eckstein AK, Plicht M, Lax H, Neuhauser M, Mann K, Lederbogen S, Heckmann C, Esser J, Morgenthaler NG.Thyrotropin receptor autoantibodies are independent risk factors for Graves' ophthalmopathy and help to predict severity and outcome of the disease. J Clin Endocrinol Metab. 2006 Sep;91(9):3464-70.
- Zakarija M, McKenzie JM: Isoelectric focusing of thyroid-stimulating antibody of Graves' disease. Endocrinology 103:1469, 1978.
- Endo K, Amir SM, Ingbar SH: Development and evaluation of a method for the partial purification of immunoglobulins specific for Graves' disease. J Clin Endocrinol Metab 52:113, 1981.
- Misrahi, M; Milgrom, E. Cleavage and shedding of the TSH receptor. Europ J Endocrinol 137 599-602 1997.
- McKenzie JM: Further evidence for a thyroid activator in hyperthyroidism. J Clin Endocrinol Metab 20:380, 1960. .
- Takasu N, Oshiro C, Akamine H, Komiya I, Nagata A, Sato Y, Yoshimura H, Ito K: Thyroid-stimulating antibody and TSH-binding inhibitor immunoglobulin in 277 Graves’ patients and in 686 normal subjects. J Endocrinol Invest 20:452-461, 1997.
- Bolk JH, Elte JWF, Bussemaker JK, Haak A, Van Der Heide D: Thyroid-stimulating immunoglobulins do not cause non- autonomous, autonomous, or toxic multinodular goiters. Lancet 1:61, 1979.
- Kuzuya N, Chiu SC, Ikeda H, Uchimura H, Ito K, Nagataki S: Correlation between thyroid stimulators and 3,5,3'-triiodo- thyronine suppressibility in patients during treatment for hyperthyroidism with thionamide drugs: Comparison of assays by thyroid-stimulating and thyrotropin-displacing activities. J Clin Endocrinol Metab 48:706, 1979.
- Soliman M, Kaplan EL, Abdel-Latif A, Scherberg N, DeGroot LJ: Does thyroidectomy, RAI therapy, or antithyroid drug treatment alter reactivity of patients’ T cells to epitopes of thyrotropin receptor in autoimmune thyroid diseases? J Clin Endocrinol Metab 80:2312-2321, 1995.
- Fenzi G, Hashizume K, Roudebush CP, DeGroot LJ: Changes in thyroid-stimulating immunoglobulins during antithyroid therapy. J Clin Endocrinol Metab 48:572, 1979.
- Mukhtar ED, Smith BR, Pyle GA et al: Relation of thyroid-stimulating immunoglobulins to thyroid function and effect of surgery, radioiodine, and antithyroid drugs. Lancet 1:713, 1975.
- Tahara K, Ishikawa N, Yamamoto K, Hirai A, Ito K, Tamura Y, Yoshida S, Saito Y, Kohn LD: Epitopes for thyroid stimulating and blocking autoantibodies on the extracellular domain of the human thyrotropin receptor. Thyroid 7:867, 1997.
- Mullins RJ, Cohen SBA, Webb LMC, Chernajovsky Y, Dayan CM, Londei M, Feldmann M: Identification of thyroid stimulating hormone receptor-specific T cells in Graves’ disease thyroid using autoantigen-transfected Epstein-Barr virus-transformed B cell lines. Clin Invest 96:30-37, 1995.
- Fisfalen M-E, Soliman M, Okamoto Y, Soltani K, DeGroot LJ. Proliferative responses of T cells to thyroid antigens and synthetic TPO peptides in autoimmune thyroid disease. J Clin Endocrinol Metab 80:1597-1604, 1995.
- Fisfalen M-E, Palmer EM, van Seventer GA, Soltani K, Sawai Y, Kaplan E, Hidaka Y, Ober C, DeGroot LJ: TSH-R and TPO specific T cell clones and their cytokine profile in autoimmune thyroid disease. J Clin Endocrinol Metab 82:3655-3663, 1997.
- Fisfalen M-E, Palmer EM, Van Seventer GA, Soltani K, Sawai Y, Kaplan E, Hidaka Y, Ober C, DeGroot, LJ. Thyrotropin-receptor and thyroid peroxidase-specific T cell clones and their cytokind profile in autoimmune thyroid disease. J Clin Endocrinol Metab 82:3655-3663, 1997.
- Mullins RJ, Cohen SBA, Webb LMC, Chernajovsky Y, Dayan CM, Londei M, Feldmann M. Identification of thyroid stimulating hormone receptor-specific T cells in Graves’ disease thyroid using autoantigen-transfected Epstein-Barr virus-transformed B cell lines. J Clin Invest 96:30-37, 1995.
- Inaba H, Martin W, Ardito M, De Groot AS, De Groot LJ.The role of glutamic or aspartic acid in position four of the epitope binding motif and thyrotropin receptor-extracellular domain epitope selection in Graves' disease.J Clin Endocrinol Metab. 2010 Jun;95(6):2909-16
- Crisp M, Starkey KJ, Lane C, Ham J, Ludgate M. Adipogenesis in thyroid eye disease. Investigative Ophthalmol Visual Sci 41:3249-3255, 2000.
- Bell A, Gagnon A, Grunder L, Parikh SJ, Smith TJ, Sorisky A. Functional TSH receptor in human abdominal preadipocytes and orbital fibroblasts. Amer J Physiol - Cell Physiol. 279:C335-C340, 2000.
- Haraguchi K, Shimura H, Kawaguchi A, Ikeda M, Endo T, Onaya T. Effects of thyrotropin on the proliferation and differentiation of cultured rat preadipocytes. Thyroid 9:613, 1999.
- Hiromatsu Y, Yang D, Bednarczuk T, Miyake I, Nonaka K, Inoue Y. Cytokine profiles in eye muscle tissue and orbital fat tissue from patients with thyroid-associated ophthalmopathy. J Clin Endocrinol Metab 85:1194-1199, 2000.
- Wakelkamp IM, Gerding MN, Van Der Meer JW, Prummel MF, Wiersinga WM. Both Th1- and Th2-derived cytokines in serum are elevated in Graves' ophthalmopathy. Clin Exper Immunol 121:453-457, 2000.
- Nygaard B, Metcalfe RA, Phipps J, Weetman AP, Hegedus L. Graves' disease and thyroid associated ophthalmopathy triggered by 131-I treatment of non-toxic goiter. J Endocrinol Invest 22:481-485, 1999.
- Ludgate M. Animal models of Graves' disease. Europ J Endocrinol 142:1-8, 2000.
- Flynn JC, Rao PV, Gora M, Alsharabi G, Wei W, Giraldo AA, David CS, BangaJP, Kong YM. Graves' hyperthyroidism and thyroiditis in HLA-DRB1*0301 (DR3) transgenic mice after immunization with thyrotropin receptor DNA.Clin Exp Immunol. 2004 Jan;135(1):35-40.
- Graves' animal models of Graves' hyperthyroidism. Thyroid. 2007 Oct;17(10):981-8.
- Horie I, Abiru N, Saitoh O, Ichikawa T, Iwakura Y, Eguchi K, Nagayama Y. Distinct role of T helper Type 17 immune response for Graves' hyperthyroidism in mice with different genetic backgrounds. Autoimmunity. 2011 Mar;44(2):159-65
- Smith TJ, Hoa N. Immunoglobulins from patients with Graves' disease induce hyaluronan synthesis in their orbital fibroblasts through the self-antigen, insulin-like growth factor-I receptor. J Clin Endocrinol Metab. 2004 Oct;89(10):5076-80
- Douglas RS, Naik V, Hwang CJ, Afifiyan NF, Gianoukakis AG, Sand D, Kamat S, Smith TJ. J Immunol. 2008 Oct 15;181(8):5768-74. B cells from patients with Graves' disease aberrantly express the IGF-1 receptor: implications for disease pathogenesis.
- Schwiebert C, Morgenthaler NG, Köhrle J, Eckstein A, Schomburg L. Autoantibodies to the IGF1 receptor in Graves' orbitopathy. J Clin Endocrinol Metab. 2013 Feb;98(2):752-60
- Krieger CC, Neumann S, Place RF, Marcus-Samuels B, Gershengorn MC. Bidirectional TSH and IGF-1 Receptor Cross Talk Mediates Stimulation of Hyaluronan Secretion by Graves' Disease Immunoglobins. J Clin Endocrinol Metab. 2015 Mar;100(3):1071-7.
- van Steensel L, Hooijkaas H, Paridaens D, van den Bosch WA, Kuijpers RW, Drexhage HA, van Hagen PM, Dik WA.PDGF enhances orbital fibroblast responses to TSHR stimulating autoantibodies in Graves' ophthalmopathy patients.J Clin Endocrinol Metab. 2012 Jun;97(6):
- Hamada N, DeGroot LJ, Portmann L, Yamakawa J, Noh J, Okamoto Y, Ohno M, Ito K, Morii H. Thyroid microsomal antigen in Graves’ thyroid is not different from that in normal thyroid. Endocrinol Japon 38:471-478, 1991.
- Holm IA, Manson JE, Michels KB, Alexander EK, Willett WC, Utiger RD.Smoking and other lifestyle factors and the risk of Graves' hyperthyroidism.Arch Intern Med. 2005 Jul 25;165(14):1606-11
- Abbas AK, Lichtman AH, Pober JS: Cellular and molecular immunology. Philadelphia: WB Saunders, 1991.
- Strominger JL: Developmental biology of T cell receptors. Science 244:943-950, 1989.
- Spitzweg C, Joba W, Heufelder AE. Expression of thyroid-related genes in human thymus. Thyroid 9:133, 1999.52.11. .
- Sospedra M, Ferrer-Francesh X, Dominguez O, Juan M, Foz-Sala M, Pujol-Borrell R. Transcription of a broad range of self antigens in human thymus suggests a role for central mechanisms in tolerance toward peripheral antigens. J Immunol 161:5918-5929, 1998.
- Giménez-Barcons M, Casteràs A, Armengol Mdel P, Porta E, Correa PA, Marín A, Pujol-Borrell R, Colobran R. Autoimmune predisposition in Down syndrome may result from a partial central tolerance failure due to insufficient intrathymic expression of AIRE and peripheral antigens. J Immunol. 2014 Oct 15;193(8):3872-9
- Wenzel BE, Franke TF, Heufelder AE: Autoimmune thyroid diseases and enteropathogenic Yersinia enterocoliitica. Autoimmunity 7:295-303, 1990.
- Wolf MW, Misaki T, Bech K, Tvede M, Silva JE, Ingbar SH: Immunoglobulins of patients recovering from Yersinia enterocolitica infections exhibit Graves’ disease-like activity in human thyroid membranes. Thyroid 1:315-320, 1991.
- Wenzel BE, Heesemann J, Wenzel KW, Scriba PC: Patients with autoimmune thyroid diseases have antibodies to plasmid encoded proteins of enteropathogenic Yersinia. J Endocrinol Invest 11:139-140, 1988.
- Weiss M, Ingbar SH, Winblad S, Kasper DL: Demonstration of a saturable binding site for thyrotropin in Yersinia enterocolitica. Science 219:1331-1333, 1983.
- Werner J, Gelderblom H: Isolation of foamy virus from patients with de Quervain thyroiditis. Lancet 2:258-259, 1979.
- Nakachi K, Takasu N, Akamine H, Komiya I, Ishikawa K, Shinjyo T, Masuda M: Association of HLTV-1 with autoimmune thyroiditis in patients with adult T-cell leukemia (ATL) and in HTLV-1 carriers and a patient of ATL with autoimmune thyroiditis and uveites. Abstract No. PO27, presented at the 6th Asia and Oceania Thyroid Association Congress, November 9-12, 1997, Osaka, Japan.
- de Luis DA, Varela C, de la Calle H, Canton R, de Argila CM, San Roman AL, Boixeda D. Helicobacter pylori infection is markedly increased in patients with autoimmune atrophic thyroiditis. J Clin Gastroenterol 26:259-263, 1998.
- Szlachcic A, Sliwowski Z, Karczewska E, Bielanski W, Pytko-Polonczyk J, Konturek SJ. Helicobacter pylori and its eradication in rosacea. J Physiol Pharmacol 50:777-786. 1999.
- Rochman H, DeGroot LJ, Rieger CHL, Varnavides LA, Refetoff S, Joung JI, Hoye K: Carcinoembryonic antigen and humoral antibody response in patients with thyroid carcinoma. Cancer Research 35:2689-2692, 1975.
- Hancock SL, Cox RS, McDougall IR: Thyroid diseases after treatment of Hodgkin's disease. N Engl J Med 325:599, 1991.
- Vermiglio F, Castagna MG, Volnova E, Lo Presti VP, Moleti M, Violi MA, et al. 1999 Post-Chernobyl increased prevalence of humoral thyroid autoimmunity in children and adolescents from a moderately iodine-deficient area in Russia. Thyroid 9:781.
- Monzani F, Del Guerra P, Caraccio N, Casolaro A, Lippolis PV, Goletti O: Appearance of Graves’ disease after percutaneous ethanol injection for the treatment of hyperfunctioning thyroid adenoma. J Endocrinol Invest 20:294-298, 1997.
- Nygaard B, Helmer Knudsen J, Hegedus L, Vege Cand Scient A, Erik Molholm Hansen J: Thyrotropin receptor antibodies and Graves’ disease, a side effect of 131-I treatment in patients with nontoxic goiter. J Clin Endocrinol Metab 82:2926-2930, 1997.
- Schmidt M, Gorbauch E, Dietlein M, Faust M, Stutzer H, Eschner W, Theissen P, Schicha HIncidence of postradioiodine immunogenic hyperthyroidism/Graves' disease in relation to a temporary increase in thyrotropin receptor antibodies after radioiodine therapy for autonomous thyroid disease. Thyroid.2006 Mar;16(3):281-8.
- Klavinskis LS, Notkins AL, Oldstone MBA: Persistent viral infection of the thyroid gland: alteration of thyroid function in the absence of tissue injury. Endocrinology 122:567, 1988.
- Ronnblom LE, Alm GV, Oberg KE: Autoimmunity after alpha-interferon therapy for malignant carcinoid tumors. Ann Intern Med 115:178-183, 1991.
- Van Liessum PA, De Mulder PHM, Mattijssen EJM, Corstens FHM, Wagener DJT: Hypothyroidism and goiter during interleukin-2 therapy without LAK cells. Lancet 1:224, 1989.
- Hoekman K, von Blomberg-Van Der Flier BME, Wagstaff J, Drexhage HA, Pinedo HM: Reversible thyroid dysfunction during treatment with GM-CSF. Lancet 338:541-542, 1991.
- Brix TH, Christensen K, Holm NV, Harvald B, Hegedus L. A population-based study of Graves’ disease in Danish twins. Clinical Endocrinol 48:397-400, 1998
- Coles AJ, Wing MG, Hale G, Waldmann H, Weetman AP, Compston DAS, Chatterjee VKK. Anti-lymphocyte monoclonal antibody treatment of multiple sclerosis suppresses disease activity but induces Graves’ disease. J Endocrinol Invest 21:3, 1998
- Figueroa-Vega N, Alfonso-Pérez M, Benedicto I, Sánchez-Madrid F, González-Amaro R, Marazuela M. Increased circulating pro-inflammatory cytokines and Th17 lymphocytes in Hashimoto's thyroiditis. J Clin Endocrinol Metab. 2010 Feb;95(2):953-62
- Prummel, MF; Laurberg, P. Interferon- and autoimmune thyroid disease.Thyroid 13 547 2003
- Durelli L, Ferrero B, Oggero A, Verdun E, Ghezzi A, Montanari E, Zaffaroni M, the Betaferon Safety Trial Study Group. Thyroid function and autoimmuinity during Interferon alpha-1b treatment: A multicenter prospective study. J Clin Endocrinol Metab 86:3525-3532, 2001
- Bartels ED: Heredity in Graves' Disease. Copenhagen, Enjnar Munksgaards Forlag, 1941.
- Martin L: The hereditary and familial aspect of exophthalmic goiter and nodular goiter. Q J Med 14:207, 1945.
- Harvald B, Hauge M: A catamnestic investigation of Danish twins. Dan Med Bull 3:150, 1956.
- Brix TH, Kyvik KO, Christensen K, Hegedus L. Evidence for a major role of heredity in Graves’ disease: A population-based study of two Danish twin cohorts. J Clin Endocrinol Metab 86:930-934, 2001.
- Hansen PS, Brix TH, Iachine I, Kyvik KO, Hegedus L. The relative importance of genetic and environmental effects for the early stages of thyroid autoimmunity: a study of healthy Danish twins. Eur J Endocrinol.2006 Jan;154(1):29-38.
- Grumet FC, Payne RO, Konishi J, Kriss JP: HL-A antigens as markers for disease susceptibility and autoimmunity in Graves' disease. J Clin Endocrinol Metab 39:1115, 1974.
- Farid NR, Stone E, Johnson G: Graves' disease and HLA: Clinical and epidemiologic associations. Clin Endocrinol 13:535, 1980.
- Mangklabruks A, Cox N, DeGroot LJ: Genetic factors in autoimmune thyroid disease analyzed by restriction fragment length polymorphisms of candidate genes. J Clin Endocrinol Metab 73:236-244, 1991.
- Yanagawa T, Mangklabruks A, Chang Y-B, Okamoto Y, Fisfalen M-E, Curran PG, DeGroot LJ. Human histocompatibility leukocyte antigen-DQA1*0501 allele associated with genetic susceptibility to Graves' disease in a Caucasian population. J Clin Endocrinol Metab 76:1569-1574, 1993.
- Yanagawa T, Mangklabruks A, DeGroot LJ. Strong association between HLA-DQA1*0501 and Graves’ disease in a male Caucasian population. J Clin Endocrinol Metab 79:227-229, 1994.
- Chen Q-Y, Huang W, She J-X, Baxter F, Volpe R, MacLaren NK. 1999 HLA-DRB1*08, DRB1*03/DRB3*0101, and DRB3*0202 are susceptibility genes for Graves' disease in North American Caucasians, whereas DRB1*07 is protective. J Clin Endocrinol Metab 84:3182-3186.
- Trowsdale J, Ragoussis J, Campbell RD: Caption for Figure 2: The map of the human MHC is a compilation of physical mapping and cloning data from a number of laboratories. Immunol Today 14:349-352, 1993.
- Geluk A, Van Meijgaarden KE, Janson AAM, Wouter Drijfhout J, Meloen RH, DeVries RRP, Ottenhoff THM: Functional analysis of DR17(DR3)-restricted mycobacterial T cell epitopes reveals DR17-binding motif and enables the design of allele-specific competitor peptides. J Immunol 149:2864-2871, 1992.
- Sawai Y, DeGroot LJ. Binding of human thyrotropin receptor peptides to a Graves' disease predisposing human leukocyte antigen Class II molecule. J Clin Endocrinol Metab 85:1176-1179, 2000.
- Farid NR, Sampson L, Moens H, Barnard JM: The association of goitrous autoimmune thyroiditis with HLA-DR5. Tissue Antigens 21:173-175, 1981.
- Kong YC, Lomo LC, Motte RW, Giraldo AA, Baisch J, Strauss G, Hammerling GJ, David CS. HLA-DRB1 polymorphism determines susceptibility to autoimmune thyroiditis in transgenic mice: definitive association with HLA-DRB1*0301 (DR3) gene. J Exp Med 184:1167-1172, 1996.
- Pichurin, P; Chen, C-R; Pichurina, O; David, C; Rapoport, B; McLachlan, SM. Thyrotropin receptor-DNA vaccination of transgenic mice expressing HLA-DR3 or HLA-DQ6b. Thyroid 13 911-917 2003.
- Yanagawa T, Hidaka Y, Guimaraes V, Soliman M, DeGroot LJ: CTLA-4 gene polymorphism associated with Graves’ disease in a Caucasian population. J Clin Endocrinol Metab 80:41-45, 1995.
- Yanagawa T, Taniyama M, Enomoto S, Gomi K, Maruyama H, Ban Y, Saruta T: CTLA4 gene polymorphism confers susceptibility to Graves’ disease in Japanese. Thyroid 7:843, 1997.
- Thompson CB: Distinct roles for the costimulatory ligands B7-1 and B7-2 in T helper cell differentiation? Cell 81:979-982, 1995.
- Karandikar NJ, Vanderlugt CL, Walunas TL, Miller SD, Bluestone JA: CTLA4: A negative regulator of autoimmune disease. J Exp Med 184:783-788, 1996.
- Donner H, Rau H, Walfish PG, et al: CTLA4 alanine-17 confers genetic susceptibility to Graves’ disease and to Type I Diabetes Mellitus. J Clin Endocrinol Metab 82:143-146, 1997.
- Heward JM, Allahabadia A, Armitage M, Hattersley A, Dodson PM, MacLeod K, et al. 1999 The development of Graves' disease and the CTLA-4 gene on chromosome 2q33. J Clin Endocrinol Metab 84:2398-2401.
- Vaidya B, Imrie H, Perros P, Young ET, Kelly WF, Carr D, et al. 1999 The cytotoxic T lymphocyte antigen-4 is a major Graves' disease locus. Human Molecul Genet 8:1195-1199.
- Zaletel, K; Krhin, B; Gaberscek, S; Pirnat, E; Hojker, S. The influence of the exon 1 polymorphism of the cytotoxic T lymphocyte antigen 4 gene on thyroid antibody production in patients with newly diagnosed Graves’ disease. Thyroid 12 373 2002
- Kouki T, Sawai Y, Gardine C, Fisfalen M-E, Alegre M-L, DeGroot LJ. CTLA-4 gene polymorphism at position 49 in exon 1 reduces the inhibitory function of CTLA-4 and contributes to the pathogenesis of Graves' disease. J Immunol 165:6606-6611, 2000.
- Cuddihy RM, Dutton CM, Bahn RS: A polymorphism in the extracellular domain of the thyrotropin receptor is highly associated with autoimmune thyroid disease in females. Thyroid 5:89-95, 1995.
- Kotsa KD, Watson PF, Weetman AP: No association between a thyrotropin receptor gene polymorphism and Graves’ disease in the female population. Thyroid 7:31, 1997.
- Hiratani H, Bowden DW, Ikegami S, Shirasawa S, Shimizu A, Iwatani Y, Akamizu T Multiple SNPs in intron 7 of thyrotropin receptor are associated with Graves' disease. J Clin Endocrinol Metab. 2005 May;90(5):2898-903. Epub 2005 Mar 1.
- Dechairo BM, Zabaneh D, Collins J, Brand O, Dawson GJ, Green AP, Mackay I,Franklyn JA, Connell JM, Wass JA, Wiersinga WM, Hegedus L, Brix T, Robinson BG,Hunt PJ, Weetman AP, Carey AH, Gough SC.Association of the TSHR gene with Graves' disease: the first disease specific locus. Eur J Hum Genet. 2005 Aug 17;
- Vaidya B, Imrie H, Perros P, Young ET, Kelly WF, Carr D, Large DM, Toft AD, Kendall-Taylor P, Pearce SH. Evidence for a new Graves’ disease susceptibility locus at chromosome 18q21. Amer J Human Genetics 66:1710-1714, 2000.
- Ban Y, Taniyama M, Ban Y. Vitamin D receptor gene polymorphism is associated with Graves' disease in the Japanese population. J Clin Endocrinol Metab 85:4639-4643, 2000.
- Tomer Y, Concepcion E, Greenberg DA. A C/T single-nucleotide polymorphism in the region of the CD40 gene is associated with Graves’ disease. Thyroid 12:1129, 2002.
- Kurylowicz A, Kula D, Ploski R, Skorka A, Jurecka-Lubieniecka B, Zebracka J, Steinhof-Radwanska K, Hasse-Lazar K, Hiromatsu Y, Jarzab B, Bednarczuk T. Association of CD40 Gene Polymorphism (C-1T) with Susceptibility and Phenotype of Graves' Disease. Thyroid.2005 Oct;15(10):1119-24.
- Lopez ER, Zwermann O, Segni M, Meyer G, Reincke M, Seissler J, Herwig J, Usadel KH, Badenhoop K. A promoter polymorphism of the CYP27B1 gene is associated with Addison's disease, Hashimoto's thyroiditis, Graves' disease and type 1 diabetes mellitus in Germans. Eur J Endocrinol. 2004 Aug;151(2):193-7
- Shirasawa S, Harada H, Furugaki K, Akamizu T, Ishikawa N, Ito K, Ito K, Tamai H, Kuma K, Kubota S, Hiratani H, Tsuchiya T, Baba I, Ishikawa M, Tanaka M, Sakai K, Aoki M, Yamamoto K, Sasazuki T. SNPs in the promoter of a B cell-specific antisense transcript, SAS-ZFAT, determine susceptibility to autoimmune thyroid disease. Hum Mol Genet. 2004 Oct 1;13(19):2221-31. Epub 2004 Aug 04.
- Hiromatsu Y, Fukutani T, Ichimura M, Mukai T, Kaku H, Nakayama H, Miyake I, Shoji S, Koda Y, Bednarczuk T Interleukin-13 gene polymorphisms confer the susceptibility of Japanese populations to Graves' disease.. J Clin Endocrinol Metab. 2005 Jan;90(1):296-301.
- Ban Y, Tozaki T, Taniyama M, Tomita M, Ban Y. The Codon 620 Single Nucleotide Polymorphism of the Protein Tyrosine Phosphatase-22 Gene Does not Contribute to Autoimmune Thyroid Disease Susceptibility in the Japanese. Thyroid.2005 Oct;15(10):1115-8.
- Yamada H, Watanabe M, Nanba T, Akamizu T, Iwatani Y.The +869T/C polymorphism in the transforming growth factor-beta1 gene is associated with the severity and intractability of autoimmune thyroid disease.Clin Exp Immunol. 2008 Mar;151(3):379-82.
- Zeitlin AA, Simmonds MJ, Gough SC.Genetic developments in autoimmune thyroid disease: an evolutionary process.Clin Endocrinol (Oxf). 2007 Dec 13
- Sutherland A, Davies J, Owen CJ, Vaikkakara S, Walker C, Cheetham TD, James RA, Perros P, Donaldson PT, Cordell HJ, Quinton R, Pearce SH Genomic polymorphism at the interferon-induced helicase (IFIH1) locus contributes to Graves' disease susceptibility.J Clin Endocrinol Metab. 2007 Aug;92(8):3338-41.
- Liu N, Li X, Liu C, Zhao Y, Cui B, Ning G Hum Immunol. 2010 Feb 8 The association of interleukin-1alpha and interleukin-1beta polymorphisms with the risk of Graves' disease in a case-control study and meta-analysis.
- T aylor JC, Gough SC, Hunt PJ, Brix TH, Chatterjee K, Connell JM, Franklyn JA, Hegedus L, Robinson BG, Wiersinga WM, Wass JA, Zabaneh D, Mackay I, Weetman AP. A Genome-wide Screen in 1119 Relative Pairs with Autoimmune Thyroid Disease. J Clin Endocrinol Metab.2005 Nov 8;
- Berisso GA, van Lint MT, Bacigalupo A, Marmont AM. 1999 Adoptive autoimmune hyperthyroidism following allogeneic stem cell transplantation from an HLA-identical sibling with Graves' disease. Bone Marrow Transplantation. 23:1091-1092.
- Kisiel B, Bednarczuk T, Kostrzewa G, Kosińska J, Miśkiewicz P, Płazińska MT, Bar-Andziak E, Królicki L, Krajewski P, Płoski R.Polymorphism of the oestrogen receptor beta gene (ESR2) is associated with susceptibility to Graves' disease.Clin Endocrinol (Oxf). 2008 Mar;68(3):429-34
- Volpe R, Farid NR, Westarp CV, Row VV: The pathogenesis of Graves’ disease and Hashimoto’s thyroiditis. Clin Endocrinol (Oxf) 3:239, 1974.
- Lahl K, Loddenkemper C, Drouin C, Freyer J, Arnason J, Eberl G, Hamann A,Wagner H, Huehn J,Sparwasser T. Selective depletion of Foxp3+ regulatory T cells induces a scurfy-like disease.J Exp Med. 2007 Jan 22;204(1):57-63.
- Seddiki N, Santner-Nanan B, Martinson J, Zaunders J, Sasson S, Landay A,Solomon M, Selby W, Alexander SI, Nanan R, Kelleher A, Fazekas de St Groth B. Expression of interleukin (IL)-2 and IL-7 receptors discriminates between human regulatory and activated T cells.J Exp Med. 2006 Jul 10;203(7):1693-700.
- Banham AH, Powrie FM, Suri-Payer E. FOXP3+ regulatory T cells: Current controversies and future perspectives. Eur J Immunol. 2006 Nov;36(11):2832-6.
- Liu W, Putnam AL, Xu-Yu Z, Szot GL, Lee MR, Zhu S, Gottlieb PA, Kapranov P,Gingeras TR, Fazekas de St Groth B, Clayberger C, Soper DM, Ziegler SF,Bluestone JA. CD127 expression inversely correlates with FoxP3 and suppressive function of human CD4+ T reg cells. J Exp Med. 2006 Jul 10;203(7):1701-11.
- Raimondi G, Turner MS, Thomson AW, Morel PA. Naturally occurring regulatory T cells: recent insights in health and disease. Crit Rev Immunol. 2007;27(1):61-95.
- Beissert S, Schwarz A, Schwarz T. Regulatory T cells.J Invest Dermatol.2006 Jan;126(1):15-24.
- Pyzik M, Piccirillo CA TGF-{beta}1 modulates Foxp3 expression and regulatory activity in distinct CD4+ T cell subsets. J Leukoc Biol. 2007 May 2.
- Miyara M, Sakaguchi S. Natural regulatory T cells: mechanisms of suppression. Trends Mol Med. 2007 Mar;13(3):108-16.
- Pinkse GG, Tysma OH, Bergen CA, Kester MG, Ossendorp F, van Veelen PA,Keymeulen B, Pipeleers D, Drijfhout JW, Roep BO. Autoreactive CD8 T cells associated with beta cell destruction in type 1diabetes.Proc Natl Acad Sci U S A. 2005 Dec 20;102(51):18425-30.
- Alyanakian MA, You S, Damotte D, Gouarin C, Esling A, Garcia C, Havouis S, Chatenoud L, Bach JF.Diversity of regulatory CD4+T cells controlling distinct organ-specific autoimmune diseases. Proc Natl Acad Sci U S A. 2003 Dec 23;100(26):15806-11.
- You S, Leforban B, Garcia C, Bach JF, Bluestone JA, Chatenoud L. Adaptive TGF-beta-dependent regulatory T cells control autoimmune diabetes and are a privileged target of anti-CD3 antibody treatment. Proc Natl Acad Sci U S A. 2007 Apr 10;104(15):6335-40..PMID: 17389382
- You S, Leforban B, Garcia C, Bach JF, Bluestone JA, Chatenoud L. Adaptive TGF-beta-dependent regulatory T cells control autoimmune diabetes and are a privileged target of anti-CD3 antibody treatment. Proc Natl Acad Sci U S A. 2007 Apr 10;104(15):6335-40.
- Korn T, Reddy J, Gao W, Bettelli E, Awasthi A, Petersen TR, Bäckström BT, Sobel RA, Wucherpfennig KW, Strom TB, Oukka M, Kuchroo VK. Myelin-specific regulatory T cells accumulate in the CNS but fail to control autoimmune inflammation.Nat Med. 2007 Apr;13(4):423-31
- Alvarado-Sanchez B, Hernandez-Castro B, Portales-Perez D, Baranda L, Layseca-Espinosa E, Abud-Mendoza C, Cubillas-Tejeda AC, Gonzalez-Amaro R. Regulatory T cells in patients with systemic lupus erythematosus.J Autoimmun. 2006 Sep;27(2):110-8.
- Yu J, Heck S, Patel V, Levan J, Yu Y, Bussel JB, Yazdanbakhsh K Defective circulating CD25 regulatory T cells in patients with chronic immune thrombocytopenic purpura. Blood. 2008 Apr 17.
- Li X, Xiao BG, Xi JY, Lu CZ, Lu JH. Decrease of CD4(+)CD25(high)Foxp3(+) regulatory T cells and elevation of CD19(+)BAFF-R(+) B cells and soluble ICAM-1 in myasthenia gravis. Clin Immunol.2008 Feb;126(2):180-8.
- Nakano A, Watanabe M, Iida T, Kuroda S, Matsuzuka F, Miyauchi A, Iwatani Y. Apoptosis-induced Decrease of Intrathyroidal CD4(+)CD25(+) Regulatory T Cells.in Autoimmune Thyroid Diseases.Thyroid. 2007 Jan;17(1):25-31.
- Marazuela M, Garcia-Lopez MA, Figueroa-Vega N, de la Fuente H,Alvarado-Sanchez B, Monsivais- Urenda A, Sanchez-Madrid F, Gonzalez-Amaro R. Regulatory T cells in human autoimmune thyroid disease.J Clin Endocrinol Metab. 2006 Sep;91(9):3639-46. Epub 2006 Jun 27.
- Gangi E, Vasu C, Cheatem D, Prabhakar BS. IL-10-producing CD4+CD25+ regulatory T cells play a critical role in granulocyte-macrophage colony-stimulating factor-induced suppression of experimental autoimmune thyroiditis.J Immunol. 2005 Jun 1;174(11):7006-13.
- Molteni M, Rossetti C, Scrofani S, Bonara P, Scorza R, Kohn LD. Regulatory CD8+ T cells control thyrotropin receptor-specific CD4+ clones in healthy subjects.Cancer Detect Prev. 2003;27(3):167-74.
- Watanabe M, Yamamoto N, Maruoka H, Tamai H, Matsuzuka F, Miyauchi A, Iwatani Y. Independent involvement of CD8+ CD25+ cells and thyroid autoantibodies in disease severity of Hashimoto's disease.Thyroid. 2002 Sep;12(9):801-8.
- Vaidya B, Shenton BK, Stamp S, Miller M, Baister E, Andrews CD, Dickinson AJ, Perros P, Kendall-Taylor P. Thyroid. 2005 Sep;15(9):1073-8.Analysis of peripheral blood T-cell subsets in active thyroid-associated ophthalmopathy: absence of effect of octreotide-LAR on T-cell subsets in patients with thyroid-associated ophthalmopathy.
- Glick AB, Wodzinski A, Fu P, Levine AD, Wald DN. Impairment of regulatory T-cell function in autoimmune thyroid disease. Thyroid. 2013 Jul;23(7):871-8
- Klatka M, Grywalska E, Partyka M, Charytanowicz M, Kiszczak-Bochynska E, Rolinski J.Th17 and Treg cells in adolescents with Graves' disease. Impact of treatment with methimazole on these cell subsets. Autoimmunity. 2014 May;47(3):201-11
- Pan D, Shin YH, Gopalakrishnan G, Hennessey J, De Groot LJ: Regulatory T cells in Graves' disease. Clin Endocrinol (Oxf). 2009 Oct;71(4):587-93. Epub 2009 Feb 16.
- Mao C, Wang S, Xiao Y, Xu J, Jiang Q, Jin M, Jiang X, Guo H, Ning G, Zhang Y. Impairment of regulatory capacity of CD4+CD25+ regulatory T cells mediated by dendritic cell polarization and hyperthyroidism in Graves' disease.J Immunol. 2011 Apr 15;186(8):4734-43
- Pacini F, DeGroot LJ: Studies of immunoglobulin synthesis in cultures of peripheral T and B lymphocytes. Reduced T- suppressor cell activity in Graves' disease. Clin Endocrinol 18:219, 1983.
- Topliss DJ, Okita N, Lewis M, Row VV, Volpe R: Allosuppressor T lymphocytes abolish migration inhibition factor production in autoimmune thyroid disease: Evidence from radiosensitivity experiments. Clin Endocrinol 15:335, 1981.
- Sridama V, Pacini F, DeGroot LJ: Decreased suppressor T-lymphocytes in autoimmune thyroid diseases detected by monoclonal antibodies. J Clin Endocrinol Metab 54:316, 1982.
- Topliss D, How J, Lewis M, Row V, Volpe R: Evidence for cell-mediated immunity and specific suppressor T-lymphocyte dysfunction in Graves' disease and diabetes mellitus. J Clin Endocrinol Metab 57:700, 1983.
- Ando T, Imaizumi M, Graves PN, Unger P, Davies TF. Intrathyroidal fetal microchimerism in Graves’ disease. J Clin Endocrinol Metab 87:3315-3320, 2002.
- Bottazo GF, Pujol-Borrell R, Hanfusa T, Feldman F: Role of aberrant HLA-DR expression and antigen presentation in induction of endocrine autoimmunity. Lancet 2:1115, 1983.
- Matsunaga M, Eguchi K, Fukuda T, Kurata A, Tezuka H, Shimomura C, Otsubo T, Ishikawa N, Ito K, Nagataki S: Class II major histocompatibility complex antigen expression and cellular interactions in thyroid glands of Graves’ disease. J Clin Endocrinol Metab 62:723, 1986.
- Eguchi K, Otsubo T, Kawabe Y, Shimomura C, Ueki Y, Nakao H, Tezuka H, Matsunaga M, Fukuda T, Ishikawa N, Ito K, Nagataki S: Synergy in antigen presentation by thyroid epithelial cells and monocytes from patients with Graves’ disease. Clin Exp Immunol 72:84, 1988.
- Piccinini LA, MacKenzie WA, Platzer M, Davies TF: Lymphokine regulation of HLA-DR gene expression in human thyroid cell monolayers. J Clin Endocrinol Metab 64:543, 1987.
- Mukuta T, Arreaza G, Nishikawa M, Resetkova E, Jamieson C, Tamai H, Volpe R: Thyroid xenografts from patients with Graves’ disease in severe combined immunodeficient mice and NIH-beige-nude-xid mice. Clin Investigative Med 20:5-15, 1997.
- Leclere J, Bene MC, Duprez A, Faure G, Thomas JL, Vignaud JM, Burlet C: Behavior of thyroid tissue from patients with Graves’ disease in nude mice. J Clin Endocrinol Metab 59:175, 1984.
- Markmann J, Lo D, Naji A, Palmiter RD, Brinster RL, Heber Katz E: Antigen presenting function of class II MHC expressing pancreatic beta cells. Nature 336:476-479, 1988.
- Iversen K: Temporary rise in the frequency of thyrotoxicosis in Denmark 1941-1945. Copenhagen, Rosenkilde and Bagger, 1948.
- Chiovato L, Pinchera A: Stressful life events and Graves’ disease. Europ J Endocrinol 134:680-682, 1996.
- Radosavljevic VR, Jankovic SM, Marinkovic JM: Stressful life events in the pathogenesis of Graves’ disease. Europ J Endocrinol 134:699-701, 1996.
- Winsa B, Adami HO, Bergstrom R, Gamstedt A, Dahlberg PA, Adamson U, Jansson R, Karlsson FA: Stressful life events and Graves' disease. Lancet 338:1475-1479, 1991.
- Matos-Santos A, Nobre EL, Costa JG, Nogueira PJ, Macedo A, Galvao-Teles A, de Castro JJ. Relationship between the number and impact of stressful life events and the onset of Graves’ disease and toxic nodular goiter. Clin Endocrinol 55:15-19, 2001.
- Landsberg L: Catecholamines and hyperthyroidism. Clin Endocrinol Metab 6:697, 1977.
- Bruun E: Exophthalmic goiter developing after treatment with thyroid preparations. Acta Med Scand 122:13, 1945.
- Vagenakis AG, Wang CA, Bruger A, Maloof F, Braverman LE, Ingbar SH: Iodide-induced thyrotoxicosis in Boston. N Engl J Med 287:523, 1972.
- Stanbury JB, Ermans AE, Bourdoux P, Todd C, Oken E, Tonglet R, Vidor G, Braverman LE, Medeiros-Neto G: Iodine-induced hyperthyroidism: Occurrence and epidemiology. Thyroid 8:83-100, 1998.
- Vidor GI, Stewart JC, Wall JR, Wangel A, Hetzel BS: Pathogenesis of iodine-induced thyrotoxicosis: Studies in northern Tasmania. J Clin Endocrinol Metab 37:901, 1973. Nilsson G: Self-limiting episodes of jodbasedow. Acta Endocrinol 74:475, 1973.
- Stewart JC, Vidor GI, Butterfield IH, Hetzel BS: Epidemic thyrotoxicosis in northern Tasmania. Studies of clinical features and iodine nutrition. Aust NZ J Med 3:203, 1971.
- Boukis IA, Koutras DA, Souvantzoglou A, Evangelopolou A, Vrontakis A, Moulopoulos SD: Thyroid hormone and immunological studies in endemic goiter. J Clin Endocrinol Metab. 57:859-862, 1983.
- Rasooly L, Burek CL, Rose NR: Iodine-induced autoimmune thyroiditis in NOD-H-2h4 mice. Clin Immunol Immunopathol 81:287-292, 1996.
- Allen EM, Appel MC, Braverman LE: The effect of iodine ingestion on the development of spontaneous lymphocytic thyroiditis in the diabetes prone BB/W rat. Endocrinol 118:1977-1981, 1986.
- Plummer HS: Results of administering iodine to patients having exophthalmic goiter. J Amer Med Assn 80:1955, 1923.
- Feinberg WD, Hoffman DL, Owen CA: The effects of varying amounts of stable iodide on the function of the human thyroid. J Clin Endocrinol Metab 19:567, 1959.
- Greer MA, DeGroot LJ: The effect of stable iodide thyroid secretion in man. Metabolism 5:682, 1956. Ochi Y, Hachiya T, Yoshimura M, Shiomi K, Miyazaki T: Inhibitory effect of excess iodide on Graves' disease. Iodine Metab Thyroid Function 6:127, 1973.
- Buhler UK, DeGroot LJ: Effects of stable iodine on thyroid iodine release. J Clin Endocrinol Metab 29:1546, 1969.
- Schuppert F, Taniguchi S-I, Schroder S, Dralle H, Von Zur Muhlen A, Kohn LD: In vivo and in vitro evidence for iodide regulation of major histocompatibility complex class I and class II expression in Graves’ disease. J Clin Endocrinol Metab 81:3622-3628, 1996.
- Salvi M, Pedrazzoni M, Girasole G, Giuliani N, Minelli R, Wall JR, Roti E. Serum concentrations of proinflammatory cytokines in Graves' disease: effect of treatment, thyroid function, ophthalmopathy and cigarette smoking. Europ J Endocrinol 143:197-202, 2000.
- Wegelius O, Naumann J, Brunish R: Uptake of 35S labeled sulfate in the harderian and the ventral lachrymal glands of the guinea pig during stimulation with ophthalmotrophic pituitary agents. Acta Endocrinol 30:53, 1959.
- Lamberg BA, Ripatti J, Gordin A, Juustila H, Sivula A, Bjorkesten G: Chromophobe pituitary adenoma with acromegaly and TSH-induced hyperthyroidism associated with parathyroid adenoma. Acta Endocrinol 60:157, 1969.
- Weintraub BD, Gershengorn MC, Kourides IA, Fein H: Inappropriate secretion of thyroid-stimulating hormone. Ann Intern Med 95:339, 1981.
- Duprez L, Parma J, Van Sande J, Allgeier A, Leclere J, Schvartz C, Delisle M-J, Decoulx M, Orgiazzi J, Dumont J, Vassart G: Germline mutations in the thyrotropin receptor gene cause non-autoimmune autosomal dominant hyperthyroidism. Nature Genetics 7:396-401, 1994.
- Iversen K: Temporary rise in the frequency of thyrotoxicosis in Denmark 1941-1945. Copenhagen, Rosenkilde and Bagger, 1948.
- Kasagi K, Konishi J, Endo K, Mori T, Nagahara K, Makimoto K, Kuma K, Torizuka K: Adenylate cyclase activity in thyroid tissue from patients with untreated Graves' disease. J Clin Endocrinol Metab 51:492, 1980.
- Stanbury JB, Janssen MA: The iodinated albumin-like component of the plasma of thyrotoxic patients. J Clin Endocrinol Metab 22:978, 1962.
- Farran HEA, Shalom ES: Effect of L-tyrosine upon the protein bound iodine in thyrotoxicosis. J Clin Endocrinol Metab 26:918, 1966.
- Sterling K, Chodos RB: Radiothyroxine turnover studies in myxedema, thyrotoxicosis, and hypermetabolism without endocrine disease. J Clin Invest 35:806, 1956.
- Ingbar SH, Freinkel N: Studies on thyroid function and the peripheral metabolism of 131I-labeled thyroxine in patients with treated Graves' disease. J Clin Invest 37:1603, 1958.
- Uller RP, Van Herle AJ: Effect of therapy on serum thyroglobulin levels in patients with Graves' disease. J Clin Endocrinol Metab 46:747, 1978.
- Greer MA, Smith GE: Method for increasing the accuracy of the radioiodine uptake as a test for thyroid function by the use of desiccated thyroid. J Clin Endocrinol Metab 14:1374, 1954.
- Werner SC, Spooner M: A new and simple test for hyperthyroidism employing L-triiodothyronine and the twenty-four hour 131I uptake methods. Bull NY Acad Med 31:137, 1955.
- Clague R, Mukhtar ED, Pyle GA, Nutt J, Clark F, Scott M, Evered D, Smith BR, Hall R: Thyroid-stimulating immunoglobulins and the control of thyroid function. J Clin Endocrinol Metab 43:550, 1976.
- Lamberg BA, Ard A, Saarinen P, Totterman T, Makinen T: Response to TRH, serum thyroid hormone concentration, and serum markers of autoimmunity after antithyroid therapy in Graves' disease. J Endocrinol Invest 1:9, 1978.
- Chopra IJ, Chopra U, Orgiazzi J: Abnormalities of hypothalamo-hypophyseal-thyroid axis in patients with Graves' ophthalmopathy. J Clin Endocrinol Metab 37:955, 1973.
- Franco PS, Hershman JM, Haigler ED, Pittman JA Jr: Response to thyrotropin-releasing hormone compared with thyroid suppression tests in euthyroid Graves' disease. Metabolism 22:1357, 1973.
- Plummer HS: Results of administering iodine to patients having exophthalmic goiter. J Amer Med Assn 80:1955, 1923.
- Raben MS: The paradoxical effects of thiocyanate and of thyrotropin on the organic binding of iodine by the thyroid in the presence of large amounts of iodide. Endocrinology 45:296, 1949.
- Paris J, McConahey WM, Tauxe WN, Woolner LB, Bahn RC: The effect of iodides on Hashimoto's thyroiditis. J Clin Endocrinol Metab 21:1037, 1961.
- Suzuki H, Mashimo K: Significance of the iodide-perchlorate discharge test in patients with 131I-treated and untreated hyperthyroidism. J Clin Endocrinol Metab 34:332, 1972.
- Braverman LE, Woeber KA, Ingbar SH: Induction of myxedema by iodide in patients euthyroid after radioiodine or surgical treatment of diffuse toxic goiter. N Engl J Med 281:816, 1969.
- Ochi Y, Hachiya T, Yoshimura M, Shiomi K, Miyazaki T: Inhibitory effect of excess iodide on Graves' disease. Iodine Metab Thyroid Function 6:127, 1973.
- Buhler UK, DeGroot LJ: Effects of stable iodine on thyroid iodine release. J Clin Endocrinol Metab 29:1546, 1969.
- DeGroot L: Kinetic analysis of iodine metabolism. J Clin Endocrinol Metab 26:149, 1966.
- Furszyfer J, Kurland LT, McConahey WM, Elveback LR: Graves' disease in Olmsted County, Minnestoa, 1935 through 1967. Mayo Clin Proc 45:636, 1970.
- Tunbridge WMG, Evered DE, Hall R, Appleton D, Brewis M, Clark F, Grimley-Evans J, Young E, Bird T, Smith PA: The spectrum of thyroid disease in a community: The Wickham Survey. Clin Endocrinol 7:481, 1977.
- Vanderpump MPJ et al: Incidence of thyroid disorders in the community based on a twenty year follow-up of the Whickham survey population. Clinical Endocrinol 43:55-68, 1995.
- Hollowell JG, Staehling NW, Flanders WD, Hannon WH, Gunter EW, Spencer CA, Braverman LE. Serum TSH, T(4), and thyroid antibodies in the United States population (1988 to 1994): National Health and Nutrition Examination Survey (NHANES III). J Clin Endocrinol Metab. 2002 Feb;87(2):489-99
- Heimann P: Ultrastructure of human thyroid. Acta Endocrinol 53(suppl 110):5, 1966.
- Adams RD, Denny-Brown D, Pearson CM: In Diseases of Muscle. New York, Harper & Row Publishers Inc, 1962.
- Bostrom H, Hed R: Thyrotoxic myopathy and polymyositis in elderly patients: Differential-diagnostic viewpoints. Acta Med Scand 162:225, 1958.
- McEachern D, Ross WD: Chronic thyrotoxic myopathy: Report of three cases with review of previously reported cases. Brain 65:181, 1942.
- Askanazy M, Rutishauser E: Die Knochen der Basedow-Kranken: Beitrag zur latenten Osteodystrophia fibrosa. Virchows Arch 291:653, 1933.
- Dudgeon LS, Urquhart AL: Lymphorrhages in the muscles in exophthalmic goiter. Grain 49:182, 1926.
- Naffziger HC: Progressive exophthalmos after thyroidectomy. West J Surg Obstet Gynecol 40:530, 1932.
- Rundle FF, Pochin EE. Orbital tissues in thyrotoxicosis: Quantitative analysis relating to exophthalmos. Clin Sci 5:51, 1944.
- Terndrup TE, Heisig DG, Garceau JP. Sudden death associated with undiagnosed Graves' disease. J Emergency Med 8:553-555, 1990.
- Ezrin C, Swanson HE, Humphrey JG, Dawson JW, Hill FM: The cells of the human adenohypophysis in thyroid disorders. J Clin Endocrinol Metab 19:958, 1959.
- Scheithauer BW, Kovacs KT, Young Jr WF, Randall RV: The pituitary gland in hyperthyroidism. Mayo Clin Proc 67:22- 26, 1992.
- Beaver DC, Pemberton J de J: The pathologic anatomy of the liver in exophthalmic goiter. Ann Intern Med 7:687, 1933.
- Movitt ER, Gerstl B, Davis AE: Needle biopsy in thyrotoxicosis. Arch Intern Med 91:729, 1953.
- Piper J, Poulsen E: Liver biopsy in thyrotoxicosis. Acta Med Scand 127:439, 1947.
- Bergman TA, Mariash CN, Oppenheimer JH: Anterior mediastinal mass in a patient with Graves' disease. J Clin Endocrinol Metab 55:587-588, 1982.
- Fallon MD, Perry III HM, Bergfeld M, Droke D, Teitelbaum SL, Avioli LV: Exogenous hyperthyroidism with osteoporosis. Arch Intern Med 143:442-444, 1983.
- Follis RH: Skeletal changes associated with hyperthyroidism. Bull Johns Hopkins Hosp 92:405, 1953.
- Siddiqi A, Burrin JM, Noonan K, James I, Wood DF, Price CP, Monson JP. A longitudinal study of markers of bone turnover in Graves’ disease and their value in predicting bone mineral density. J Clin Endocrinol Metab 82:753-759, 1997.
- Shulz H, Low H, Ernster L, Sjostrand FS: Electronenmikroskopische Studien an Leberschnitten von Thyroxin-behandelten Ratten, in Sjostrand FS, Rhodin J (eds): European Regional Conference on Electron Microscopy, 1st Proceedings of the Stockholm Conference, Sept. 1956, New York, Academic Press Inc, 1957, p 134.
- White WH: On prognosis of secondary symptoms of exophthalmic goiter. Br Med J 2:151, 1886.
- Sattler H: In Marchand GW, Marchand JF (trans): Basedow's Disease. New York, Grune & Stratton Inc. 1952.
- Hegedus L, Hansen JM, Karstrup S: High incidence of normal thyroid gland volume in patients with Graves' disease. Clin Endocrinol 19:603-607, 1983.
- Werner S: Classification of the eye changes of Graves' disease. J Clin Endocrinol Metab 29:982, 1969.
- Leblond CP, Fertman MB, Puppel ID, Curtis GM: Radioiodine autography in studies of human goitrous thyroid glands. Arch Pathol Lab Med 41:510, 1946.
- Mahaux JE, Chamla-Soumenkoff J, Delcourt R, Levin S: Painful enlargement of left subtrapezoid lymph nodes in Graves' disease. Br Med J 1:384, 1971.
- Rumbyrt JS, Schocket AL. Chronic urticaria and thyroid disease.Immunol Allergy Clin North Am. 2004 May;24(2):215-23, vi. Review.
- Brownlie BE, Rae AM, Walshe JW, Wells JE. Psychoses associated with thyrotoxicosis - 'thyrotoxic psychosis.' A report of 18 cases, with statistical analysis of incidence. Europ J Endocrinol 142:438-444, 2000.
- Perrild H, Hansen JM, Arnung K, Olsen PZ, Danielsen U: Intellectual impairment after hyperthyroidism. Acta Endocrinol 112:185-191, 1986.
- Feibel JH, Campa JF: Thyrotoxic neuropathy (Basedow's paraplegia). J Neurol Neurosurg Psychiatry 39:491, 1976.
- Condon JV, Becka DR, Gibbs FA: Electroencephalographic abnormalities in hyperthyroidism. J Clin Endocrinol Metab 14:1511, 1954.
- Shaw PJ, Walls TJ, Newman PK, Cleland PG, Cartlidge NE. 1991 Hashimoto's encephalopathy: a steroid-responsive disorder associated with high antithyroid antibody titers-report of 5 cases. Neurology 41:228-233.
- Canton A, de Fabregas O, Tintore M, Mesa J, Codina A, Simo R. Encephalopathy associated to autoimmune thyroid disease: a more appropriate term for an underestimated condition? J Neurol Sci 176:65-69, 2000.
- Woodbury DM, Hurley RE, Lewis NG, McArthur MW, Copeland WW, Kirschrink JF, Goodman LS: Effect of thyroxine, thyroidectomy, and 6-N-propyl-2-thiouracil on brain function. J Pharmacol Exp Ther 106:331, 1952.
- Ravera JJ, Cervino JM, Fernandez G, Ferrari Foreade A, Malosetti H, Muxi F, MaggioloJ, Mussio Fournier JC, Rawak R: Two cases of Graves' disease with signs of a pyramidal lesion: Improvement in neurologic signs during treatment with antithyroid drugs. J Clin Endocrinol Metab 20:876, 1960.
- Swanson JW, Kelly, Jr. JJ, McConahey WM: Neurologic aspects of thyroid dysfunction. Mayo Clin Proc 56:504-512, 1981.
- Waldenstrom J: Acute thyrotoxic encephalomyopathy: Its cause and treatment. Acta Med Scand 121:251, 1945.
- Feibel JH, Campa JF: Thyrotoxic neuropathy (Basedow's paraplegia). J Neurol Neurosurg Psychiatry 39:491-497, 1976.173a. Bernal J. Action of thyroid hormone in brain. J Endocrinol Invest 25:268-288, 2002.
- Sensenbach W, Madison L, Eisenberg S, Ochs L: The cerebral circulation and metabolism in hyperthyroidism and myxedema. J Clin Invest 33:1434, 1954.174a.
- Whitfield AGW, Hudson WA: Chronic thyrotoxic myopathy. Q J Med 30:257, 1961.
- Gold HK, Spann JF Jr, Braunwald E: Effects of alterations in the thyroid state on the intrinsic contractile properties of isolated rat skeletal muscle. J Clin Invest 49:849, 1970.
- Sanderson KV, Adey WR: Electromyographic and endocrine studies in chronic thyrotoxic myopathy. J Neurol Neurosurg Psychiatry 15:200, 1952.
- Zurcher RM, Horber FF, Grunig BE, Frey FJ: Effect of thyroid dysfunction on thigh muscle efficiency. J Clin Endocrinol Metab 69:1082, 1989.
- Erkintalo M, Bendahan D, Mattei J-P, Fabreguettes C, Vague P, Cozzone PJ. Reduced metabolic efficiency of skeletal muscle energetics in hyperthyroid patients evidenced quantitatively by in vivo phosphorus-31 magnetic resonance spectroscopy. Metabolism 47:769-776, 1998.
- Fitch CD, Coker R, Dinning JS: Metabolism of creatine I-14C by Vitamin E deficient and hyperthyroid rats. Am J Physiol 198:1232, 1960.
- Thorn G: Creatine studies in thyroid disease. Endocrinology 20:628, 1936.
- Drachman DB: Myasthenia gravis and the thyroid gland. N Engl J Med 266:330, 1962.
- Engel AG: Thyroid function and periodic paralysis. Am J Med 30:327, 1961.
- Fisher J: Thyrotoxic periodic paralysis with ventricular fibrillation. Arch Intern Med 142:1362-1364, 1982.
- Fraser SA, Anderson JB, Smith DA, Wilson GM: Osteoporosis and fractures following thyrotoxicosis. Lancet 1:981, 1971.
- Rosen CJ, Adler RA. Longitudinal changes in lumbar bone density among thyrotoxic patients after attainment of euthyroidism. J Clin Endocrinol Metab 75:1531-1534, 1992.
- Langdahl BL, Loft AGR, Eriksen EF, Mosekilde L, Charles P. Bone mass, bone turnover, body composition, and calcium homeostasis in former hyperthyroid patients treated by combined medical therapy. Thyroid 6:161, 1996.
- Vestergaard, P; Mosekilde, L. Hyperthyroidism, bone mineral, and fracture risk – a meta-analysis. Thyroid 13 585 2003
- Jodar E, Martinez-Diaz-Guerra G, Azriel S, Hawkins F. Bone mineral density in male patients with L-thyroxine suppressive therapy and Graves’ disease. Calcified Tissue International 69:84-87, 2001.
- Uzzan B, Campos J, Cucherat M, Nony P, Boissel JP, Perret GY. Effects on bone mass of long term treatment with thyroid hormones: A meta-analysis. J Clin Endocrinol Metab 81:4278-4289, 1996.
- Krane SM, Brownell GL, Stanbury JB, Corrigan H: The effect of thyroid disease on calcium metabolism in man. J Clin Invest 35:874, 1956.
- Harvey RD, McHardy KC, Reid IW, Paterson F, Bewsher PD, Duncan A, Robins P: Measurement of bone collagen degradation in hyperthyroidism and during thyroxine replacement therapy using pyridinium cross-links as specific urinary markers. J Clin Endocrinol Metab 72:1189-1194, 1991.
- Sataline LR, Powell C, Hamwi GJ: Suppression of the hypercalcemia of thyrotoxicosis by corticosteroids. N Engl J Med 267:646, 1962.
- Sallin O: Hypercalcemic nephropathy in thyrotoxicosis. Acta Endocrinol 29:425, 1958.
- Epstein FH, Freedman LR, Levitin H: Hypercalcemia, nephrocalcinosis and reversible renal insufficiency associated with hyperthyroidism. N Engl J Med 258:782, 1958.
- Bortz W, Eisenberg E, Bowers CY, Pout M: Differentiation between thyroid and parathyroid causes of hypercalcemia. Ann Intern Med 54:610, 1961.
- David NJ, Verner JV, Engel FL: Diagnostic spectrum of hypercalcemia: Case reports and discussion. Am J Med 33:88, 1962.
- Kleeman CR, Tuttle S, Bassett SH: Metabolic observations in a case of thyrotoxicosis with hypercalcemia. J Clin Endocrinol Metab 18:477, 1958.
- Lukert BP, Higgins JC, Stoskopf MM: Serum osteocalcin is increased in patients with hyperthyroidism and decreased in patients receiving glucocorticoids. J Clin Endocrinol Metab 62:1056, 1986.
- Cooper DS, Kaplan MM, Ridgway EC, Maloof F, Daniels GH: Alkaline phosphatase isoenzyme patterns in hyperthyroidism. Ann Intern Med 90:164, 1979.
- Garrel DR, Delmas PD, Malaval L, Tourniaire J: Serum bone gla protein: A marker of bone turnover in hyperthyroidism. J Clin Endocrinol Metab 62:1052, 1986.
- Bouillon R, DeMoor P: Parathyroid function in patients with hyper- or hypothyroidism. J Clin Endocrinol Metab 38:999, 1974.
- Bouillon R, Muls E, DeMoor P: Influence of thyroid function on the serum concentration of 1,25-dihydroxyvitamin D3. J Clin Endocrinol Metab 51:793, 1980.
- Peerenboom H, Keck E, Kruskemper HL, Strohmeyer G: The defect of intestinal calcium transport in hyperthyroidism and its response to therapy. J Clin Endocrinol Metab 59:936, 1984.
- Rude RK, Oldham SB, Singer FR, Nicoloff JT: Treatment of thyrotoxic hypercalcemia with propranolol. N Engl J Med 294:431, 1976.
- Rosen HN, Moses AC, Gundberg C, Kung VT, Seyedin SM, Chen T, Holick M, Greenspan SL. Therapy with parenteral pamidronate prevents thyroid hormone-induced bone turnover in humans. J Clin Endocrinol Metab 77:664-669, 1993.
- Jodar E, Munoz-Torres M, Escobar-Jimenez F, Quesada M, Luna JD, Olea N. Antiresorptive therapy in hyperthyroid patients: longitudinal changes in bone and mineral metabolism. J Clin Endocrinol Metab 82:1989-1994, 1997.
- Barsotti MM, Targovnik JH, Verso TA: Thyrotoxicosis, hypercalcemia, and secondary hyperparathyroidism. Arch Intern Med 139:661, 1979
- Siafakas NM, Milona I, Salesiotou V, Filaditaki V, Tzanakis N, Bouros D. Respiratory muscle strength in hyperthyroidism before and after treatment. Amer Rev Respiratory Dis 146:1025-1029, 1992.
- Siafakas NM, Milona I, Salesiotou V, Filaditaki V, Tzanakis N, Bouros D. Respiratory muscle strength in hyperthyroidism before and after treatment. Amer Rev Respiratory Dis 146:1025-1029, 1992.
- Massey DG, Becklake MR, McKenzie JM, Bates DV: Circulatory and ventilatory response to exercise in thyrotoxicosis. N Engl J Med 276:1104, 1967.
- Stein M, Kinbel P, Johnson RL: Pulmonary function in hyperthyroidism. J Clin Invest 40:348, 1961.
- Hamolsky MW: Asthma and hyperthyroidism. J Allergy Clin Immunol 49:348, 1972.
- Reynolds J, Woody HB: Thyrotoxic mitral regurgitation. Am J Dis Child 122:544, 1971.
- Auer J, Scheibner P, Mische T, Langsteger W, Eber O, Eber B. Subclinical hyperthyroidism as a risk factor for atrial fibrillation. American Heart Journal 142:838-842, 2001.
- Sandler G, Wilson GM: The nature and prognosis of heart disease in thyrotoxicosis. Q J Med 28:347, 1959.
- Graettinger JS, Muenster JJ, Selverstone LA, Campbell JA: A correlation of clinical and hemodynamic studies in patients with hyperthyroidism with and without congestive heart failure. J Clin Invest 38:1316, 1959.
- Blizzard JJ, Rupp JJ: Prolongation of the P-R interval as a manifestation of thyrotoxicosis. J Amer Med Assn 173:1845, 1960.
- Resnekov L, Falicow R: Thyrotoxicosis and lactate-producing angina pectoris with normal coronary arteries. Brit Heart J 39:1051, 1977.
- Marti V, Ballester M, Rigla M, Narula J, Berna L, Pons-Llado G, Carrio I, Carreras F, Webb SM. Myocardial damage does not occur in untreated hyperthyroidism unless associated with congestive heart failure. Amer Heart J 134:1133-1137, 1997.
- Choi YH, Chung JH, Bae SW, Lee WH, Jeong EM, Kang MG, Kim BJ, Kim KW, Park JE .Severe coronary artery spasm can be associated with hyperthyroidism. Coron Artery Dis. 2005 May;16(3):135-9.
- Kotler N, Kyriakos M, Bouchard J, Warbasse JR: Myocardial infarction associated with thyrotoxicosis. Arch Intern Med 132:723, 1973.
- Rowe GG, Huston JH, Weinstein AB, Tuchman H, Brown JF, Crumpton CW: The hemodynamics of thyrotoxicosis in man with special reference to coronary blood flow and myocardial oxygen metabolism. J Clin Invest 35:272, 1956.
- Rodbard D, Fugita T, Rodbard S: Estimation of thyroid function by timing the arterial sounds. J Amer Med Assn 201:884, 1967.
- Buccino RA, Spann JF Jr, Sonnenblick EH, Braunwald E: Effect of thyroid state on myocardial contractility. Endocrinology 82:191, 1968.
- Osman F, Gammage MD, Sheppard MC, Franklyn JA. Cardiac dysrhythmias and thyroid dysfunction: the hidden menace? J Clin Endocrinol Metab 87:963-967, 2002.
- Woeber KA. Thyrotoxicosis and the heart. N Engl J Med 327:94-98, 1992.
- Kahaly GJ, Nieswandt J, Mohr-Kahaly S. Cardiac risks of hyperthyroidism in the elderly. Thyroid 8:1165, 1998.
- Mintz G, Pizzarello R, Klein I: Enhanced left ventricular diastolic function in hyperthyroidism: Noninvasive assessment and response to treatment. J Clin Endocrinol Metab 73:146-150, 1991.
- Valcavi R, Menozzi C, Roti E, Zini M, Lolli G, Roti S, Guiducci U, Portioli I. Sinus node function in hyperthyroid patients. J Clin Endocrinol Metab 75:239-242, 1992.
- Smolenski RT, Yacoub MH, Seymour AM. Hyperthyroidism increases adenosine transport and metabolism in the rat heart. Molecul Cellul Biochem 143:143-149, 1995.
- Dillmann WH: Biochemical basis of thyroid hormone action in the heart. Amer J Med 88:626-630, 1990
- Schmidt BMW, Martin N, Georgens AC, Tillmann H-C, Feuring M, Christ M, Wehling M. Nongenomic cardiovascular effects of triiodothyronine in euthyroid male volunteers. J Clin Endocrinol Metab 87:1681-1686, 2002. .
- Fatourechi V, Edwards WD. Graves' disease and low-output cardiac dysfunction: Implications for autoimmune disease in endomyocardial biopsy tissue from eleven patients. Thyroid 10:601, 2000.
- Pietras RJ, Real MA, Poticha GS, Bronsky D, Waldstein SS: Cardiovascular response in hyperthyroidism. The influence of adrenergic-receptor blockade. Arch Intern Med 129:426, 1972.
- deGroot WJ, Leonard JJ, Paley HW, Johnson JE, Warren JV: The importance of autonomic integrity in maintaining the hyperkinetic circulating dynamics of human hyperthyroidism. J Clin Invest 40:1033, 1961.
- McDevitt DG, Shanks RG, Hadden DR, Montgomery DAD, Weaver JA: The role of the thyroid in the control of the heart-rate. Lancet 1:997, 1968.
- Tse J, Wrenn RW, Kuo JF: Thyroxine-induced changes in characteristics and activities of beta-adrenergic receptors and adenosine 3',5'-monophosphate and guanosine 3',5'-monophosphate systems in the heart may be related to reputed catecholamine supersensitivity in hyperthyroidism. Endocrinology 107:6, 1980.
- Williams LT, Lefkowitz RJ, Watanabe AM, Hathaway DR, Besch H Jr: Thyroid hormone regulation of beta-adrenergic receptor number. J Biol Chem 252:2787, 1977.
- Ginsberg AM, Clutter WE, Shah SD, Cryer PE: Triiodothyro- nine-induced thyrotoxicosis increases mononuclear leukocyte beta-adrenergic receptor density in man. J Clin Invest 67:1785, 1981.
- Nilsson OR, Anderson RGG, Karlberg BE: Effects fo propranolol and atenolol on plasma and urinary cyclic adenosine 3',5'-monophosphate in hyperthyroid patients. Acta Endocrinol 94:38, 1980.
- Guttler RB, Croxon MS, De Quattro VL, Warren DW, Otis CL, Nicoloff JT: Effects of thyroid hormone on plasma adenosine 3',5'-monophosphate productin in man. Metabolism 26:1155, 1977.
- Levey GS, Klein I: Catecholamine-thyroid hormone interactions and the cardiovascular manifestations of hyperthyroidism. Amer J Med 88:642-646, 1990.
- Biondi B, Fazio S, Cuocolo A, Sabatini D, Nicolai E, Lombardi G, Salvatore M, Sacca L. Impaired cardiac reserve and exercise capacity in patients receiving long-term thyrotropin suppressive therapy with levothyroxine. J Clin Endocrinol Metab 81:4224-4228, 1996
- Smit JW, Eustatia-Rutten CF, Corssmit EP, Pereira AM, Frolich M, Bleeker GB, Holman ER, van der Wall EE, Romijn JA, Bax JJ. Reversible diastolic dysfunction after long-term exogenous subclinical hyperthyroidism: a randomized, placebo-controlled study. J Clin Endocrinol Metab. 2005 Nov;90(11):6041-7
- Nightingale S, Vitek PJ, Himsworth RL: The hematology of hyperthyroidism. Q J Med 47:35, 1978.
- Popovic WJ, Brown JE, Adamson JW: The influence of thyroid hormones on in vitro erythropoiesis. J Clin Invest 60:907, 1977.
- Rivlin RS, Wagner HN Jr: Anemia in hyperthyroidism. Ann Intern Med 70:507, 1969.
- Viherkoski M, Lamberg BA: The glucose-6-phosphate dehydrogenase activity (G-6-PD) of the red blood cells in hyperthyroidism and hypothyroidism. Scand J Clin Lab Invest 25:137, 1970.
- Irvine WJ, Wu FCW, Urbaniak SJ, Toolis F: Peripheral blood leukocytes in thyrotoxicosis (Graves' Disease) as studied by conventional light microscopy. Clin Exp Immunol 27:216, 1977.
- Hertz S, Lerman J: The blood picture in exophthalmic goiter and its changes resulting from iodine and operation. J Clin Invest 11:1179, 1932.
- Lima CS, Wittmann DE, Castro V, Tambascia MA, Lorand-Metze I, Saad ST, Costa FF ..Pancytopenia in untreated patients with graves' disease. Thyroid. 2006 Apr;16(4):403-409.
- Lamberg BA, Kivikangas V, Pelkonen R, Viopio P: Thrombocytopenia and decreased life-span of thrombocytes in hyperthyroidism. Ann Clin Res 3:98, 1971.
- Adrouny A, Sandler RM, Carmel R: Variable presentation of thrombo- cytopenia in Graves' disease. Arch Intern Med 142:1460-1464, 1982.
- Verberne HJ, Fliers E, Prummel MF, Stam J, Brandjes DP, Wiersinga WM. Thyrotoxicosis as a predisposing factor for cerebral venous thrombosis. Thyroid 10:607, 2000.
- Bergman TA, Mariash CN, Oppenheimer JH: Anterior mediastinal mass in a patient with Graves' disease. J Clin Endocrinol Metab 55:587, 1982.
- Okumura M, Hidaka Y, Kuroda S, Takeoka K, Tada H, Amino N. Increased serum concentration of soluble CD30 in patients with Graves’ disease and Hashimoto’s thyroiditis. J Clin Endocrinol Metab 82:1757-1760, 1997
- Lerman J, Means JH: The gastric secretion in exophthalmic goiter and myxedema. J Clin Invest 11:167, 1932.
- Berryhill WR, Williams HA: A study of the gastric secretion in hyper- thyroidism before and after operation. J Clin Invest 11:753, 1932.
- Siurala M, Lamberg BA: Stomach in thyrotoxicosis. Acta Med Scand 165:181, 1959.
- Seino Y, Matsukura S, Miyamoto Y, Goto Y, Taminato T, Imura H: Hyper- gastrinemia in hyperthyroidism. J Clin Endocrinol Metab 43:852, 1976.
- Wegener M, Wedmann B, Langhoff T, Schaffstein J, Adamek R. Effect of hyperthyroidism on the transit of a caloric solid-liquid meal through the stomach, the small intestine, and the colon in man. J Clin Endocrinol Metab 75:745-749, 1992.
- Lamberg BA, Gordin R: Liver function in thyrotoxicosis. Acta Endocrinol 15:82, 1954.
- Thompson Jr P, Strum D, Boehm T, Wartofsky L: Abnormalities of liver function tests in thyrotoxicosis. Military Medicine 548-551, 1978.
- Klion FM, Segal R, Schaffner F: The effect of altered thyroid function on the ultrastructure of the human liver. Am J Med 50:317, 1971.
- Greenberger NJ, Milligan FD, DeGroot LJ, Isselbacher KJ: Jaundice and thyrotoxicosis in the absence of congestive heart failure. Am J Med 36:840, 1964.
- Ford RV, Owens JC, Curd GW Jr, Moyer JH, Spurr CL: Kidney function in various thyroid states. J Clin Endocrinol Metab 21:548, 1961.
- Epstein FH, Rivera MJ: Renal concentrating ability in thyrotoxicosis. J Clin Endocrinol Metab 18:1135, 1958.
- Huth EJ, Maycock RL, Kerr RM: Hyperthyroidism associated with renal tubular acidosis. Am J Med 26:818, 1959.
- Goldsmith RE, Sturgis SH, Lerman J, Stanbury JB: The menstrual pattern in thyroid disease. J Clin Endocrinol Metab 12:846, 1952.
- Bray GA, Jacobs HS: Thyroid activity and other endocrine glands. In: Handbook of Physiology, Endocrinology, Washington, DC, American Physiology Society, 1974, vol III, p 413.
- Freedberg IM, Hamolsky MW, Freedberg AS: The thyroid gland in pregnancy. N Engl J Med 256:505, 1957.
- Abalovich M, Amino N, Barbour LA, Cobin RH, De Groot LJ, Glinoer D, Mandel SJ, Stagnaro-Green A. Management of thyroid dysfunction during pregnancy and postpartum: an Endocrine Society Clinical Practice Guideline. J Clin Endocrinol Metab. 2007 Aug;92(8 Suppl):S1-47
- Anselmo J, Cao D, Karrison T, Weiss RE, Refetoff S. Fetal loss associated with excess thyroid hormone exposure.JAMA. 2004 Aug 11;292(6):691-5.
- Poppe K, Glinoer D, Van Steirteghem A, Tournaye H, Devroey P, Schiettecatte J, Velkeniers B. Thyroid dysfunction and autoimmunity in infertile women. Thyroid. 2002 Nov;12(11):997-1001.
- Negro R, Formoso G, Mangieri T, Pezzarossa A, Dazzi D, Hassan H. Levothyroxine treatment in euthyroid pregnant women with autoimmune thyroid disease: effects on obstetrical complications. J Clin Endocrinol Metab. 2006 Jul;91(7):2587-91.
- Polak M, Le Gac I, Vuillard E, Guibourdenche J, Leger J, Toubert ME, Madec AM, Oury JF, Czernichow P, Luton D. Fetal and neonatal thyroid function in relation to maternal Graves' disease. Best Pract Res Clin Endocrinol Metab. 2004 Jun;18(2):289-302
- Becker KL, Winnacker JL, Matthews MJ, Higgins GA: Gynecomastia and hyperthyroidism. An endocrine and histological investigation. J Clin Endocrinol Metab 28:277, 1968.
- Chopra IJ, Tulchinsky D: Status of estrogen-androgen balance in hyper- thyroid men with Graves' disease. J Clin Endocrinol Metab 38:269, 1974.
- Chopra IJ, Abraham GE, Chopra U, Solomon DH, O'Dell WD: Alterations in circulating estradiol-17-beta in male patients with Graves' disease. N Engl J Med 286:124, 1972.
- Bercovici JP, Mauvais-Jarvis P: Hyperthyroidism and gynecomastia: Metabolic studies. J Clin Endocrinol Metab 35:671, 1972.
- Southren AL, Olivo J, Gordon GG, Vittek J, Brener J, Rafii F: The conversion of androgens to estrogens in hyperthyroidism. J Clin Endocrinol Metab 38:207, 1974.
- Kidd SG, Glass AR, Vigersky RA: The hypothalamic-pituitary- testicular axis in thyrotoxicosis. J Clin Endocrinol 48:798, 1979.
- Krassas, GE; Perros, P. Thyroid disease and male reproductive function. J Endocrinol Invest 26 372-380 2003
- Abalovich M, Levalle O, Hermes R, Scaglia H, Aranda C, Zylbersztein C, et al. 1999 Hypothalamic-pituitary-testicular axis and seminal parameters in hyperthyroid males. Thyroid 9:857262.2. In thyrotoxicosis, mean sperm density is lower, and fewer sperm have normal morphology. Motility is lower in thyrotoxic males. The abnormalities normalize when the patients become euthyroid.
- Sawers JSA, Kellett HA, Brown NS, Seth J, Toft AD: Prolactin response to metoclopramide in hyperthyroidism. J Clin Endocrinol Metab 55:175, 1982.
- Kapcala LP: Galactorrhea and thyrotoxicosis. Arch Intern Med 144:2349-2350, 1984.
- Peterson RE: The influence of the thyroid on adrenal cortical function. J Clin Invest 37:736, 1958.
- Kenny FM, Iturzaeta N, Preeyasombat C, Taylor FH, Migeon CJ: Cortisol production rate. VII. Hypothyroidism and hyperthyroidism in infants and children. J Clin Endocrinol 27:1616, 1967.
- Hellman L, Bradlow HL, Zumoff B, Gallagher TF: Influence of thyroid hormone on hydrocortisone production and metabolism. 21:1231, 1961.
- Gallagher TF, Hellman L, Finkelstein J, Yoshida K, Weitzman ED, Roffwarg HD, Fukushima D: Hyperthyroidism and cortisol secretion in man. J Clin Endocrinol Metab 34:919, 1972.
- Hilton JG, Black WC, Athos W, McHugh B, Westermann CD: Increased ACTH-like activity in plasma of patients with thyrotoxicosis. J Clin Endocrinol Metab 22:900, 1962.
- Felber JP, Reddy WJ, Selenkow HA, Thorn GW: Adrenocortical response to the 48-hour ACTH test in myxedema and hyperthyroidism. J Clin Endocrinol Metab 19:895, 1959.
- Hellman L, Bradlow HL, Zumoff B, Fukushima DK, Gallagher TF: Thyroid-androgen interrelations and the hypocholesteremic effect of androsterone. J Clin Endocrinol Metab 19:936, 1959.
- Plummer HS, Boothby WM: The cost of work in exophthalmic goiter. Am J Physiol 53:406, 1923.
- Briard SP, McClintock JT, Baldridge CW: Cost of work in patients with hypermetabolism due to leukemia and to exophthalmic goiter. Arch Intern Med 56:30, 1935.
- Acheson K, Jequier E, Burger A, Danforth Jr E: Thyroid hormones and thermogenesis: The metabolic cost of food and exercise. Metabolism 33:262-265, 1984.
- Kabadi UM, Eisenstein AB: Impaired pancreatic alpha-cell response in hyperthyroidism. J Clin Endocrinol Metab 51:478, 1980.
- Wennlund A, Arner P, Ostman J: Changes in the effects of insulin on human adipose tissue metabolism in hyperthyroidism. J Clin Endocrinol Metab 53:631, 1981.
- Houssay BA: Thyroid and metathyroid diabetes. Endocrinology 35:158,1944.
- Bratusch-Marrain PR, Komjati M, Waldhausal WK: Glucose metabolism in noninsulin-dependent diabetic patients with experimental hyperthyroidism. J Clin Endocrinol Metab 60:1063, 1985.
- Elrick H, Hlad CJ Jr, Arai Y: Influence of thyroid metabolism on carbohydrate metabolism and a new method for assessing response to insulin. J Clin Endocrinol Metab 21:387, 1961.
- Gerstein HC. Incidence of postpartum thyroid dysfunction in patients with type I diabetes mellitus. Annals Int Med 118:419-423, 1993.
- Kritchevsky D: Influence of thyroid hormones and related compounds on cholesterol biosynthesis and degradation: A review. Metabolism 9:984, 1960.
- Siperstein MD, Murray AW: Cholesterol metabolism in man. J Clin Invest 34:1149, 1955.
- O’Brien T, Katz K, Hodge D, Nguyen TT, Kottke BA, Hay ID. The effect of the treatment of hypothyroidism and hyperthyroidism on plasma lipids and apolipoproteins AI, AII, and E. Clin Endocrinol 46:17-20, 1997.
- Chait A, Bierman EL, Albers JJ: Regulatory role of triiodothyronine in the degradation of low density lipoprotein by cultured human skin fibroblasts. J Clin Endocrinol Metab 48:887, 1979.
- Tulloch BR, Lewis B, Fraser RT: Triglyceride metabolism in thyroid disease. Lancet 1:391, 1973.
- Cachefo A, Boucher P, Vidon C, Dusserre E, Diraison F, Beylot M. Hepatic lipogenesis and cholesterol synthesis in hyperthyroid patients. J Clin Endocrinol Metab 86:5353-5357, 2001.
- Arons DL, Schreibman PH, Downs P, Braverman LE, Arky RA: Decreased postheparin lipases in Graves' disease. N Engl J Med 286:233, 1972.
- Sachs BA, Danielson E, Isaacs MC, Weston RE: Effect of triiodothyronine on the serum lipids and lipoproteins of euthyroid and hyperthyroid subjects. J Clin Endocrinol Metab 18:506, 1958.
- Kung AW, Pang RW, Lauder I, Lam KS, Janus ED. Changes in serum lipoprotein(a) and lipids during treatment of hyperthyroidism. Clin Chem 41:226-231, 1995.
- Ozata M, Uckaya G, Bolu E, Corapcioglu D, Bingol N, Ozdemir IC. Plasma leptin concentrations in patients with Graves’ disease with or without ophthalmopathy. Medical Science Monitor 7:696-700, 2001.
- Strisower B, Elmlinger P, Gofman JW, deLalla O: Effect of L-thyroxine on serum lipoprotein and cholesterol concentrations. J Clin Endocrinol Metab 19:117, 1959.
- Postel S: Total free tocopherols in the serum of patients with thyroid disease. J Clin Invest 35:1345, 1956.
- Rich C, Bierman EL, Schwartz I: Plasma nonesterified fatty acids in hyperthyroid states. J Clin Invest 38:275, 1959.
- Lewallen CG, Rall JE, Berman M: Studies of iodoalbumin metabolism. II. The effects of thyroid hormone. J Clin Invest 38:88, 1959.
- Crispell KR, Parson W, Hollifield G: A study of the rate of protein synthesis before and during the administration of L-triiodothyronine to patients with myxedema and healthy volunteers using N-15 glycine. J Clin Invest 35:164, 1956.
- Sokoloff L, Kaufman S: Effects of thyroxine on amino acid incorporation into protein. Science 129:569, 1959.
- Sokoloff L, Kaufman S, Gelboin HV: Thyroxine stimulation of soluble ribonucleic acid bound amino acid transfer to microsomal protein. Biochim Biophys Acta 52:410, 1961.
- Kivirikko KI, Laitinen O, Aer J, Halme J: Metabolism of collagen in experimental hyperthyroidism and hypothyroidism in the rat. Endocrinology 80:1051, 1967.
- Singh SP, Snyder AK: Effect of thyrotoxicosis on gluconeogenesis from alanine in the perfused rat liver. Endocrinology 102:182, 1978.
- Benvenga S, Ruggeri RM, Russo A, Lapa D, Campenni A, Trimarchi F. Usefulness of L-carnitine, a naturally occurring peripheral antagonish of thyroid hormone action, in iatrogenic hyperthyroidism: a randomized, double-blind, placebo-controlled clinical trial. J Clin Endocrinol Metab 86:3579-3594, 2001.
- Guttler RB, Croxson MS, DeQuattro VL, Warren DW, Otis CL, Nicoloff JT: Effects of thyroid hormone on plasma adenosine 3',5'-monophosphate production in man. Metabolism 26:1155, 1977.
- Nilsson OR, Andersson RGG, Karlberg BE: Effects of propranolol and atenolol on plasma and urinary cyclic adenosine 3',5'-monophosphate in hyperthyroid patients. Acta Endocrinol 94:38, 1980.
- Peracchi M, Bamonti-Catena F, Lombardi L, Reschini E, Toschi V, Maiolo AT, Polli EE: Plasma and urine cyclic nucleotide levels in patients with hyperthyroidism and hypothyroidism. J Endocrinol Invest 6:173, 1983.
- Wohl MG, Levy HA, Szutka A, Maldia G: Pyridoxine deficiency in hyperthyroidism. Proc Soc Exp Biol Med 105:523, 1960.
- Stein JA, Griem ML: Effect of triiodothyronine on radiosensitivity. Nature 182:1681, 1958.
- Parker JLW, Lawson DH: Death from thyrotoxicosis. Lancet 2:894, 1973.
- Davis PJ, Davis FB: Hyperthyroidism in patients over the age of 60 years. Medicine 53:161, 1974.
- Wong GWK, Lai J, Cheng PS. Growth in childhood thyrotoxicosis. Eur J Pediatr 158:776-779, 1999.
- Segni M, Leonardi E, Mazzoncini B, Pucarelli I, Pasquino AM. Special features of Graves' disease in early childhood. Thyroid 9:871, 1999.