ABSTRACT
In people with diabetes the comorbidity with depression is associated with micro- and macrovascular complications and increased mortality. Health-related quality of life is often reduced, and the adherence to treatment is generally low. Screening and diagnostic tools for depression are widely available. However, their use is only effective if subsequent treatment pathways are provided, which is often not the case. Meta-analyses on the treatment of depression in diabetes indicate that depression can be effectively treated with a variety of psychological and/or psychopharmacological interventions. However, results from the most frequently studied psychological treatment, cognitive behavioral therapy (CBT), have revealed decreasing effects ranging from large to low-grade, the longer the studies’ follow-up lasts. This may indicate CBT’s reduced long-term efficacy in diabetes patients with depression compared to depressive people without diabetes. As few data are available on the long-term effects of drug treatments, the studies’ conclusiveness is limited. Regarding the amelioration of glycemic control, the treatment results are heterogeneous, indicating a slight improvement by selective serotonin reuptake inhibitors and contradictory evidence for psychological interventions. This chapter concludes with a practice-oriented model of stepped care for depression treatment in people with diabetes. For complete coverage of this and all related areas of Endocrinology, please visit our FREE on-line web-textbook, www.endotext.org.
INTRODUCTION
Diabetes mellitus can be considered a paradigm of a 21st century chronic disease where prognosis and progression are significantly dependent on the patients’ lifestyle and self- management behaviors. Treatment requires lifelong planning and controlling both one’s food intake and making multiple changes to other lifestyles such as physical activity. The patient bears a high degree of personal responsibility, and many patients have trouble implementing treatment recommendations successfully. Comorbid mental disorders, which often hinder the smooth integration of diabetes into everyday life, constitute a major barrier to successful diabetes self-management.
As one of the most common mental disorders, depression occurs frequently in patients with diabetes and has a bi-directional relationship: diabetes raises the risk of depression, and depressed individuals also have an increased risk of developing type 2 diabetes. Since the co-morbidity of diabetes and depression has been understood for many years as a "hen-egg problem", recent findings imply parallel development of biological mechanisms thought to be responsible for both the development of depressive disorders and type 2 diabetes. Among other factors, it is assumed that the hypothalamic pituitary adrenal axis is dysregulated and that the immune system is overactivated over the life span, factors promoting insulin resistance, endothelial dysfunction, and cardiovascular disease, all of which raise the risk of depression, type 2 diabetes, and premature mortality. A direct depression-promoting effect of inflammatory processes on the brain is also assumed. Apart from these biological factors, numerous findings indicate that the psychosocial stress caused by diabetes and its complications also contribute to increased depression rates.
The comorbidity of depression and diabetes impacts medical outcome of people suffering from both conditions. More specifically, symptoms of depression are associated with hyperglycemia, micro- and macrovascular complications, and significantly increased mortality in people with diabetes. Patients often suffer from lower general and diabetes-specific quality of life, making how they manage their diabetes and adhere to therapy recommendations much more difficult.
The detection rate for depression in patients with diabetes is low and this inevitably goes hand-in-hand with inadequate therapy. Several screening and diagnostic tools have been developed that enable the rapid and reliable diagnosis of depression and are not costly to implement. But, if depression screening and subsequent diagnosis fail to lead to adequate care through an established treatment pathway, the potential adverse effects of not providing an evidence based intervention may outweigh the benefit of screening for depression.
The treatment of comorbid depression in diabetes differs mainly in its objectives from those of depression not accompanied by chronic physical illness. In view of the complex psychological and somatic interactions, depression treatment targets not only mental symptoms and problems, it also includes diabetes-related medical goals that focus particularly on improving the patient’s adherence to treatment and glycemic control to help prevent diabetes-related complications. The models of care for depression in diabetes include various approaches ranging from psychopharmacological treatment to psychological interventions. These are complemented by algorithm-based interventions delivered by different professional groups (e.g., collaborative care or stepped-care approaches). These are now being supplemented by telemedical or web-based interventions. Current scientific evidence demonstrated good treatment results regarding depression outcome in diabetes patients with comorbid depression. This applies to a variety of psychopharmacological or psychological interventions or a combination of both. Results are much less consistent regarding the physical targets of diabetes therapy, as contradictory findings reveal that we still do not know which is the most effective therapeutic approach in this field.
Based on existing guidelines and updated by current research, we suggest a practice oriented model of stepped care for patients with diabetes and depression that could be adapted to the individual patient’s care requirements.
This ENDOTEXT chapter aims to summarize the current state of research on the co-morbidity of diabetes and depression in adult patients with type 1 and type 2 diabetes.
Since a great deal of published research does not differentiate between the different types of diabetes, both types are addressed in this chapter as well. However, where differentiated information is possible, it is specified in the text. The concept that diabetes distress overlaps with depression but should be distinguished from depression as a mental disorder (1, 2) is not the subject of this chapter.
DEPRESSION
As depression is one of the mental disorders often overlooked by non-psychiatric physicians, it is frequently treated inadequately (3). This is also the case for patients with diabetes and comorbid depression; their diagnostic rate for depression ranges from 45% (4) to 50% (5). The dangerous interactions with diabetes and the frequent disregard of depression highlight the importance of informing healthcare professionals about the diagnostic criteria of depressive disorders.
According to the ICD-10 classification of the World Health Organization, depressive symptoms can be assigned to different categories of mental disorders (6). One essential distinguishing feature concerns the course of the depression. For the most part, a distinction is made between depressive episodes of varying degrees of severity that occur for the first time, and recurrent or persistent disorders. In addition, depressive symptoms are treated in the context of adaptation disorders, other diseases and various diagnostic "residual categories". The present chapter deals only with unipolar depression and not with bipolar affective disorders, cyclothymia, or manic episodes.
The main focus of the ICD-10 classification is on the depressive episode, which distinguishes at least two weeks of major symptoms from additional symptoms.
Main symptoms
- depressed mood,
- loss of interest and enjoyment
- reduced energy leading to increased fatigability and diminished activity
Other common symptoms
- reduced concentration and attention
- reduced self-esteem and self-confidence
- feeling guilty and worthless
- bleak and pessimistic views of the future;
- ideas or acts of self-harm or suicide;
- disturbed sleep;
- diminished appetite.
The severity of the depressive episode is specified by counting the number of current depression symptoms:
- mild (4-5 symptoms): 2 main and to 2-3 additional symptoms
- moderate (6-7 symptoms): 2 main and 3-5 additional symptoms
- severe (at least 8 symptoms): 3 main and at least 4 additional symptoms
In addition, a mild or moderate depressive episode can be described as coinciding with "somatic syndrome" or not. This applies if at least four characteristics of the somatic syndrome are fulfilled (in case of severe depressive episodes, the somatic syndrome is generally assumed due to the variety of symptoms).
Characteristics of Somatic Syndrome
- loss of interest or pleasure in normally enjoyable activities
- inability to react emotionally to a friendly environment or joyful events
- early morning awakening (two or more hours before the usual time)
- morning low
- psychomotor inhibition or agitation
- significant loss of appetite
- weight loss, often more than 5% of body weight in the past month;
- significant loss of libido
Furthermore, regarding a severe depressive episode: a distinction between patients "with" and "without" psychotic symptoms can be made. If the patient presents any psychotic symptoms (e g, impoverishment or delusional delusions), hallucinations, or depressive stupor, the patient is additionally referred to as "with psychotic symptoms".
The most important diagnostic categories of ICD 10 in which symptoms of unipolar depression occur are the
- Depressive episode during which the above-mentioned symptoms occur for the first time for at least two weeks;
- Recurrent depressive disorder diagnosed if a depressive episode has already occurred in the patient’s past;
- Dysthymia, a persistent affective disorder in which symptoms of depression persist for at least two years but do not or rarely fulfill the criteria of a depressive episode; and
- Adjustment disorders in which depressive symptoms may occur as part of a disturbed adaptation process following a stressful life event, but at no time are all the criteria of a complete depressive episode fulfilled. A distinction is made between the short depressive reaction (duration shorter than one month), the longer depressive reaction (lasting up to two years) or the adjustment disorder with mixed anxiety and depressed mood.
For a comprehensive description of the criteria for each category of specific disorders, see ICD-10 Clinical descriptions and diagnostic guidelines (6). More detailed criteria are defined in the ICD 10 Diagnostic criteria for research (7).
EPIDEMIOLOGY OF DEPRESSION IN DIABETES
Prevalence and Incidence of Depression in Patients with Diabetes
People with diabetes (type 1 and type 2) are affected by depressive symptoms relatively often. They reveal approximately twice the prevalence of depressive disorders as control groups without diabetes. According to the most often-cited meta-analysis of controlled studies published already in 2001, about 9% of patients with diabetes suffer from a depressive disorder (vs. 5% in the control groups) and about 25% of them suffer from clinically significant depression symptoms, some of which do not meet the criteria of a depressive disorder (vs. 14% in the control group) (8).
A recent meta-analysis using data from longitudinal studies included 11 studies reporting binary estimates (RR) and five studies reporting time-to-event estimates [hazard ratio (HR)]. Both RR and HR were significant at 1.27 (95 % CI 1.17–1.38) and 1.23 (95 % CI 1.08–1.40) for incident depression associated with diabetes mellitus and confirmed that diabetes is a significant risk factor for the subsequent onset of depression (9).
As a limitation to the generalizability of these results, it should be noted that rates of depression differ greatly across studies depending on their methodology and how they were conceived, factors that contribute to the inconsistency of their findings. For example, depressive symptoms and depressive disorders are often not clearly distinguished in scientific publications. Confusion is also caused by the fact that the concepts of diabetes distress and depression symptoms only partially overlap and are often insufficiently differentiated (2, 10, 11).
It should also be noted that sample selection effects and confounding sociodemographic variables also contribute to inconsistent results. For example, symptoms of depression were detected more frequently in type 1 than type 2 diabetes (12), in women compared to men (12, 13), in clinical samples compared to population-based samples, in patients with more than two diabetes complications (13) and also more frequently in questionnaire studies than in the more valid (semi-)standardized diagnostic interviews (8, 14, 15). Other differences arise from comparing patient cohorts of different ethnicities and from diverse regions (summarized in: (16)).
Recent results of a meta-analysis comparing patients with prediabetes, undiagnosed diabetes, and diagnosed diabetes revealed higher depression rates especially in individuals with diagnosed diabetes. This is likely due to the psychological burden of knowing you have a life-long chronic illness like diabetes and of being confronted with the burden of diabetes treatment – a few of the factors contributing to the occurrence of depressive symptoms (17).
Prevalence and Incidence of Diabetes in Patients with Depression
The interactions between depression and diabetes are bi-directional: not only does the risk of developing depression in addition to having diabetes increase, but depressed individuals also have a higher risk of developing type 2 diabetes during the course of their affective disorder. Previous meta-analyses of the relationship between depression and subsequent type 2 diabetes have revealed risk increases of 25% (18), 37% (19), 38% (20), and 60% (21). The most recent meta-analysis, published in 2015, included 33 studies covering a total of more than 2.4 million participants. Its results indicate that people with depression have a 41% higher risk of developing diabetes mellitus (with no differentiation between the types of diabetes) (22). Studies of this meta-analysis addressing only type 2 diabetes reported a 32% increase in the risk of developing diabetes in people with depression. Interestingly, this meta-analysis also examined regional differences, showing that studies from the USA reported a 28% risk increase, European studies 31%. By contrast, studies conducted in Asia showed a 149% increase in risk, however, the differences are not explained (22).
Interactions Between Diabetes and Comorbid Depression
Interactions between depressive disorders and diabetes are life-threatening and affect almost all outcome parameters of diabetes. There is ample evidence that depressive co-morbidity is associated with a markedly worse diabetes course. In patients with diabetes, depressive disorders are associated with hyperglycemia (23-25) and micro- and macrovascular complications (26). They also present markedly increased mortality (27-30), especially in elderly patients with type 2 diabetes (28) and in those with type 2 diabetes after myocardial infarction (31).
In terms of gender-specific differences: the results are inconclusive regarding the mortality of people with diabetes and depression. Findings from the Nurses' Health Study, a large prospective cohort study including 78.282 women in the US indicated an 3.1-fold increased relative risk (RRs) of mortality for women with diabetes and depression compared with participants without either condition; the authors interpreted this to mean that women have the higher risk of mortality (32). However, a large population-based longitudinal study in Norway recently revealed an excess mortality risk of 3,4 (Hazard ratio) associated with depression and anxiety only in men with diabetes, but not in women (33).
Negative interactions between diabetes and depression are also evident with regards to psychological outcomes. For example, the health-related quality of life of patients with diabetes and depression is considerably reduced (34) and the stress caused by diabetes is perceived as more pronounced than in non-depressed diabetes patients (35).
There is also solid evidence that depressed individuals with diabetes find it much harder to adhere to treatment recommendations. It has been observed that as depression worsens, diabetes medicines are taken less regularly and patients’ satisfaction with diabetes therapy decreases. Depressed patients with diabetes also eat unhealthier diets (36), are physically less active, are often overweight or obese, and are more likely to smoke than non-depressed people with diabetes (37-39).
DEPRESSION DIAGNOSIS AND SCREENING IN DIABETES
At most, half of depressed patients with diabetes in primary care are acknowledged to be depressed and insufficiently treated (40). Such inadequate diagnostic rates of depressive disorders are not specific to people with diabetes - they are a major problem in medical care (41, 42).
However, if patients with diabetes suffer from comorbid depression, diagnosing a depressive disorder can be made even harder because of the two conditions’ overlapping symptoms. Non-depressed individuals with diabetes, especially those with poor glycemic control, may suffer from fatigue, appetite change, or libido reduction (43) - typical symptoms of depression. Yet other symptoms of depression do not overlap with the physical symptoms of diabetes, and their detection by clinicians can facilitate the correct diagnosis of depression. This applies to the cognitive and affective symptoms of depression (e.g., depressive mood, anxiety, diminished self-esteem, feelings of guilt, pessimism, anhedonia, suicidal thoughts) (44). Another difficulty in diagnosing depression in patients with diabetes is that those affected are often unaware they are suffering from depression. They are often more apt to complain about diffuse physical maladies during medical consultation, while psychological symptoms are frequently concealed or trivialized (45).
Clinical interviews such as the Structured Clinical Interview for DSM-IV-TR Axis I Disorders SCID interview (46) SKID or the Schedule for Clinical Assessment in Neuropsychiatry 2.1 (47), are considered the gold standard for diagnosing mental disorders like depression. Being time-consuming and requiring appropriate training, they are mainly used in research. In some cases, questionnaires alone are used to screen and diagnose depression concurrently. However, as this method is associated with exaggerated rates of depression (48, 49), it is not an advisable diagnostic option.
Given the time constraints of medical consultation in primary care, offering screening questionnaires as the first step can be a time-saving alternative. Only a positive screening result would then be followed by a clinical diagnostic procedure to confirm the diagnosis and consider differential diagnoses. For the subsequent diagnosis of a depressive episode after positive screening, it can be useful to go through the individual items on the Patient Health Questionnaire PHQ-9 (50) together with the patient in conversation to check whether the criteria for a depressive disorder are fulfilled. To diagnose more complex forms of depression, it is advisable to refer the patient to a specialist such as a psychiatrist or clinical psychologist.
A confirmed diagnosis of depression must always be followed by a clear treatment pathway and continuous monitoring of therapy results (51).
Overview and Evaluation of Screening Tools
There are several screening and diagnostic tools that facilitate rapid and reliable diagnosis of depression and are not costly. In their review from 2012, Roy and colleagues (49) identified the following questionnaires as being those most frequently used to detect depression in patients with diabetes: the Beck Depression Inventory (BDI (52); BDI-II (53)), Center for Epidemiologic Studies Depression Scale (CES-D) (54), the Hospital Anxiety and Depression Scale (HADS) (55), and different versions of the Patient Health Questionnaire (PHQ)(56). The WHO-5 Well-Being Index (57) has also been evaluated as a first-step screening instrument for depression in adults with diabetes (58).
Many studies to identify the most effective questionnaires for screening depression in people with diabetes have led to contradictory results, some of which are attributable to different populations and settings (35, 48, 59, 60). In conclusion, the BDI, CES-D, PHQ, WHO-5 and HADS exhibit adequate clinical specificity and sensitivity when screening for depression in individuals with diabetes (58, 61). Altogether, the PHQ-9 seems to have the highest validity as the first step in a two-stage screening procedure in people with diabetes (62); it yielded the best evidence for adequate sensitivity (63). A recent population-based cohort study including patients with type 2 diabetes recommended using a PHQ-9 cutoff score of 5, as that demonstrated the best sensitivity (92.3%), with acceptable specificity (70.4%), in a two-stage screening setup in primary care to select individuals who need to undergo further psychological evaluation to confirm or reject the depression diagnosis (63).
Effectiveness of Depression Screening in Diabetes Care
There is a controversy about the recommendations to screen for illnesses, as long as no advantage of screening has been demonstrated and negative consequences cannot be ruled out. An example of a screening disadvantage is that the stigma of a depression diagnosis can lead to the exclusion of health insurance coverage (64).
Results of a Cochrane meta-analysis showed that depression screening in the general population has little or no effect on detection rates or the treatment of depression by practitioners when a positive screening result is not associated with specific treatment options. Thus recommendations for screening for depression without subsequent established treatment pathways are not considered worthwhile (65). Similar conclusions have also been drawn by various reviews addressing the screening for depression in people with diabetes (49, 51, 61, 66).
Two RCTs demonstrated the lack of a benefit from depression screening in individuals with diabetes in the absence of subsequent treatment pathways for patients identified as positive for depression. Both studies’ authors found that compared to standard therapy, there was no subsequent alleviation of depressive symptoms thanks to a positive screening result, even when treatment costs rose slightly (67, 68).
Various reasons have been identified for the disappointing efficacy of depression screening in people with diabetes (51): First, the quality of treatment for depression in primary care tends to be suboptimal (69) – an assumption we can also make for patients with diabetes (61). Another factor is that individuals with diabetes tend to be reluctant to participate in depression screening and to follow a subsequent treatment recommendation (70-72). There is also evidence that patients (70, 71) or those in poor health (72) are often underrepresented in screening procedures. Finally, an obstacle to effective depression screening also lies in the fact that some medical staff may perceive screening as being incompatible with a patient-centered approach (73).
To date, there is insufficient scientific evidence for the cost-effectiveness of depression screening in diabetes patients. Furthermore, as the quality criteria (74) for screening are unfulfilled in many countries, screening recommendations must always be considered against the background of the different health care systems in many countries (75). In contrast to the limitations described above, there is clear scientific evidence that depression screening has a positive effect on the outcome of treatment provided it is followed by subsequent confirmation of the diagnosis and adequate treatment (76, 77).
To summarize the latest scientific evidence, we can conclude that depression screening for patients with diabetes is only effective when it is embedded in a comprehensive healthcare system that ensures subsequent diagnosis and treatment. Where these possibilities exist, screening should be offered on a regular basis. Otherwise, depression screening is neither effective nor ethically justifiable (51).
Practical Recommendation for Screening
Different questionnaires like the Problem Areas in Diabetes (PAID) questionnaire (78) or the WHO-5-Well-Being-Index, which possess good specificity and sensitivity, have been used for depression screening. However, their items are formulated in a manner quite distinct from the diagnostic criteria of a depressive disorder. Screening with the Patient Health Questionnaire PHQ-9 (79) is therefore preferable as its items are almost identical to those of depression criteria – a factor that could help structure the subsequent depression diagnosis in a face-to-face consultation in case of a positive screening result (text box 1).
Text box 1: Example of an effective two-step screening and diagnostic procedure for depression followed by treatment recommendation in primary care The two questions of the Patient Health Questionnaire PHQ-2 (50) (sensitivity 95%, specificity 57%(80)) can either be presented as a questionnaire in preparation for the consultation or it can be included in the interview. They are identical to the first two items on the PHQ-9. If they are presented during the face-to-face consultation it is advisable to briefly explain the procedure to the patient beforehand. For example: “I’d like to go through a list of symptoms that sometimes occur when you feel unwell. It is simply a question of whether you have suffered from the symptoms on this list over the past two weeks on most days and most of the day.” After the patient has given his or her consent, the questions of the PHQ-9 are read aloud.
Start with the two questions ‘Over the past two weeks have you been bothered by 1. a) little interest or pleasure in doing things 1. b) feeling down, depressed or hopeless’.
If one of the questions is answered with “yes” ask the remaining seven questions on the PHQ-9 (representing the depression criteria). If not, the procedure can be terminated here. Since this is a third-party assessment, the decision as to whether a criterion is fulfilled is up to the physician, not the patient. If this procedure is carried out consistently, the diagnostic assessment takes less than 5 minutes. If you are not qualified to diagnose depression, you should refer the patient to a healthcare professional after a positive screening result. With trained healthcare professionals, the depression diagnosis is confirmed or rejected based on the patient’s answers. If there are doubts about the validity of the depression diagnosis, consultations should be carried out by a specialist (psychiatrist or clinical psychologist). If the diagnosis is confirmed, it is crucial to inform the patient about treatment options and specific pathways where treatment can be obtained. |
THE LINK BETWEEN DEPRESSION AND DIABETES IN TYPE 1 DIABETES
Overview
Patients with type 1 diabetes have a complex management regimen to follow to maintain optimal health. This includes regular and frequent monitoring and keeping a record of blood glucose concentrations, knowing what varying glucose values signify, and being able to calculate and administer insulin doses in line with their carbohydrate intake and physical activity. These self-care activities have a psychological impact, and it is widely acknowledged that many patients have difficulty mastering them while striving towards optimal glycemic control. Understanding the mechanism between type 1 diabetes and depression will be examined along the lifespan from childhood and adolescence to adulthood.
Childhood
Around half of all cases of type 1 diabetes have their onset in childhood and thus the potential to cause substantial disruption to normal developmental processes which in turn increase the risk of depression.
Depression can be difficult but not impossible to diagnose in childhood, yet few studies have investigated the association between depression and type 1 diabetes during that phase of life. It is likely that the child experiences distress that probably goes undetected but which might manifest as irritability, oppositional behavior, refusing to go to school, or abnormal illness behaviors. The adult caregiver is usually responsible for the complex decision-making associated with these tasks, such as dosing insulin based on blood glucose readings and diet, and these issues should be considered in conjunction with the burden of parental responsibility (81).
Very few studies have addressed of the effect of the family milieu, and those that exist suggest that children with type 1 diabetes who grow up in an high expressed emotion environment are more likely to have poor glycemic control (82, 83). The quality, nature and strength of attachment between child and parents is a key factor in how well the child adjusts to type 1 diabetes and in its psychological sequelae. The attachment theory proposes that past experiences with caregivers are incorporated psychologically to form cognitive models that inform future interpersonal relationships, and these patterns of attachment persist in later life and may replicate in the individual's relationship to their diabetes (84, 85).
Adolescence
The transition from childhood to adolescence is when caregiving is transferred from the patient’s parents to self-managing their diabetes. Greater independence coincides with emergence of risk-taking behaviors, such as experimenting with alcohol and other substances, the development of one’s identity, and desire for peer approval (86, 87). Biologically speaking, puberty is associated with physiological insulin resistance in part to increase the production of growth hormone. In view of these rapid psychological and physiological changes, diabetes distress is widely acknowledged in adolescents with type 1 diabetes, and is associated with poor glycemic control, prominent negative beliefs about diabetes, such as the fear of hypoglycemia when with others, and reduced self-efficacy (88). These experiences of transition from childhood dependence towards adulthood and independence can be linked to emotions expressed within the family that are associated with poor glycemic control (89).
Adolescence is a critical window for the emergence of eating disorders, as becoming more aware of one’s body and the body image are part of the process of becoming aware of the self, and their interaction with type 1 diabetes is additive at this stage of life. Depression is often a comorbidity of eating disorders (90). In a case-control study comparing patients with both type 1 diabetes and an eating disorder to those with only an eating disorder, the group with type 1 diabetes had statistically significantly lower scores on the Beck Depression Inventory—an unexpected outcome (91).
Early Adulthood
Despite the plethora of studies reporting the prevalence of depression in type 1 diabetes in adulthood, mechanistic studies of depression are sparse. Those who are most depressed have the poorest glycemic control (92). However, cross-sectional evidence suggests that diabetes-specific emotional distress, rather than depression, is associated with poor glycemic control in adults with type 1 diabetes (93). Another mechanism is social problems that are very often neglected in the conventional clinical model; patients with depression are more likely to be of non-Caucasian ethnicity and have lower socioeconomic status (94).
The link between depression and diabetes in type 2 diabetes
Common Biological Origins of Depression and Type 2 Diabetes
The association between depression and type 2 diabetes is bidirectional and the underlying mechanisms are complex. Mediation by behavioral factors such as reduced self-care do not fully explain this link. For instance, depressive symptoms are not as strongly associated with worse glycemic control as would expect (95). Depressive symptoms appear to have a stronger effect on the risk of type 2 diabetes than the effect of type 2 diabetes on the risk for depression (60% versus 24%, respectively) (21, 96).
In terms of the natural history of type 2 diabetes, pooled data suggest there is also a link between depression and insulin resistance (97). A few prospective studies showed that depression is associated with later insulin resistant partly attenuated by obesity (98). These findings suggest there may be preceding risk factors and mechanisms shared by both conditions. Potential common mechanisms include the chronic activation of innate immunity, the HPA axis, and circadian rhythms.
Innate Immunity and Inflammation
Activation of the innate immunity and an acute-phase inflammatory response underlie the pathogenesis of type 2 diabetes, factors substantiated by systematic reviews of observational studies (99). There is also evidence that the mechanism of some experimental diabetes treatments may be anti-inflammatory, such as interleukin 1 receptor antagonist and non-steroidal anti-inflammatory drugs (100, 101). The cytokine-mediated inflammatory response is also implicated in depression. Increased cytokine serum concentrations appear to communicate across the blood-brain barrier (102). Depressive symptoms are a common side effect of inflammatory agents such as the cytokine interferon alfa (103). A meta-analysis of the association between cytokines and major depression found that depressed individuals presented statistically significantly higher circulating concentrations of tumor necrosis factor (TNF) and interleukin-6 (104). Furthermore, adjunctive therapy with the anti-inflammatory celecoxib (PTGS2 inhibitor) reduced depressive symptoms (105).
Whether the role of innate immunity in depression in people with type 2 diabetes is the same as those with depression but without type 2 diabetes is not known, but on face value it is likely to be the same mechanism. In a population-based sample of people with incident-type 2 diabetes, those with significant depressive symptoms already had a poor prognosis at diagnosis. They were more overweight, approximately 5 years younger, had higher concentrations of C-reactive protein (CRP) and interleukin 1-receptor antagonist, and higher white-cell counts, but not worse glycemic control than people with type 2 diabetes who were not depressed (106). Increased concentrations of CRP and interleukin 6 are known to raise the risk of type 2 diabetes (107) and depression (108) after adjusting for covariates. In a large Japanese cohort of over 3500 outpatients with type 2 diabetes, the cross-sectional association between high CRP concentrations and depression was significant in those with high BMI (109). Depression is associated with a significant increased risk of dementia in patients with type 2 diabetes compared to those without depression and without type 2 diabetes, (110) and increased concentrations of interleukin 6 are associated with cognitive decline in patients with type 2 diabetes (111).
The association between the activation of innate immunity and depression and type 2 diabetes has been observed early in the life course (112). Childhood abuse, neglect, or both are major mediators of the cross-sectional relation between increased concentrations of inflammatory cytokines and depression in adults (113).
If inflammation is involved in pathogenesis of depression in type 2 diabetes, reducing inflammation might be a novel treatment. However, no studies have attempted to modify inflammation when treating depression in patients with type 2 diabetes. With the potential benefit of improving glycemic control and alleviating depressive symptoms concurrently, anti-inflammatory approaches to treat depression in patients with type 2 diabetes should be a focus of the next generation of research.
The Hypothalamic-Pituitary-Adrenal Axis (HPA Axis)
Acute and chronic stress activates the HPA axis which regulates the adrenal glands’ glucocorticoid production. There are many definitions of psychosocial stress, and depression lies within the stress spectrum. Here stress is understood as what the individual perceives as a psychological burden based on how they interpret their past and present sensory inputs from their environment. Psychosocial stress entails many different environmental processes and factors ranging from socioeconomic deprivation and occupational status and hierarchy, life events, abuse, and trauma.
Chronic stress is associated with hypercortisolemia and can lead to increased circulating free fatty acids that can impair the function of insulin (114). These factors are thus on the causal pathway toward metabolic dysfunction, insulin resistance, and type 2 diabetes.
Depression is associated with chronic dysregulation of the HPA axis. Excess cortisol hinders neurogenesis in the hippocampus, a region implicated in both depression and type 2 diabetes (115-117) The difficulty in interpreting many of these studies is that overactivation of the HPA axis is most obvious in patients with severe depression, whereas most depressed individuals suffer from depression of mild-to-moderate severity. The evidence of HPA-axis dysregulation in this group is less convincing (118).
Nevertheless, that HPA-axis dysregulation might play a role represents a plausible common link in both depression and type 2 diabetes (119). Excess stress early in the life course leads to attenuated development of the hippocampus and amygdala, which are areas in the brain linked to both depression and type 2 diabetes (120). Pharmacological manipulation of the HPA axis could provide novel treatments for both conditions, as animal models have suggested, but such investigations have not been conducted in humans yet (121).
Circadian Rhythms
Both depression (122) and type 2 diabetes reveal disrupted circadian rhythm and sleep patterns. It is noteworthy that increased concentrations of CRP and interleukin 6 are present in a range of disturbed sleep patterns such as hypersomnolence, decreased slow-wave sleep, and increased rapid eye movement density (123-126).
At the cellular level, environmental cues such as light–dark cycles, food, and social cues, control the expression clock genes (127). In patients with type 2 diabetes, clock gene expression has been directly associated with fasting glucose concentrations (128). In patients with depression, sleep deprivation therapy might be due to the resetting of abnormal clock genes and subsequent restoration of circadian rhythms (129). This finding highlights the translational potential of these emerging biological pathways, and studies investigating the role of clock genes in depression in patients with type 2 diabetes are needed.
Psychological Factors
Depression is one of the most common psychological factors in type 2 diabetes, but there are additional independent but not mutually exclusive psychological factors, namely the diabetes distress or burden, and other psychological processes such as disordered eating.
Diabetes Distress
Generic quality of life, wellbeing and distress measures enable us to compare conditions, but they do not capture the distress and burden that relate specifically to the patient’s disease (2, 35). This is important because these specific cognitions and affect or worries are linked to avoidant disease behaviors. In the context of diabetes, this includes medication administration, the self-monitoring of biodata, and assuming a healthier lifestyle. Thus, there is validity to proposal that detecting and intervening with negative, diabetes-related cognitions and emotions may help improve confidence and the ability to self-manage. Typical type 2 diabetes cognitions are very closely related to guilt and shame worsened by societal stigma and “fat shaming”, namely cultural beliefs that the patient is to be blamed for the condition through the neglect of their health and body. Other diabetes-specific stressors are living with fluctuating blood glucose concentrations, the continuous need to calculate and balance insulin doses with carbohydrate intake and physical activity, and worries about hypoglycemia and about passing the condition on to their offspring. Patients often describe these as burdens that are '24/7'.
Delays in initiating insulin treatment are also increasingly acknowledged (130). The reasons are multifactorial, but in essence the patient-clinician relationship sometimes can sometimes discourage the use of insulin. Clinicians may avoid mentioning insulin as a treatment option, as that is akin to giving bad news, and some use insulin as a threat in the ill-founded hope that the patient will then adopt a healthier lifestyle and take their diabetes medication as prescribed. Patients, on the other hand, perceive insulin as the end-of-the-line, that disabling and frightening complications are around the corner, that they have been a 'bad diabetic' and failed; they may worry about injecting, or be afraid of needles (of both injecting insulin and other injectable therapies, and of lancing to check blood glucose) (131). In some cultures, insulin initiation may lead to marital breakdown. When these fears lead to anxious or depressive symptoms, this can lead to the phobic avoidance of insulin therapy.
The most commonly used measures of diabetes distress are the Problem Areas In Diabetes (PAID) scale (78), and its revised and shorter version, the 17-item Diabetes Distress Scale (DDS) (132), both of which quantify diabetes-distress. Up to a third of people with diabetes suffer from diabetes distress, and its severity and frequency differ across clinical settings (less in primary than in secondary care) (133), nations, and ethnicities (134).
Diabetes-distress exhibits moderate to strong positive correlations with self-reported measures of depression (135). There has been longstanding controversy as to which (depression or distress) has the strongest effects on self-care, and whether diabetes distress is a cognitive representation of depression or an independent psychological process (136). In a large longitudinal (lasting over 12 months) multinational study of adult patients with both types of diabetes, there were slightly higher prevalence rates of diabetes distress versus depressive symptoms (13% versus 10%, respectively ) with about 5% screening positive for both (137). On the other hand, in another study, worse glycemic control increased the risk for diabetes distress, but not for depressive symptoms (138). There is still a need for more observational studies using repeated measures to monitor the onset, progression, and interaction between depressive symptoms and diabetes distress and the relative effects of these on self-management. This is clinically important to develop innovations in psychological interventions, as the scientific community needs to decide which should take priority: treating depressive symptoms, or alleviating diabetes distress.
Other Psychological Factors
That eating disorders are common in type 2 diabetes was first described over 20 years (139, 140), yet there is still insufficient research in this field. Many patients describe their behavior as grazing, emotional eating, or a feature of boredom especially when they are sedentary, such as when doing office work or watching TV. There is a paucity of cross-sectional studies assessing this comorbidity and determining which of these conditions comes first. This topic would seem important to the primary prevention of type 2 diabetes, and to ensuring the most appropriate treatments are targeted. For instance, interventions to integrate depression management in type 2 diabetes might alleviate depression, but if longstanding habits, e.g., relieving negative emotions by eating, are not also addressed, they will persist.
PREVENTION OF DEPRESSION IN PEOPLE WITH DIABETES
Given the increased risk of depression in people with diabetes, it seems necessary to offer interventions to prevent depression for this high-risk group of patients. In fact, as results from a meta-analysis demonstrated, there are effective approaches to prevent depression in people without diabetes (141). However, there is presently no scientific evidence on such interventions in people with diabetes (142).
Two recent trials focused on the secondary prevention of major depression for patients with diabetes already affected by minor depression, a group with a substantially higher risk for exacerbated depression. In the first trial, a stepped-care program for subthreshold depression was compared with usual care in patients with type 2 diabetes and/or coronary heart disease. Their results indicated that this intervention was no more effective than usual care in preventing major depression (143). In contrast, results from a multicentric, randomized, controlled trial including elderly patients with type 2 diabetes and minor depression demonstrated that after 15 months, cognitive behavioral therapy (CBT) was more effective than treatment as usual to prevent the progression or conversion to major depression (144).
TREATMENT OF DEPRESSION IN DIABETES
Treatment Goals
Considering the deleterious interactions between the two disorders, depression treatment should obviously always focus simultaneously on medical and psychological goals in people with diabetes (51, 145). Depression-related goals prioritize the prevention of suicidal acts where relevant in individual cases. Apart from this, the central aim of the treatment of depression is complete remission or at least significant alleviation of depression symptoms. Further objectives include preventing subsequent depressive episodes and improving health-related quality of life. The most important medical treatment goal is to reduce diabetes-related complications and premature mortality. Glycated hemoglobin (HbA1c) is an established biomarker for the prognosis of diabetes and is thus usually considered a primary target variable. These somewhat general therapy goals are supplemented by the patients’ goals, which are usually defined more concretely and often incorporate other aspects of their life (text box 2). In summary, providing the ideal therapy of depression for patients with diabetes would be an intervention that reduces the symptoms o depression and improves glycemic control concurrently (51, 145).
There is no scientific evidence for prioritizing medical or psychological treatment goals for people suffering from depression and diabetes. However, from the clinical perspective it would appear sensible to focus initially on the rapid alleviation or remission of depression. This recommendation is based on the differences in the time course of treatment responses; where response to treatment can be expected within 2 to 4 weeks of starting antidepressants and several weeks longer for psychological interventions. By contrast, behavioral changes towards a healthier lifestyle or changes in diabetes treatment leading to better glycemic control take several months until an assessment is feasible. Most important, alleviating depression may be a prerequisite to good diabetes self-management, as people with diabetes are more likely and willing to adhere to their diabetes management if they are in better spirits and are feeling more positive (51).
Text box 2: How to improve the adherence to treatment goals in patients with diabetes and depression treated in primary care? Following a participatory decision-making encounter, it is advantageous to discuss therapy goals together with the patient to heighten their commitment to achieving their goals (146). It is not uncommon, however, for patients to formulate goals that cause the practitioner to worry that ‘diabetes is being neglected’. In such cases, it makes obvious sense to encourage additional diabetes-related targets. However, therapy goals set by healthcare providers are usually only effective when the patient genuinely adopts them. For example, a well-intentioned recommendation to reduce the "HbA1c value by a percentage point" or to become "physically more active" can trigger a socially desirable response in many patients: The patients agreement to the recommendation without actually having decided to commit themselves to this goal. Since they often fail to fulfill this obligation, they often feel guilty or discouraged - factors that can then impair the relationship between the practitioner and patient. To avoid this negative interaction and introduce goals from the practitioner’s perspective, it can be useful to explicitly emphasize that in addition to the patient's goals, a suggestion is made from the practitioner's professional point of view. That goal should be formulated as concretely and as action-oriented as possible to be fulfilled within a specific time frame. Instead of proposing weight loss or lower HbA1c, the specific behaviors that would lead to those conditions should be identified and recommended (147). The patient should then be asked whether they are willing to work toward this objective and how important they find the recommendation. The basic attitude in the conversation should convey serious openness towards the patients‘ potentially different goal. If the patient agrees in principle with the recommended goal, they should be asked what problems are likely to crop up while trying to reach the goal. If the goal seems overly ambitious, the practitioner should attempt to adapt their recommendation to the patient's condition, motivation, and resources as the first step. In the further treatment course the practitioner can then check whether more demanding goals would be acceptable and more apt to be implemented by the patient. |
Models of Care
Various care models are available for treating depression in diabetes, namely conventional consultations, psychopharmacological treatments, psychological interventions and increasingly, telemedical and web-based treatment approaches (51, 148, 149).
The vast majority of the evaluated psychopharmacological treatment used antidepressants, especially selective serotonin reuptake inhibitors (SSRI) and in some cases supplementation with magnesium and vitamin D for patients with corresponding deficiencies.
Psychological interventions delivered by different professional groups include problem-solving techniques, self-management strategies and counseling, cognitive behavioral therapy (CBT) and rarely, psychodynamic therapy. These approaches have also been supplemented by attempts to increase the level of physical activity in depressed patients with type 2 diabetes. The treatment settings of the various interventions ranged from individual or group therapy to complex interventions such as collaborative care or stepped-care approaches.
Collaborative care interventions are interdisciplinary team-based and population-focused approaches (150) in which evidence-based treatment options, the routine monitoring of outcomes, and proactive follow-up contacts are provided. This is supplemented by self-management training and support for patients, the supervision of care managers, and decision support for primary care physicians. On the other hand, stepped-care approaches rely on algorithm-based treatment plans that are individually tailored to the needs and problems of each patient. The fundamental idea behind these models is to offer different step-by-step, evidence-based treatments whereby, based on specific cut-off measurements, those involved decide whether the outcome suffices, or whether the next treatment stage should be initiated. Patients' preferences are also taken into account (148).
Most recent developments in the treatment of depression in diabetes are web-based interventions and mobile health applications that focus on behavioral health coaching and the CBT-oriented self-management of depression (149).
Summary of Meta-Analyses for the Treatment of Depression in Diabetes
Interventions to treat depression in people with diabetes have been systematically evaluated since 1998 in randomized controlled trials (151). Since then, this field of research has been developing rapidly and several meta-analyses or systematic reviews are now available (see table 1). However, considering the summarizing results of the meta-analyses it is important to consider that the underlying individual RCTs show large methodological variations. This includes the type of interventions investigated (face-to-face intervention, telephone or web-based treatment, group versus individual treatment, medication, etc.), comparison conditions, the type of health-care provider service providers (e g, primary, secondary or collaborative care) and the methodological quality of the studies. Altogether this leads to the limited comparability of the available studies. While older meta-analyses or systematic reviews attempted to summarize all available intervention studies in this field (51, 152, 153), meta-analyses for specific interventions (154-157) are now increasingly being published.
In line with the objective of a summarizing description, this chapter does not present individual studies on the treatment of depression in diabetes, but presents all meta-analyses and systematic reviews of RCTs published so far, supplemented by a meta-review from 2015 (51) (see table 1). The results are presented in individual sections for the different interventions. Effect sizes (Cohen`s d and Hedges’ g) are considered as small from 0.2 to 0.5, moderate from 0.5 to 0.8, and large for > 0.8 based on benchmarks suggested by Cohen among others (158, 159).
Psychological Interventions
Summarizing the results of the available meta-analyses on the effectiveness of psychological interventions for the treatment of depressive disorders in people with diabetes, moderate to strong treatment effects can be observed in meta-analyses with some methodological limitations, including the use of combined measures not differentiating between glycemic control and depression symptoms (152) or the non-inclusion of a substantial number of relevant RCTs (155). Meta-analyses or systematic reviews with a more rigorous methodology, on the other hand, tend to indicate only medium effect sizes regarding the reduction of depression intensity and the achievement of remission (51,161).
Two recent meta-analyses (154, 157), analyzed exclusively the effectiveness of CBT in people with diabetes and comorbid depression and included some of the RCTs of the aforementioned meta-analysis updated with newer RCTs. Interestingly, the results show decreasing effects for CBT the longer the follow-up duration of the studies lasts, starting with moderate-to-strong effects in the short-term, small-to-moderate effects in the medium-term and small effects in the long-term follow-up analyses. These data may indicate CBT’s reduced long-term efficacy in diabetes patients with depression compared to depressive people not affected by diabetes (160). Thus, the former assumption that people with diabetes can benefit from CBT as much as depressive people without diabetes is challenged by newer data. It can still be assumed that this group of patients benefits well in the short and medium term, but the long-term effects may be lower than expected due to the impact of the comorbidity with diabetes.
Results of the meta-analyses are inconclusive in terms of improving glycemic control by psychological interventions in people with diabetes and comorbid depression (51, 153-155, 157). CBT may have a short-term positive effect that is no longer detectable in medium and long-term follow-up examinations, as (only) one meta-analysis revealed (157).
In conclusion, psychological interventions including CBT demonstrate moderate effectiveness in reducing depression severity and achieving remission in people with diabetes and depression. Results concerning the improvement in glycemic control, however, are inconsistent and indicate low effectiveness at best.
Pharmacological Treatment
Results from placebo-controlled RCTs on the treatment of depression in people with diabetes with antidepressants (mostly SSRIs) exhibit medium-to-large short-term effects for up to six months. Due to the limited number of follow-up examinations, no conclusions can be made about the medium-to long-term effectiveness of these drugs in comparison with placebo - an important limitation considering depression’s frequent relapse. Studies comparing various antidepressants showed no significant differences in the effectiveness of depression treatment (51, 153).
The results are heterogeneous regarding the amelioration of glycemic control, indicating a slight improvement in HbA1c by selective serotonin reuptake inhibitors in the short term. The comparison of various antidepressants showed no significant difference, with the exception of one trial in which ameliorated glycemic control was observed over the short term in those patients treated with fluoxetine compared with those who took citalopram (51, 153).
In summary, pharmacological interventions are effective in the short-term treatment of depression in diabetes when compared to placebo. Antidepressants demonstrated the most consistent mild-to-moderate effect regarding better glycemic control, but the results remain inconclusive and long-term effects are unknown.
Table 1: Meta-analyses, systematic reviews and meta-review of treatment for depression in diabetes.
Reference |
Methods, number of RCTs and patients, topic of research (within diabetes and depression treatment) |
Results for depression | Results for glycemic control |
Li et al (2017) (154) |
Meta-Analysis (10 RCTs, 998 patients) Topic: Effectiveness of CBT Summary measure Standardized mean differences (SMD) |
Cognitive behavioral therapy Depression severity (7 RCTs) SMD = -0.65 (95%CI -0.98 to -0.31) Depression severity (short-term, 6 RCTs) SMD = -0.86 (95%CI -1.41 to -0.31) Depression severity (long-term, 5 RCTs) SMD = -0.38 (95%CI -0.57 to -0.19) |
Cognitive behavioral therapy HbA1c (7 RCTs) SMD = - 0.22 (95% CI –0.53 to – 0.08) No statistically significant effects |
Xie et al (2017) (155) |
Meta-Analysis (31 RCTs, 2616 patients) Topic: Effectiveness of ‘psychosocial intervention‘(157) (unspecified) Summary measure: SMD |
Psychosocial intervention (unspecified) Note: 23 RCTs written in Chinese and 5 RCTs written in English. Most internationally published RCTs of the other meta-analyses were not included Depression severity (28 RCTs) SMD = -1.50 (95% CI = -1.83 to -1.18). |
Psychosocial intervention (unspecified) Note: 17 RCTs written in Chinese and 5 RCTs written in English. Most internationally published RCTs of the other meta-analyses were missed. HbA1c (22 RCTs) SMD = -0.81 (95% CI =-1.10 to -0.53) |
Reference |
Methods, number of RCTs and patients, topic of research (within diabetes and depression treatment) |
Results for depression | Results for glycemic control |
Uchendu et al (2016) (157) |
Meta-Analysis (12 RCTs, 1445 patients) Topic: Effectiveness of CBT Summary measure: SMD |
Cognitive behavioral therapy Depression severity (short-term, 4 RCTs) SMD = - 0.52 (95% CI –0.79 to – 0.26) Depression severity (medium-term, 5 RCTs) SMD = - 0.43 (95% CI –0.79 to –0.06) Depression severity (long-term, 5 RCTs) SMD = -0.26 (95% CI -0.41 to -0.10) |
Cognitive behavioral therapy HbA1c (short-term, 5 RCTs) SMD = - 0.2 (95% CI –0.5 to – 0.02) No statistically significant effects HbA1c (medium-term, 7 RCTs) SMD = - 0.4 (95% CI –0.6 to – 0.2) HbA1c (long-term, 6 RCTs) SMD = - 0.1 (95% CI –0.3 to – 0.1) No statistically significant effects |
Petrak et al (2015) (51) |
Meta-Review (29 RCTs, 3739 patients) Topics Psychological interventions (12 RCTs, 1647 patients) |
Psychological interventions Depression severity (12 RCTs) SMD from -0.14 to -1.47 |
Psychological interventions HbA1c (7 RCTs) SMD from -0.68 to 0.47 |
Reference |
Methods, number of RCTs and patients, topic of research (within diabetes and depression treatment) |
Results for depression | Results for glycemic control |
Petrak et al (2015) (51) - continued |
Pharmacological interventions (11 RCTs, 470 patients) Psychological vs. pharmacological interventions (1 RCTs, 251 patients) Collaborative/ stepped-care (5 RCTs, 1371 patients) Summary measure: SMD or Odds ratio (OR) |
Pharmacological interventions versus placebo Depression severity (7 RCTs) SMD from -0.25 to -1.65 Comparison between active interventions Fluoxetine versus Paroxetine Remission of depression (1 RCTs) OR = 1.4 (95% CI 0.23 to 8.46) Magnesium supplementation versus Imipramine in depressive patients with a magnesium deficiency Depression severity (1 RCTs) SMD = 0.50 (-3.04 to 4.04) Fluoxetine versus Citalopram Depression severity (1 RCTs) SMD = 0.40 (-1.43 to 2.23) |
Pharmacological interventions versus placebo HbA1c (short-term, 6 RCTs) SMD from -0.10 to -0.98 Comparison between active interventions Fluoxetine versus paroxetine (1 RCTs) Not reported Magnesium supplementation versus Imipramine in depressive patients with a magnesium deficiency (1 RCTs) SMD = -0.10 (-1.24 to 1.04) Fluoxetine versus Citalopram (1 RCTs) SMD = -1.00 (-1.85 to -0.15) |
Reference |
Methods, number of RCTs and patients, topic of research (within diabetes and depression treatment) |
Results for depression | Results for glycemic control |
Petrak et al (2015) (51) - continued |
Diabetes-specific CBT versus Sertraline Depression severity (1 RCT) SMD = -0.39 (-0.02 to 0.76), not significant Collaborative or stepped-care interventions Depression severity: SMD from -0.13 to -0.68 |
Diabetes-specific CBT versus Sertraline (1 RCT) SMD = -0.12 (-0.49 to 0.24), not significant Collaborative or stepped-care interventions SMD from 0.00 to -0.54 |
|
Atlantis et al (2014) (156) |
Meta-Analysis (7 RCTs, 1895 patients, including 2 RCT with mixed samples comprising diabetes patients) Topic: Effectiveness of collaborative care Summary measures Depression severity: SMD HbA1c: Weighted mean difference (WMD) |
Collaborative care vs. usual care Depression severity (short-to-medium term, (7 RCTs) |
Collaborative care vs. usual care HbA1c (short-to-medium term, 7 RCTs) WMD = - 0.33% (95% CI −0.66% to −0.00%) |
Reference | Methods, number of RCTs and patients, topic of research (within diabetes and depression treatment) |
Results for depression | Results for glycemic control | ||||||
Baumeister et al (2012) (153) |
Cochrane Systematic Review (19 RCTs, 1592 patients) |
Psychological interventions Depression severity (8 RCTs) SMD from -1.47 to -0.14 |
Psychological interventions HbA1c (short-term, 4 RCTs) MD = -0.4% (95% CI -0.6 to -0.1) |
||||||
Topic: Effectiveness of psychotherapy, antidepressant medication and collaborative care Psychological interventions (8 RCTs, 1122 patients) Pharmacological interventions vs. placebo (8 RCTs, 377 patients) Pharmacological interventions vs other pharmacological interventions (3 RCTs, 93 patients)
|
Remission of depression (short-term, 4 RCTs) OR = 2.88 (95% CI 1.58 to 5.25). Remission of depression (medium-term, 2 RCTs) OR = 2.49 (95% CI 1.44 to 4.32) Pharmacological interventions vs. placebo Depression severity (7 RCTs): SMD = -0.61 (95% CI -0.94 to -0.27) Remission of depression (short-term, 3 RCTs) OR = 2.50 (95% CI 1.21 to 5.15). |
Pharmacological interventions vs. placebo HbA1c (short-term): MD = -0.4% (95% CI -0.6 to -0.1) Pharmacological interventions vs other pharmacological interventions No significant differences between the examined pharmacological agents, Exeption in one trial (Fluoxetine versus Citalopram) HbA1c (short-term): MD = -1.0% (95% CI -1.9 to -0.2) |
|||||||
Reference | Methods, number of RCTs and patients, topic of research (within diabetes and depression treatment) |
Results for depression |
Results for glycemic control
|
||||||
Baumeister et al (2012) (153) - continued |
Summary measures HbA1c: Mean differences (MD) Depression severity: SMD Depression remission: OR |
Pharmacological interventions vs other pharmacological interventions No significant differences between the examined pharmacological agents (3 RCTs) |
Pharmacological interventions vs other pharmacological interventions No significant differences between the examined pharmacological agents (3 RCTs) |
||||||
van der Feltz-Cornelis et al (2010) (152) |
Meta-Analysis (14 RCTs, 1724 patients) Topic: Effectiveness of psychotherapy, antidepressant medication and collaborative care Summary measures Effect sizes (Cohen's d) for a combined outcome measure (depressive symptoms and HbA1c together) |
Estimate of interventions on combined outcomes not differentiating between depression symptoms and HbA1c Psychotherapeutic interventions Cohen's d: - 0.581 (95% CI −0.770 to −0.391) Pharmacological interventions Cohen's d: - 0.467 (95% CI −0.665 to −0.270) Collaborative care interventions Cohen's d: - 0.292 (95% CI −0.429 to −0.155) |
|||||||
Psychopharmacological vs. Psychological Interventions
So far, there has only been one RCT in people with diabetes and co-morbid depression in which the efficacy of an antidepressant, in this case sertraline, was compared to a psychological intervention, in this case CBT (51, 161).
The study was conducted in a secondary-care setting and compared the effectiveness of effects of diabetes-specific CBT for 12 weeks compared to sertraline treatment in patients with poorly controlled diabetes and major depression. Continuous treatment to prevent relapse of depression was provided for an additional 12 months in the sertraline group. In the CBT group, investigators assumed that carry-over effects would stabilize the results without further treatment. Both sertraline and CBT reduced depression severity at the end of treatment, but sertraline was more effective than CBT in preventing relapse at the 1-year follow-up for patients who remitted with treatment. Poor glycemic control remained nearly unchanged during the entire trial in both intervention groups. The authors concluded that CBT and sertraline as single treatment are insufficient to treat diabetes patients with depression and poor glycemic control in secondary care (161).
Collaborative Care and Stepped-Care Approaches
Meta-analyses for collaborative or stepped-care interventions have shown a considerable between-study heterogeneity and altogether have demonstrated moderate effectiveness compared to usual care in alleviating the depression severity in people with diabetes and depression. The targeted improvement in glycemic control revealed small-to-moderate effectiveness in the short-to-medium term (51, 152, 153, 156)
In summary: collaborative and stepped-care approaches demonstrating a slight-to-moderate positive influence on depression and glycemic control.
Critical Appraisal of the Current Evidence
Considering the current state of research on the treatment of depression in people with diabetes, we notice that despite considerably more intensive research, we have gained little knowledge in recent years.
There is ample evidence of the moderate efficacy of psychological or psychopharmacological interventions in alleviating depressive symptoms. However, it should be emphasized that only the short-term efficacy of drug interventions has been well investigated, whereas there are practically no medium- and long-term study results. Combined therapies have been assessed particularly in the USA. In these so-called "collaborative care" and "stepped care" approaches, psychological and drug treatments were flexibly offered or combined according to certain algorithms and depending on the response to individual treatment steps. This study design does not enable us to identify effective components of a treatment and/or to test the superiority of one component over another. The only thing that can be predicted is whether this combined treatment approach offers advantages in contrast to a standard treatment, which has repeatedly been demonstrated with moderate effect sizes.
Studies investigating CBT have usually applied more stringent research methodology than have other RCTs of psychological interventions in the field of diabetes research. Recent meta-analytical summaries of these studies showed that CBT’s effect becomes weaker the longer the study lasts; it thus appears to be less effective than in depressed people with diabetes than in those without it.
The evidence of improved glycemic control has been far more heterogeneous and contradictory, especially regarding psychological interventions. RCTs that reported a positive influence on HbA1c, yielded at best modest-to-moderate effects and included SSRIs and partly collaborative care interventions. For patients in secondary-care settings with very poor glycemic control, neither CBT nor sertraline led to better glycemic control
The generalizability of the available studies is limited by sample selection effects and other methodological limitations. Claims about the treatment of patients in secondary (specialized practices) and tertiary care (in-patient treatment) can hardly be made, as almost all studies were conducted in primary care settings. As a further limitation, it should be noted that most of these studies were conducted in countries with comparatively advanced health care systems, i.e., Europe and the US, while the vast majority of people with diabetes live in low- and middle-income countries (171) with different cultures and under other socioeconomic conditions and healthcare systems – all factors that limit the applicability of the current scientific evidence to those countries. Most important, since comparative therapy studies between active interventions for this group of patients have been so few, the question as to which is the most effective treatment method remains unanswered.
Future research needs to be more specific in describing the characteristics of participants (e.g., primary vs. secondary care; good vs. poor glycemic control, etc.) instead of perpetuating the general conclusion that all examined interventions are more or less effective for all people with diabetes and depression. More knowledge is needed about which are the most effective components within complex interventions, and about treatment duration and dosage regimens. Finally, it is of particularly importance to develop suitable communication strategies that allow us to reach those patient groups with special needs, and to adapt treatments according to the requirements of the specific clinical setting, culture, and country.
RECOMMENDATIONS FOR CLINICAL PRACTICE
The implementation of medical standards is constrained by limited resources in many parts of the developing world, leading to different levels of medical care worldwide. The International Diabetes Federation (IDF) classifies levels of care as ranging from ‘limited care’ (with insufficient medical resources and too little qualified medical staff), to ‘recommended care’ (evidence-based cost-effective care), and finally, ‘comprehensive care’ (most up-to-date, all necessary health technologies available) (75). Until now, only one evidence-based guideline specifically addressing the treatment for depression in people with diabetes has been published in Germany within a healthcare system at the IDF level of ‘recommended’ or ‘comprehensive care’ (145, 162). Other guidelines from the UK National Institute for Health and Care Excellence (NICE) address the treatment of depression in people with chronic physical disorders, including patients with diabetes (163).
There is still insufficient scientific evidence to make concrete recommendations regarding the treatment of depression in diabetes patients with specific characteristics such as the type of diabetes, quality of glycemic control, diabetes complications, etc. Nevertheless, such characteristics must be considered when treating individual patients. Diabetes therapy as such does not differ from the treatment of non-depressive patients with comorbid depression, and should follow national and international guidelines, e.g., (164-166).
Safety Precautions for the Psychopharmacological Treatment of Depression in
Patients with Diabetes
Treatment with antidepressants, especially in patients with diabetes, requires particular attention to the side effects and contraindications, as well as potential interactions with glucose metabolism and other drugs. For this reason, the guidelines "Psychosocial and Diabetes Mellitus" of the German Diabetes Association (145), which also incorporate recommendations from the German National Depression Guidelines (167), make specific recommendations regarding drug-based antidepressant therapy.
In particular they warned against tricyclic antidepressants, as well as mirtazapine and mianserin. These drugs can raise blood sugar levels and promote weight gain, which should be avoided in patients with type 2 diabetes. Due to their problematic cardiac profile, patients with heart disease and diabetes are explicitly warned against taking tricyclic antidepressants. Furthermore, the potential of increased insulin sensitivity due to SSRIs and of hypoglycemia, which may require a change in the insulin dose, is also noted. With regards to elderly patients with diabetes, the guidelines recommend that interactions with other drugs be given special attention, as polypharmacy is a frequent an issue due to other co-morbidities. Particular attention is drawn to the interaction within the cytochrome P450 system, which can lead to alterations in drug concentrations.
Furthermore, it must be assumed that life-threatening polymorphic ventricular tachyarrhythmias can be triggered by antidepressant medication (especially in the presence of other risk factors) by prolonging the QTc interval in individual cases. This includes tri- and tetracyclic antidepressants as well as citalopram, escitalopram, fluoxetine, paroxetine, and venlafaxine. Cardiac contraindications must be taken into account. In particular, based on the manufacturers' warnings, prescriptions for citalopram and escitalopram should be very carefully considered when treating patients with heart disease; those who present the additional risk of a QT interval prolongation should not be given those drugs at all.
A special warning is made against combining certain substances that can lead to an extension of the QT interval. If the benefits are weighed against the risks, SSRIs are considered the first-choice antidepressant medication for patients with diabetes. Risk-benefit analysis should be carried out before prescribing any psychotropic drug, especially when additional cardiac risk factors are known (145).
A Practice-Oriented Stepped-Care Model for the Treatment of Depression in Patients with Diabetes
In the following, we present a stepped-care model of the treatment of depression in patients with diabetes developed based on current scientific evidence and guidelines from the German Diabetes Society (51, 145); it can be adapted to specific levels of care (see figure 1). The treatment steps are determined by the severity and duration of depressive symptoms and are summarized in four stages. During therapy, it is recommended to regularly monitor response and suicidal ideation through all phases and, depending on the severity and development of depression, the intervention step should be changed. This stepped-care approach should enable flexible and individually-adjustable treatment with the overall goal of complete remission of depression.
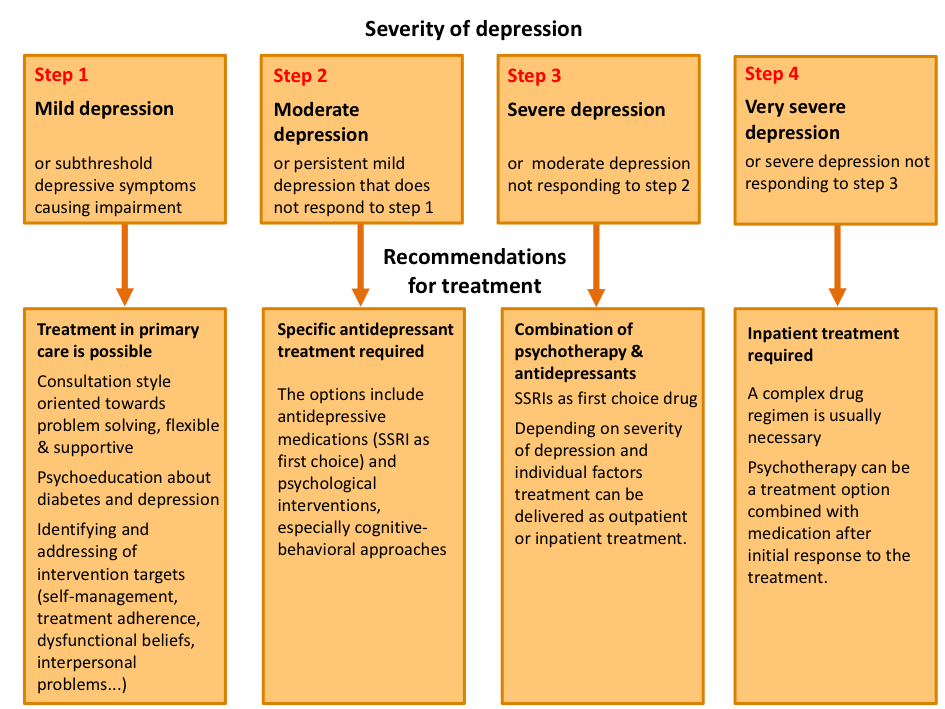
Figure 1: Model of stepped care for the treatment of depression in diabetes
adapted from (51, 145, 162)
1) Mild depression (or impairing subthreshold depressive symptoms):
A depression of this severity can usually be treated within the framework of primary care, provided there are no acute crises or suicidal thoughts. The consultation style should be flexible and supportive, and oriented towards problem-solving. It is important to inform patients about their depression and the potential links between depression and diabetes. If possible, individual explanations should be worked out with the patient from which starting points for different interventions can be derived. These could be, for example, identifying dysfunctional thoughts, interpersonal problems, or insufficient adherence to diabetes therapy. Self-help materials or web-based self-help tools for depression can be a useful to overcome the depression at this stage.
If there is a history of severe recurrent depression, SSRI medication or psychotherapy should be considered already at this stage of treatment. The course of the depressive symptoms should be carefully monitored over a 2- to 4-week period. If there is no improvement by then, the next step must be initiated, which is also the starting point for moderate depression.
2) Moderate depression (or persistent mild depression not responding to step 1):
Depression of moderate severity requires specific treatment, and the patient should be informed of the various treatment options so that informed decision-making is facilitated.
Cognitive behavioral therapy is considered the first-choice psychotherapeutic treatment, whereas SSRIs should be given priority in psychopharmacological treatment. The combination of medication and psychotherapy is particularly recommended for recurrent depressive disorders. Psychopharmacological treatment requires continued monitoring so that a change in dosage or medication can be considered after 2-4 weeks of treatment if the response remains insufficient. Psychotherapeutic treatment should also be monitored, but with individualized time frames for the transition to the next treatment stage, which must be initiated if the treatment outcome is inadequate.
3) Severe depression (or moderate depression not responding to step 2):
With this severity level, the treatment options include antidepressants, which are often combined with psychotherapy or psychotherapy without medication. Depending on the patient’s individual requirements, outpatient or inpatient therapy can be carried out.
4) Very severe depression (or severe depression not responding to step 3):
In case of a very severe depression, psychotherapy will usually not suffice and is often impossible. Treatment should usually be provided on an in-patient basis and often involves a complex medication regime. Depending on the patient’s individual requirements, psychotherapy can be offered concomitantly or frequently after an initial improvement through medication.
CONCLUSION
The comorbidity of diabetes and depression remains a considerable clinical challenge for patients and healthcare professionals. While the means of screening and diagnosing depressive disorders in people with diabetes have improved significantly in recent years, this does not automatically lead to better care, as clear treatment pathways after a positive diagnosis are often lacking or are rejected by the affected individuals.
Taken together, the scientific evidence on various treatments for depression in diabetes is somewhat encouraging, as it demonstrates that depression is treatable with moderate to good results in depressed people with diabetes. However, we still need efficient treatments to achieve simultaneous a long-term improvement in glycemic control.
A positive development is that the awareness of the importance of depression in diabetes has grown, and that many treatment alternatives for patients are now available. Although the scientific evidence still has much room for improvement in this area of research, there are many options for individualized treatment that give cause for optimism for the individual patient.
REFERENCES
- Fisher L, Gonzalez JS, Polonsky WH. The confusing tale of depression and distress in patients with diabetes: a call for greater clarity and precision. Diabetic Medicine. 2014;31(7):764–72.
- Snoek FJ, Bremmer MA, Hermanns N. Constructs of depression and distress in diabetes: time for an appraisal. Lancet Diabetes Endocrinol. 2015 Jun;3(6):450-60. PubMed PMID: 25995123.
- Cepoiu M, McCusker J, Cole MG, Sewitch M, Belzile E, Ciampi A. Recognition of depression by non-psychiatric physicians--a systematic literature review and meta-analysis. J Gen Intern Med. 2008 Jan;23(1):25-36. PubMed PMID: 17968628. Pubmed Central PMCID: PMC2173927.
- Li C, Ford ES, Zhao G, Ahluwalia IB, Pearson WS, Mokdad AH. Prevalence and correlates of undiagnosed depression among U.S. adults with diabetes: the Behavioral Risk Factor Surveillance System, 2006. Diabetes Res Clin Pract. 2009 Feb;83(2):268-79. PubMed PMID: 19111364. Epub 2008/12/30. eng.
- Katon WJ, Simon G, Russo J, Von Korff M, Lin EH, Ludman E, et al. Quality of depression care in a population-based sample of patients with diabetes and major depression. Medical care. 2004 Dec;42(12):1222-9. PubMed PMID: 15550802. Epub 2004/11/20. eng.
- World°Health°Organization. The ICD-10 classification of mental and behavioural disorders: Clinical descriptions and diagnostic guidelines. Geneva. http://www.who.int/classifications/icd/en/bluebook.pdf1992.
- World°Health°Organization. The ICD-10 classification of mental and behavioural disorders: Diagnostic criteria for research. Geneva. http://www.who.int/classifications/icd/en/GRNBOOK.pdf 1993.
- Anderson RJ, Freedland KE, Clouse RE, Lustman PJ. The prevalence of comorbid depression in adults with diabetes: a meta-analysis. Diabetes Care. 2001 Jun;24(6):1069-78. PubMed PMID: 11375373. Epub 2001/05/26. eng.
- Hasan SS, Mamun AA, Clavarino AM, Kairuz T. Incidence and risk of depression associated with diabetes in adults: evidence from longitudinal studies. Community Ment Health J. 2015 Feb;51(2):204-10. PubMed PMID: 24951962.
- Moulton CD, Pickup JC, Ismail K. The link between depression and diabetes: the search for shared mechanisms. Lancet Diabetes Endocrinol. 2015 Jun;3(6):461-71. PubMed PMID: 25995124.
- Fisher L, Hessler DM, Polonsky WH, Masharani U, Peters AL, Blumer I, et al. Prevalence of depression in Type 1 diabetes and the problem of over-diagnosis. Diabet Med. 2016 Nov;33(11):1590-7. PubMed PMID: 26433004.
- Roy T, Lloyd CE. Epidemiology of depression and diabetes: a systematic review. J Affect Disord. 2012 Oct;142 Suppl:S8-21. PubMed PMID: 23062861. Epub 2012/10/25. eng.
- Jacob L, Kostev K. Prevalence of depression in type 2 diabetes patients in German primary care practices. J Diabetes Complications. 2016 Apr;30(3):432-7. PubMed PMID: 26790576.
- Barnard KD, Skinner TC, Peveler R. The prevalence of co-morbid depression in adults with Type 1 diabetes: systematic literature review. Diabet Med. 2006 Apr;23(4):445-8. PubMed PMID: 16620276.
- Ali S, Stone MA, Peters JL, Davies MJ, Khunti K. The prevalence of co-morbid depression in adults with Type 2 diabetes: a systematic review and meta-analysis. Diabet Med. 2006 Nov;23(11):1165-73. PubMed PMID: 17054590. Epub 2006/10/24. eng.
- Egede LE, Ellis C. Diabetes and depression: global perspectives. Diabetes research and clinical practice. 2010 Mar;87(3):302-12. PubMed PMID: 20181405. Epub 2010/02/26. eng.
- Chen S, Zhang Q, Dai G, Hu J, Zhu C, Su L, et al. Association of depression with pre-diabetes, undiagnosed diabetes, and previously diagnosed diabetes: a meta-analysis. Endocrine. 2016 Jul;53(1):35-46. PubMed PMID: 26832340.
- Cosgrove MP, Sargeant LA, Griffin SJ. Does depression increase the risk of developing type 2 diabetes? Occup Med (Lond). 2008 Jan;58(1):7-14. PubMed PMID: 17965449.
- Knol MJ, Twisk JW, Beekman AT, Heine RJ, Snoek FJ, Pouwer F. Depression as a risk factor for the onset of type 2 diabetes mellitus. A meta-analysis. Diabetologia. 2006 May;49(5):837-45. PubMed PMID: 16520921. Epub 2006/03/08. eng.
- Rotella F, Mannucci E. Depression as a risk factor for diabetes: a meta-analysis of longitudinal studies. J Clin Psychiatry. 2013 Jan;74(1):31-7. PubMed PMID: 23419223. Epub 2013/02/20. eng.
- Mezuk B, Eaton WW, Albrecht S, Golden SH. Depression and type 2 diabetes over the lifespan: a meta-analysis. Diabetes Care. 2008 Dec;31(12):2383-90. PubMed PMID: 19033418. Pubmed Central PMCID: 2584200. Epub 2008/11/27. eng.
- Yu M, Zhang X, Lu F, Fang L. Depression and Risk for Diabetes: A Meta-Analysis. Can J Diabetes. 2015 Mar 13. PubMed PMID: 25773933.
- Lustman PJ, Anderson RJ, Freedland KE, de Groot M, Carney RM, Clouse RE. Depression and poor glycemic control: a meta-analytic review of the literature. Diabetes Care. 2000;23(7):934-42.
- Silverman J, Krieger J, Kiefer M, Hebert P, Robinson J, Nelson K. The Relationship Between Food Insecurity and Depression, Diabetes Distress and Medication Adherence Among Low-Income Patients with Poorly-Controlled Diabetes. J Gen Intern Med. 2015 Oct;30(10):1476-80. PubMed PMID: 25917659. Pubmed Central PMCID: PMC4579205.
- Zhang Y, Ting RZ, Yang W, Jia W, Li W, Ji L, et al. Depression in Chinese patients with type 2 diabetes: associations with hyperglycemia, hypoglycemia, and poor treatment adherence. J Diabetes. 2015 Nov;7(6):800-8. PubMed PMID: 25349949. Pubmed Central PMCID: PMC4964948.
- De Groot M, Anderson R, Freedland K, Clouse R, Lustman P. Association of depression and diabetes complications: a meta-analysis. Psychosom Med. 2001;63(4):619 - 30.
- Katon WJ, Rutter C, Simon G, Lin EH, Ludman E, Ciechanowski P, et al. The association of comorbid depression with mortality in patients with type 2 diabetes. Diabetes Care. 2005 Nov;28(11):2668-72. PubMed PMID: 16249537.
- Black SA, Markides KS, Ray LA. Depression Predicts Increased Incidence of Adverse Health Outcomes in Older Mexican Americans With Type 2 Diabetes. Diabetes Care. 2003 October 1, 2003;26(10):2822-8.
- Ismail K, Winkley K, Stahl D, Chalder T, Edmonds M. A cohort study of people with diabetes and their first foot ulcer: the role of depression on mortality. Diabetes Care. 2007 Jun;30(6):1473-9. PubMed PMID: 17363754.
- Richardson LK, Egede LE, Mueller M. Effect of race/ethnicity and persistent recognition of depression on mortality in elderly men with type 2 diabetes and depression. Diabetes Care. 2008 May;31(5):880-1. PubMed PMID: 18332155. Epub 2008/03/12. eng.
- Bot M, Pouwer F, Zuidersma M, van Melle JP, de Jonge P. Association of coexisting diabetes and depression with mortality after myocardial infarction. Diabetes Care. 2012 Mar;35(3):503-9. PubMed PMID: 22301118. Pubmed Central PMCID: PMC3322704.
- Pan A, Lucas M, Sun Q, van Dam RM, Franco OH, Willett WC, et al. Increased mortality risk in women with depression and diabetes mellitus. Arch Gen Psychiatry. 2011 Jan;68(1):42-50. PubMed PMID: 21199964. Pubmed Central PMCID: 3081788. Epub 2011/01/05. eng.
- Naicker K, Johnson JA, Skogen JC, Manuel D, Overland S, Sivertsen B, et al. Type 2 Diabetes and Comorbid Symptoms of Depression and Anxiety: Longitudinal Associations With Mortality Risk. Diabetes Care. 2017 Mar;40(3):352-8. PubMed PMID: 28077458.
- Moussavi S, Chatterji S, Verdes E, Tandon A, Patel V, Ustun B. Depression, chronic diseases, and decrements in health: results from the World Health Surveys. Lancet. 2007 Sep 8;370(9590):851-8. PubMed PMID: 17826170. Epub 2007/09/11. eng.
- Hermanns N, Kulzer B, Krichbaum M, Kubiak T, Haak T. How to screen for depression and emotional problems in patients with diabetes: comparison of screening characteristics of depression questionnaires, measurement of diabetes-specific emotional problems and standard clinical assessment. Diabetologia. 2006;49(3):469–77.
- Anderson JR, Novak JR, Johnson MD, Deitz SL, Walker A, Wilcox A, et al. A dyadic multiple mediation model of patient and spouse stressors predicting patient dietary and exercise adherence via depression symptoms and diabetes self-efficacy. J Behav Med. 2016 Dec;39(6):1020-32. PubMed PMID: 27696127.
- Lin EH, Katon W, Von Korff M, Rutter C, Simon GE, Oliver M, et al. Relationship of depression and diabetes self-care, medication adherence, and preventive care. Diabetes Care. 2004 Sep;27(9):2154-60. PubMed PMID: 15333477. Epub 2004/08/31. eng.
- Gonzalez JS, Peyrot M, McCarl LA, Collins EM, Serpa L, Mimiaga MJ, et al. Depression and diabetes treatment nonadherence: a meta-analysis. Diabetes Care. 2008 Dec;31(12):2398-403. PubMed PMID: 19033420. Pubmed Central PMCID: 2584202. Epub 2008/11/27. eng.
- Sumlin LL, Garcia TJ, Brown SA, Winter MA, Garcia AA, Brown A, et al. Depression and Adherence to Lifestyle Changes in Type 2 Diabetes: A Systematic Review. Diabetes Educ. 2014 Jun 17. PubMed PMID: 24939883. Epub 2014/06/19. Eng.
- Katon W, Simon G, Russo J, Von Korff M, Lin E, Ludman E, et al. Quality of depression care in a population-based sample of patients with diabetes and major depression. Med Care. 2004;42(12):1222-9.
- Cepoiu M, McCusker J, Cole MG, Sewitch M, Belzile E, Ciampi A. Recognition of depression by non-psychiatric physicians--a systematic literature review and meta-analysis. J Gen Intern Med. 2008 Jan;23(1):25-36. PubMed PMID: 17968628. Pubmed Central PMCID: 2173927. Epub 2007/10/31. eng.
- Lotfi L, Flyckt L, Krakau I, Martensson B, Nilsson GH. Undetected depression in primary healthcare: occurrence, severity and co-morbidity in a two-stage procedure of opportunistic screening. Nord J Psychiatry. 2010 Dec;64(6):421-7. PubMed PMID: 20429746.
- Musselman DL, Betan E, Larsen H, Phillips LS. Relationship of depression to diabetes types 1 and 2: epidemiology, biology, and treatment. Biol Psychiatry. 2003 Aug 1;54(3):317-29. PubMed PMID: 12893107. Epub 2003/08/02. eng.
- Sultan S, Luminet O, Hartemann A. Cognitive and anxiety symptoms in screening for clinical depression in diabetes: a systematic examination of diagnostic performances of the HADS and BDI-SF. J Affect Disord. 2010 Jun;123(1-3):332-6. PubMed PMID: 19861228. Epub 2009/10/29. eng.
- Petrak F. [Depression and diabetes mellitus. A dangerous vicious circle] In: F. Petrak, F. & S. Herpertz (Eds.) [Psychodiabetologie], 127-144. Springer: Heidelberg. 2013.
- First MB, Williams JBW, Spitzer RL, Gibbon M. Structured Clinical Interview for DSM-IV-TR Axis I Disorders, Clinical Trials Version (SCID-CT) New York: Biometrics Research, New York State Psychiatric Institute; 2007.
- Wing JK, Babor T, Brugha T, Burke J, Cooper JE, Giel R, et al. SCAN. Schedules for Clinical Assessment in Neuropsychiatry. Arch Gen Psychiatry. 1990 Jun;47(6):589-93. PubMed PMID: 2190539. Epub 1990/06/01. eng.
- Twist K, Stahl D, Amiel SA, Thomas S, Winkley K, Ismail K. Comparison of depressive symptoms in type 2 diabetes using a two-stage survey design. Psychosom Med. 2013 Oct;75(8):791-7. PubMed PMID: 23922402. Epub 2013/08/08. eng.
- Roy T, Lloyd CE, Pouwer F, Holt RIG, Sartorius N. Screening tools used for measuring depression among people with Type 1 and Type 2 diabetes: a systematic review. Diabetic Medicine. 2012;29(2):164–75.
- Lowe B, Kroenke K, Grafe K. Detecting and monitoring depression with a two-item questionnaire (PHQ-2). J Psychosom Res. 2005 Feb;58(2):163-71. PubMed PMID: 15820844. Epub 2005/04/12. eng.
- Petrak F, Baumeister H, Skinner TC, Brown A, Holt RI. Depression and diabetes: treatment and health-care delivery. Lancet Diabetes Endocrinol. 2015 Jun;3(6):472-85. PubMed PMID: 25995125.
- Beck AT, Ward CH, Mendelson M, Mock J, Erbaugh J. An inventory for measuring depression. Arch Gen Psychiatry. 1961 Jun;4:561-71. PubMed PMID: 13688369. Epub 1961/06/01. eng.
- Beck AT, Steer RA, Brown GK. Manual for the Beck Depression Inventory-II. San Antonio: Psychological Corporation; 1996.
- Radloff LS. The CES-D scale: a self-report depression scale for research in the general population. Appl Psychol Meas. 1977;1:385-401.
- Zigmond AS, Snaith RP. The hospital anxiety and depression scale. Acta Psychiatr Scand. 1983 Jun;67(6):361-70. PubMed PMID: 6880820. Epub 1983/06/01. eng.
- Spitzer RL, Kroenke K, Williams JB. Validation and utility of a self-report version of PRIME-MD: the PHQ primary care study. Primary Care Evaluation of Mental Disorders. Patient Health Questionnaire. Jama. 1999 Nov 10;282(18):1737-44. PubMed PMID: 10568646. Epub 1999/11/24. eng.
- Bech P. Health-related quality of life measurements in the assessment of pain clinic results. Acta Anaesthesiol Scand. 1999 Oct;43(9):893-6. PubMed PMID: 10522736.
- Halliday JA, Hendrieckx C, Busija L, Browne JL, Nefs G, Pouwer F, et al. Validation of the WHO-5 as a first-step screening instrument for depression in adults with diabetes: Results from Diabetes MILES - Australia. Diabetes Res Clin Pract. 2017 Oct;132:27-35. PubMed PMID: 28783530.
- McHale M, Hendrikz J, Dann F, Kenardy J. Screening for depression in patients with diabetes mellitus. Psychosom Med. 2008 Oct;70(8):869-74. PubMed PMID: 18842744. Epub 2008/10/10. eng.
- Stahl D, Sum CF, Lum SS, Liow PH, Chan YH, Verma S, et al. Screening for depressive symptoms: validation of the center for epidemiologic studies depression scale (CES-D) in a multiethnic group of patients with diabetes in Singapore. Diabetes Care. 2008 Jun;31(6):1118-9. PubMed PMID: 18337303. Epub 2008/03/14. eng.
- Holt RI, van der Feltz-Cornelis CM. Key concepts in screening for depression in people with diabetes. J Affect Disord. 2012 Oct;142 Suppl:S72-9. PubMed PMID: 23062860. Epub 2012/10/25. eng.
- Kroenke K, Spitzer RL, Williams JB, Lowe B. The Patient Health Questionnaire Somatic, Anxiety, and Depressive Symptom Scales: a systematic review. Gen Hosp Psychiatry. 2010 Jul-Aug;32(4):345-59. PubMed PMID: 20633738. Epub 2010/07/17. eng.
- Janssen EP, Kohler S, Stehouwer CD, Schaper NC, Dagnelie PC, Sep SJ, et al. The Patient Health Questionnaire-9 as a Screening Tool for Depression in Individuals with Type 2 Diabetes Mellitus: The Maastricht Study. J Am Geriatr Soc. 2016 Nov;64(11):e201-e6. PubMed PMID: 27783384.
- Gilbody S, Sheldon T, Wessely S. Should we screen for depression? BMJ. 2006;332(7548):1027–30.
- Gilbody S, Sheldon T, House A. Screening and case-finding instruments for depression: a meta-analysis. Canadian Medical Association Journal. 2008;178(8):997–1003.
- Hermanns N, Caputo S, Dzida G, Khunti K, Meneghini LF, Snoek F. Screening, evaluation and management of depression in people with diabetes in primary care. Prim Care Diabetes. 2013;7(1):1–10.
- Pouwer F, Tack CJ, Geelhoed-Duijvestijn PH, Bazelmans E, Beekman AT, Heine RJ, et al. Limited effect of screening for depression with written feedback in outpatients with diabetes mellitus: a randomised controlled trial. Diabetologia. 2011 Apr;54(4):741-8. PubMed PMID: 21221528. Pubmed Central PMCID: 3052512. Epub 2011/01/12. eng.
- Scollan-Koliopoulos M, Herrera I, Romano K, Gregory C, Rapp K, Bleich D. Healthcare technician delivered screening of adults with diabetes to improve primary care provider recognition of depression. J Family Med Prim Care. 2012 Jul;1(2):97-102. PubMed PMID: 24479015. Pubmed Central PMCID: 3893961. Epub 2012/07/01. eng.
- Simon GE. Evidence review: efficacy and effectiveness of antidepressant treatment in primary care. Gen Hosp Psychiatry. 2002 Jul-Aug;24(4):213-24. PubMed PMID: 12100832. Epub 2002/07/09. eng.
- Hayashino Y, Suzuki H, Yamazaki K, Izumi K, Noda M, Kobayashi M. Depressive symptoms, not completing a depression screening questionnaire, and risk of poor compliance with regular primary care visits in patients with type 2 diabetes: The Japan Diabetes Outcome Intervention Trial 2 (J-DOIT2) Study Group. Experimental and Clinical Endocrinology & Diabetes. 2011;119(05):276–80.
- Fleer J, Tovote KA, Keers JC, Links TP, Sanderman R, Coyne JC, et al. Screening for depression and diabetes-related distress in a diabetes outpatient clinic. Diabet Med. 2013 Jan;30(1):88-94. PubMed PMID: 22924587. Epub 2012/08/29. eng.
- Schierhout G, Nagel T, Si D, Connors C, Brown A, Bailie R. Do competing demands of physical illness in type 2 diabetes influence depression screening, documentation and management in primary care: a cross-sectional analytic study in Aboriginal and Torres Strait Islander primary health care settings. Int J Ment Health Syst. 2013;7(1):16. PubMed PMID: 23738766. Pubmed Central PMCID: 3681658. Epub 2013/06/07. eng.
- Alderson SL, Russell AM, McLintock K, Potrata B, House A, Foy R. Incentivised case finding for depression in patients with chronic heart disease and diabetes in primary care: an ethnographic study. BMJ Open. 2014;4(8):e005146.
- UK°National°Screening°Committee. Criteria for appraising the viability, effectiveness and appropriateness of a screening programme. 2011.
- IDF°Clinical°Guidelines°Task°Force. Global Guidelines for Type 2 Diabetes 2012 [2014 Nov. 12]. Available from: http://www.idf.org/sites/default/files/IDF-Guideline-for-Type-2-Diabetes.pdf.
- Ell K, Katon W, Xie B, Lee PJ, Kapetanovic S, Guterman J, et al. Collaborative care management of major depression among low-income, predominantly Hispanic subjects with diabetes: a randomized controlled trial. Diabetes Care. 2010 Apr;33(4):706-13. PubMed PMID: 20097780. Pubmed Central PMCID: 2845010. Epub 2010/01/26. eng.
- Katon WJ, Lin EH, Von Korff M, Ciechanowski P, Ludman EJ, Young B, et al. Collaborative care for patients with depression and chronic illnesses. N Engl J Med. 2010 Dec 30;363(27):2611-20. PubMed PMID: 21190455. Pubmed Central PMCID: 3312811. Epub 2010/12/31. eng.
- Polonsky W, Anderson B, Lohrer P, Welch G, Jacobson A, Aponte J, et al. Assessment of diabetes-related distress. Diabetes Care. 1995;18(6):754 - 60. PubMed PMID: doi:10.2337/diacare.18.6.754.
- Lowe B, Schenkel I, Carney-Doebbeling C, Gobel C. Responsiveness of the PHQ-9 to Psychopharmacological Depression Treatment Psychosomatics. 2006 Jan-Feb;47(1):62-7 ( Download at http://www.cqaimh.org/pdf/tool_phq9.pdf). PubMed PMID: 16384809. Epub 2005/12/31. eng.
- Whooley MA, Avins AL, Miranda J, Browner WS. Case-finding instruments for depression. Two questions are as good as many. J Gen Intern Med. 1997 Jul;12(7):439-45. PubMed PMID: 9229283.
- Lawton J, Waugh N, Barnard KD, Noyes K, Harden J, Stephen J, et al. Challenges of optimizing glycaemic control in children with Type 1 diabetes: a qualitative study of parents' experiences and views. Diabet Med. 2015 Aug;32(8):1063-70. PubMed PMID: 25472898.
- Worrall-Davies A, Owens D, Holland P, Haigh D. The effect of parental expressed emotion on glycaemic control in children with Type 1 diabetes. Parental expressed emotion and glycaemic control in children. J Psychosom Res. 2002 Feb;52(2):107-13. PubMed PMID: 11832256.
- Armstrong B, Mackey ER, Streisand R. Parenting behavior, child functioning, and health behaviors in preadolescents with type 1 diabetes. J Pediatr Psychol. 2011 Oct;36(9):1052-61. PubMed PMID: 21828111. Pubmed Central PMCID: PMC3173745.
- Ciechanowski PS, Hirsch IB, Katon WJ. Interpersonal predictors of HbA(1c) in patients with type 1 diabetes. Diabetes Care. 2002 Apr;25(4):731-6. PubMed PMID: 11919133.
- Bowlby J. Attachment and Loss: Separation: anxiety and anger. 1973;2.
- Fonagy P, Moran GS, Lindsay MK, Kurtz AB, Brown R. Psychological adjustment and diabetic control. Arch Dis Child. 1987 Oct;62(10):1009-13. PubMed PMID: 3499865. Pubmed Central PMCID: PMC1778653.
- Hood KK, Rausch JR, Dolan LM. Depressive symptoms predict change in glycemic control in adolescents with type 1 diabetes: rates, magnitude, and moderators of change. Pediatr Diabetes. 2011 Dec;12(8):718-23. PubMed PMID: 21564454.
- Law GU, Walsh J, Queralt V, Nouwen A. Adolescent and parent diabetes distress in type 1 diabetes: the role of self-efficacy, perceived consequences, family responsibility and adolescent-parent discrepancies. J Psychosom Res. 2013 Apr;74(4):334-9. PubMed PMID: 23497836.
- Maas-van Schaaijk NM, Roeleveld-Versteegh AB, van Baar AL. The interrelationships among paternal and maternal parenting stress, metabolic control, and depressive symptoms in adolescents with type 1 diabetes mellitus. J Pediatr Psychol. 2013 Jan-Feb;38(1):30-40. PubMed PMID: 22988060.
- Colton PA, Olmsted MP, Daneman D, Rodin GM. Depression, disturbed eating behavior, and metabolic control in teenage girls with type 1 diabetes. Pediatr Diabetes. 2013 Aug;14(5):372-6. PubMed PMID: 23418901. Epub 2013/02/20. eng.
- Powers MA, Richter S, Ackard D, Gerken S, Meier M, Criego A. Characteristics of persons with an eating disorder and type 1 diabetes and psychological comparisons with persons with an eating disorder and no diabetes. Int J Eat Disord. 2012 Mar;45(2):252-6. PubMed PMID: 21495056.
- Johnson B, Eiser C, Young V, Brierley S, Heller S. Prevalence of depression among young people with Type 1 diabetes: a systematic review. Diabet Med. 2013 Feb;30(2):199-208. PubMed PMID: 22698387. Epub 2012/06/16. eng.
- Strandberg RB, Graue M, Wentzel-Larsen T, Peyrot M, Rokne B. Relationships of diabetes-specific emotional distress, depression, anxiety, and overall well-being with HbA1c in adult persons with type 1 diabetes. J Psychosom Res. 2014 Sep;77(3):174-9. PubMed PMID: 25149027. Epub 2014/08/26. eng.
- Trief PM, Xing D, Foster NC, Maahs DM, Kittelsrud JM, Olson BA, et al. Depression in Adults in the T1D Exchange Clinic Registry. Diabetes Care. 2014 Jun;37(6):1563-72. PubMed PMID: 24855157. Epub 2014/05/24. eng.
- Ismail K, Moulton CD, Winkley K, Pickup JC, Thomas SM, Sherwood RA, et al. The association of depressive symptoms and diabetes distress with glycaemic control and diabetes complications over 2 years in newly diagnosed type 2 diabetes: a prospective cohort study. Diabetologia. 2017 Aug 03. PubMed PMID: 28776084.
- Nouwen A, Winkley K, Twisk J, Lloyd CE, Peyrot M, Ismail K, et al. Type 2 diabetes mellitus as a risk factor for the onset of depression: a systematic review and meta-analysis. Diabetologia. 2010 Dec;53(12):2480-6. PubMed PMID: 20711716. Pubmed Central PMCID: 2974923. Epub 2010/08/17. eng.
- Kan C, Silva N, Golden SH, Rajala U, Timonen M, Stahl D, et al. A systematic review and meta-analysis of the association between depression and insulin resistance. Diabetes Care. 2013 Feb;36(2):480-9. PubMed PMID: 23349152. Pubmed Central PMCID: PMC3554272.
- Khambaty T, Stewart JC, Muldoon MF, Kamarck TW. Depressive symptom clusters as predictors of 6-year increases in insulin resistance: data from the Pittsburgh Healthy Heart Project. Psychosom Med. 2014 Jun;76(5):363-9. PubMed PMID: 24846000. Pubmed Central PMCID: PMC4065635.
- Wang X, Bao W, Liu J, Ouyang YY, Wang D, Rong S, et al. Inflammatory markers and risk of type 2 diabetes: a systematic review and meta-analysis. Diabetes Care. 2013 Jan;36(1):166-75. PubMed PMID: 23264288. Pubmed Central PMCID: PMC3526249.
- Larsen CM, Faulenbach M, Vaag A, Volund A, Ehses JA, Seifert B, et al. Interleukin-1-receptor antagonist in type 2 diabetes mellitus. N Engl J Med. 2007 Apr 12;356(15):1517-26. PubMed PMID: 17429083.
- Goldfine AB, Buck JS, Desouza C, Fonseca V, Chen YD, Shoelson SE, et al. Targeting inflammation using salsalate in patients with type 2 diabetes: effects on flow-mediated dilation (TINSAL-FMD). Diabetes Care. 2013 Dec;36(12):4132-9. PubMed PMID: 24130358. Pubmed Central PMCID: PMC3836144.
- Dantzer R, O'Connor JC, Freund GG, Johnson RW, Kelley KW. From inflammation to sickness and depression: when the immune system subjugates the brain. Nat Rev Neurosci. 2008 Jan;9(1):46-56. PubMed PMID: 18073775. Pubmed Central PMCID: PMC2919277.
- Hoyo-Becerra C, Schlaak JF, Hermann DM. Insights from interferon-alpha-related depression for the pathogenesis of depression associated with inflammation. Brain Behav Immun. 2014 Nov;42:222-31. PubMed PMID: 25066466.
- Dowlati Y, Herrmann N, Swardfager W, Liu H, Sham L, Reim EK, et al. A meta-analysis of cytokines in major depression. Biol Psychiatry. 2010 Mar 01;67(5):446-57. PubMed PMID: 20015486.
- Na KS, Lee KJ, Lee JS, Cho YS, Jung HY. Efficacy of adjunctive celecoxib treatment for patients with major depressive disorder: a meta-analysis. Prog Neuropsychopharmacol Biol Psychiatry. 2014 Jan 03;48:79-85. PubMed PMID: 24056287.
- Laake JP, Stahl D, Amiel SA, Petrak F, Sherwood RA, Pickup JC, et al. The association between depressive symptoms and systemic inflammation in people with type 2 diabetes: findings from the South London Diabetes Study. Diabetes Care. 2014 Aug;37(8):2186-92. PubMed PMID: 24842983.
- Pradhan AD, Manson JE, Rifai N, Buring JE, Ridker PM. C-reactive protein, interleukin 6, and risk of developing type 2 diabetes mellitus. Jama. 2001 Jul 18;286(3):327-34. PubMed PMID: 11466099.
- Khandaker GM, Pearson RM, Zammit S, Lewis G, Jones PB. Association of serum interleukin 6 and C-reactive protein in childhood with depression and psychosis in young adult life: a population-based longitudinal study. JAMA Psychiatry. 2014 Oct;71(10):1121-8. PubMed PMID: 25133871. Pubmed Central PMCID: PMC4561502.
- Hayashino Y, Mashitani T, Tsujii S, Ishii H, Diabetes D, Care Registry at Tenri Study G. Elevated levels of hs-CRP are associated with high prevalence of depression in japanese patients with type 2 diabetes: the Diabetes Distress and Care Registry at Tenri (DDCRT 6). Diabetes Care. 2014 Sep;37(9):2459-65. PubMed PMID: 24969579.
- Sullivan MD, Katon WJ, Lovato LC, Miller ME, Murray AM, Horowitz KR, et al. Association of depression with accelerated cognitive decline among patients with type 2 diabetes in the ACCORD-MIND trial. JAMA Psychiatry. 2013 Oct;70(10):1041-7. PubMed PMID: 23945905. Pubmed Central PMCID: PMC4212406.
- Marioni RE, Strachan MW, Reynolds RM, Lowe GD, Mitchell RJ, Fowkes FG, et al. Association between raised inflammatory markers and cognitive decline in elderly people with type 2 diabetes: the Edinburgh Type 2 Diabetes Study. Diabetes. 2010 Mar;59(3):710-3. PubMed PMID: 19959761. Pubmed Central PMCID: PMC2828661.
- Ouellet-Morin I, Odgers CL, Danese A, Bowes L, Shakoor S, Papadopoulos AS, et al. Blunted cortisol responses to stress signal social and behavioral problems among maltreated/bullied 12-year-old children. Biol Psychiatry. 2011 Dec 01;70(11):1016-23. PubMed PMID: 21839988. Pubmed Central PMCID: PMC3816750.
- Danese A, Pariante CM, Caspi A, Taylor A, Poulton R. Childhood maltreatment predicts adult inflammation in a life-course study. Proc Natl Acad Sci U S A. 2007 Jan 23;104(4):1319-24. PubMed PMID: 17229839. Pubmed Central PMCID: PMC1783123.
- Kyrou I, Tsigos C. Stress hormones: physiological stress and regulation of metabolism. Curr Opin Pharmacol. 2009 Dec;9(6):787-93. PubMed PMID: 19758844.
- Herbert J, Goodyer IM, Grossman AB, Hastings MH, de Kloet ER, Lightman SL, et al. Do corticosteroids damage the brain? J Neuroendocrinol. 2006 Jun;18(6):393-411. PubMed PMID: 16684130.
- Frodl T, Carballedo A, Hughes MM, Saleh K, Fagan A, Skokauskas N, et al. Reduced expression of glucocorticoid-inducible genes GILZ and SGK-1: high IL-6 levels are associated with reduced hippocampal volumes in major depressive disorder. Transl Psychiatry. 2012 Mar 13;2:e88. PubMed PMID: 22832853. Pubmed Central PMCID: PMC3309536.
- Moulton CD, Costafreda SG, Horton P, Ismail K, Fu CH. Meta-analyses of structural regional cerebral effects in type 1 and type 2 diabetes. Brain Imaging Behav. 2015 Dec;9(4):651-62. PubMed PMID: 25563229.
- Stetler C, Miller GE. Depression and hypothalamic-pituitary-adrenal activation: a quantitative summary of four decades of research. Psychosom Med. 2011 Feb-Mar;73(2):114-26. PubMed PMID: 21257974.
- Scharnholz B, Gilles M, Marzina A, Kommer M, Lederbogen F, Wudy SA, et al. Do depressed patients without activation of the hypothalamus-pituitary-adrenal (HPA) system have metabolic disturbances? Psychoneuroendocrinology. 2014 Jan;39:104-10. PubMed PMID: 24275009.
- Teicher MH, Andersen SL, Polcari A, Anderson CM, Navalta CP, Kim DM. The neurobiological consequences of early stress and childhood maltreatment. Neurosci Biobehav Rev. 2003 Jan-Mar;27(1-2):33-44. PubMed PMID: 12732221.
- Wu TC, Chen HT, Chang HY, Yang CY, Hsiao MC, Cheng ML, et al. Mineralocorticoid receptor antagonist spironolactone prevents chronic corticosterone induced depression-like behavior. Psychoneuroendocrinology. 2013 Jun;38(6):871-83. PubMed PMID: 23044404.
- Germain A, Kupfer DJ. Circadian rhythm disturbances in depression. Hum Psychopharmacol. 2008 Oct;23(7):571-85. PubMed PMID: 18680211. Pubmed Central PMCID: PMC2612129.
- Prather AA, Vogelzangs N, Penninx BW. Sleep duration, insomnia, and markers of systemic inflammation: results from the Netherlands Study of Depression and Anxiety (NESDA). J Psychiatr Res. 2015 Jan;60:95-102. PubMed PMID: 25307717. Pubmed Central PMCID: PMC4250403.
- Hall MH, Muldoon MF, Jennings JR, Buysse DJ, Flory JD, Manuck SB. Self-reported sleep duration is associated with the metabolic syndrome in midlife adults. Sleep. 2008 May;31(5):635-43. PubMed PMID: 18517034. Pubmed Central PMCID: PMC2398755.
- Kudlow PA, Cha DS, Lam RW, McIntyre RS. Sleep architecture variation: a mediator of metabolic disturbance in individuals with major depressive disorder. Sleep Med. 2013 Oct;14(10):943-9. PubMed PMID: 24001995.
- Modell S, Ising M, Holsboer F, Lauer CJ. The Munich vulnerability study on affective disorders: premorbid polysomnographic profile of affected high-risk probands. Biol Psychiatry. 2005 Nov 01;58(9):694-9. PubMed PMID: 16018976.
- Karthikeyan R, Marimuthu G, Spence DW, Pandi-Perumal SR, BaHammam AS, Brown GM, et al. Should we listen to our clock to prevent type 2 diabetes mellitus? Diabetes Res Clin Pract. 2014 Nov;106(2):182-90. PubMed PMID: 25172521.
- Stamenkovic JA, Olsson AH, Nagorny CL, Malmgren S, Dekker-Nitert M, Ling C, et al. Regulation of core clock genes in human islets. Metabolism. 2012 Jul;61(7):978-85. PubMed PMID: 22304835.
- Bunney BG, Li JZ, Walsh DM, Stein R, Vawter MP, Cartagena P, et al. Circadian dysregulation of clock genes: clues to rapid treatments in major depressive disorder. Mol Psychiatry. 2015 Feb;20(1):48-55. PubMed PMID: 25349171. Pubmed Central PMCID: PMC4765913.
- Khunti K, Wolden ML, Thorsted BL, Andersen M, Davies MJ. Clinical inertia in people with type 2 diabetes: a retrospective cohort study of more than 80,000 people. Diabetes Care. 2013 Nov;36(11):3411-7. PubMed PMID: 23877982. Pubmed Central PMCID: PMC3816889.
- Winkley K, Stahl D, Chamley M, Stopford R, Boughdady M, Thomas S, et al. Low attendance at structured education for people with newly diagnosed type 2 diabetes: General practice characteristics and individual patient factors predict uptake. Patient Educ Couns. 2016 Jan;99(1):101-7. PubMed PMID: 26319362.
- Polonsky WH, Fisher L, Earles J, Dudl RJ, Lees J, Mullan J, et al. Assessing psychosocial distress in diabetes: development of the diabetes distress scale. Diabetes Care. 2005 Mar;28(3):626-31. PubMed PMID: 15735199. Epub 2005/03/01. eng.
- Stoop CH, Nefs G, Pop VJ, Wijnands-van Gent CJ, Tack CJ, Geelhoed-Duijvestijn PH, et al. Diabetes-specific emotional distress in people with Type 2 diabetes: a comparison between primary and secondary care. Diabet Med. 2014 Oct;31(10):1252-9. PubMed PMID: 24766062.
- Snoek FJ, Kersch NY, Eldrup E, Harman-Boehm I, Hermanns N, Kokoszka A, et al. Monitoring of Individual Needs in Diabetes (MIND): baseline data from the Cross-National Diabetes Attitudes, Wishes, and Needs (DAWN) MIND study. Diabetes Care. 2011 Mar;34(3):601-3. PubMed PMID: 21266654. Pubmed Central PMCID: 3041189. Epub 2011/01/27. eng.
- Carper MM, Traeger L, Gonzalez JS, Wexler DJ, Psaros C, Safren SA. The differential associations of depression and diabetes distress with quality of life domains in type 2 diabetes. J Behav Med. 2014 Jun;37(3):501-10. PubMed PMID: 23515932. Pubmed Central PMCID: 3758402. Epub 2013/03/22. eng.
- Fisher L, Skaff MM, Mullan JT, Arean P, Mohr D, Masharani U, et al. Clinical Depression Versus Distress Among Patients With Type 2 Diabetes: Not just a question of semantics. Diabetes Care. 2007 March 1, 2007;30(3):542-8.
- Snoek FJ, Kersch NY, Eldrup E, Harman-Boehm I, Hermanns N, Kokoszka A, et al. Monitoring of Individual Needs in Diabetes (MIND)-2: Follow-up data from the Cross-National Diabetes Attitudes, Wishes, and Needs (DAWN) MIND Study. Diabetes Care. 2012 Jul 26. PubMed PMID: 22837364. Epub 2012/07/28. Eng.
- Fisher L, Mullan JT, Arean P, Glasgow RE, Hessler D, Masharani U. Diabetes distress but not clinical depression or depressive symptoms is associated with glycemic control in both cross-sectional and longitudinal analyses. Diabetes Care. 2010 Jan;33(1):23-8. PubMed PMID: 19837786. Pubmed Central PMCID: PMC2797978.
- Herpertz S, Albus C, Lichtblau K, Kohle K, Mann K, Senf W. Relationship of weight and eating disorders in type 2 diabetic patients: a multicenter study. Int J Eat Disord. 2000 Jul;28(1):68-77. PubMed PMID: 10800015.
- Kenardy J, Mensch M, Bowen K, Pearson SA. A comparison of eating behaviors in newly diagnosed NIDDM patients and case-matched control subjects. Diabetes Care. 1994 Oct;17(10):1197-9. PubMed PMID: 7821143. Epub 1994/10/01. eng.
- Cuijpers P, van Straten A, Smit F, Mihalopoulos C, Beekman A. Preventing the onset of depressive disorders: a meta-analytic review of psychological interventions. Am J Psychiatry. 2008 Oct;165(10):1272-80. PubMed PMID: 18765483. Epub 2008/09/04. eng.
- Kampling H, Baumeister H, Jäckel WH, Mittag O. Prevention of depression in chronically physically ill adults (protocol). Cochrane Database of Systematic Reviews. 2014 (8):Art. No.: CD011246.
- Pols AD, van Dijk SE, Bosmans JE, Hoekstra T, van Marwijk HWJ, van Tulder MW, et al. Effectiveness of a stepped-care intervention to prevent major depression in patients with type 2 diabetes mellitus and/or coronary heart disease and subthreshold depression: A pragmatic cluster randomized controlled trial. PLoS One. 2017;12(8):e0181023. PubMed PMID: 28763451. Pubmed Central PMCID: PMC5538642.
- Petrak F, Hautzinger M, Müller M, Kronfeld K, Ruckes C, Herpertz S. Cognitive Behavioral Therapy in elderly Type 2 Diabetes Patients with minor Depression or mild Major Depression: a randomized controlled Trial. Presented at the 11th IDF-WPR Congress 2016 & 8th AASD Scientific Meeting, 27th to 30th October 2016. Taipeh, Taiwan.
- Kulzer B, Albus C, Herpertz S, Kruse J, Lange K, Lederbogen F, et al. [Pychosocial Aspects of Diabetes Mellitus (Part 1) S2-Guideline Psychosocial Aspects of Diabetes – Long Version]
S2-Leitlinie Psychosoziales und Diabetes - Langfassung. Diabetologie. 2013;8(198-242).
- Parchman ML, Zeber JE, Palmer RF. Participatory decision making, patient activation, medication adherence, and intermediate clinical outcomes in type 2 diabetes: a STARNet study. Ann Fam Med. 2010 Sep-Oct;8(5):410-7. PubMed PMID: 20843882. Pubmed Central PMCID: PMC2939416.
- Peyrot M, Rubin RR. Behavioral and psychosocial interventions in diabetes: a conceptual review. Diabetes Care. 2007 Oct;30(10):2433-40. PubMed PMID: 17666457.
- Baumeister H, Hutter N. Collaborative care for depression in medically ill patients. Curr Opin Psychiatry. 2012;25(5):405–14. eng.
- Pal K, Eastwood SV, Michie S, Farmer A, Barnard ML, Peacock R, et al. Computer-based interventions to improve self-management in adults with type 2 diabetes: a systematic review and meta-analysis. Diabetes Care. 2014 Jun;37(6):1759-66. PubMed PMID: 24855158.
- APA. Dissemination of integrated care within adult primary care settings. The Collaborative Care Model. 2016.
- Lustman PJ, Griffith LS, Freedland KE, Kissel SS, Clouse RE. Cognitive Behavior Therapy for Depression in Type 2 Diabetes Mellitus - A Randomized, Controlled Trial. Annals of Internal Medicine. 1998;129(8):613–21.
- van der Feltz-Cornelis CM, Nuyen J, Stoop C, Chan J, Jacobson AM, Katon W, et al. Effect of interventions for major depressive disorder and significant depressive symptoms in patients with diabetes mellitus: a systematic review and meta-analysis. Gen Hosp Psychiatry. 2010 Jul-Aug;32(4):380-95. PubMed PMID: 20633742. Epub 2010/07/17. eng.
- Baumeister H, Hutter N, Bengel J. Psychological and pharmacological interventions for depression in patients with diabetes mellitus and depression. Cochrane Database Syst Rev. 2012 Dec 12;12:CD008381. PubMed PMID: 23235661.
- Li C, Xu D, Hu M, Tan Y, Zhang P, Li G, et al. A systematic review and meta-analysis of randomized controlled trials of cognitive behavior therapy for patients with diabetes and depression. J Psychosom Res. 2017 Apr;95:44-54. PubMed PMID: 28314548.
- Xie J, Deng W. Psychosocial intervention for patients with type 2 diabetes mellitus and comorbid depression: a meta-analysis of randomized controlled trials. Neuropsychiatr Dis Treat. 2017;13:2681-90. PubMed PMID: 29123401. Pubmed Central PMCID: PMC5661466.
- Atlantis E, Fahey P, Foster J. Collaborative care for comorbid depression and diabetes: a systematic review and meta-analysis. BMJ Open. 2014;4(4):e004706.
- Uchendu C, Blake H. Effectiveness of cognitive-behavioural therapy on glycaemic control and psychological outcomes in adults with diabetes mellitus: a systematic review and meta-analysis of randomized controlled trials. Diabet Med. 2016 Jul 29. PubMed PMID: 27472405.
- Lakens D. Calculating and reporting effect sizes to facilitate cumulative science: a practical primer for t-tests and ANOVAs. Front Psychol. 2013 Nov 26;4:863. PubMed PMID: 24324449. Pubmed Central PMCID: PMC3840331.
- Cohen J. Statistical power analysis for the bahavioral sciences. Hillsdale: Lawrence Erlbaum; 1988.
- Cuijpers P, Berking M, Andersson G, Quigley L, Kleiboer A, Dobson KS. A meta-analysis of cognitive-behavioural therapy for adult depression, alone and in comparison with other treatments. Can J Psychiatry. 2013 Jul;58(7):376-85. PubMed PMID: 23870719.
- Petrak F, Herpertz S, Albus C, Hermanns N, Hiemke C, Hiller W, et al. Cognitive Behavioral Therapy Versus Sertraline in Patients With Depression and Poorly Controlled Diabetes: The Diabetes and Depression (DAD) Study: A Randomized Controlled Multicenter Trial. Diabetes Care. 2015 May;38(5):767-75. PubMed PMID: 25690005.
- Petrak F, Herpertz S, Albus C, Hirsch A, Kulzer B, Kruse J. Psychosocial factors and diabetes mellitus: evidence-based treatment guidelines. Curr Diabetes Rev. 2005 Aug;1(3):255-70. PubMed PMID: 18220602.
- NICE guidelines [CG91] Depression in adults with a chronic physical health problem: Treatment and management [Internet]. 2009. Available from: http://www.nice.org.uk/guidance/cg91.
- Standards of Medical Care in Diabetes-2017: Summary of Revisions. Diabetes Care. 2017 Jan;40(Suppl 1):S4-S5. PubMed PMID: 27979887.
- Chamberlain JJ, Kalyani RR, Leal S, Rhinehart AS, Shubrook JH, Skolnik N, et al. Treatment of Type 1 Diabetes: Synopsis of the 2017 American Diabetes Association Standards of Medical Care in Diabetes. Ann Intern Med. 2017 Oct 3;167(7):493-8. PubMed PMID: 28892816.
- Bundesärztekammer°(BÄK) KBK, °Arbeitsgemeinschaft°der°Wissenschaftlichen°Medizinischen°Fachgesellschaften°(AWMF). Nationale VersorgungsLeitlinie Therapie des Typ-2-Diabetes – Langfassung, 1. Auflage. Version 4. 2013, zuletzt geändert: November 2014 2014. Available from: Available from: www.dm-therapie.versorgungsleitlinien.de.
- DGPPN B, °KBV,°AWMF°(Hrsg.)°für°die°Leitliniengruppe°Unipolare°Depression. S3-Leitlinie/Nationale VersorgungsLeitlinie Unipolare Depression – Langfassung, 2. Auflage. Version 5. 2015 [cited: 2017-12-18] www.depression.versorgungsleitlinien.de. . 2015.