ABSTRACT
Sport is the organized playing of competitive games according to rules. Hence doping represent drug cheating, a fraud on competitors, the sport, and the public. The charter of the World Anti-doping Agency (WADA) forms a harmonized Code that authorizes an annually updated list of prohibited doping substances and methods as well as accrediting national anti-doping labs around the world. Sports performance has 4 major components: skill, strength, endurance, and recovery, with each sport employing a distinct combination of these elements. These performance characteristics also correspond to the most potent and effective forms of doping. Sports requiring explosive power are most susceptible to androgen doping through their effect on increasing muscle mass and strength whereas sports that require endurance are most enhanced by hemoglobin (blood) doping which increases oxygen delivering capacity to exercising tissues. Performance in contact sports and those involving intense physical activity or training may also be enhanced by growth hormone and its secretagogues through speeding of tissue recovery from injury. Hormones remain the most potent and widely detected doping agents being responsible for about 2/3 of anti-doping rule violations detected by increasingly sophisticated detection methods. At present, the vast majority of positives are still due to a wide variety of androgens, including marketed and illicit nutraceutical, designer, specific androgen receptor modulator (SARM)) synthetic androgens as well as exogenous natural androgens, while the peptide hormones (erythropoiesis stimulating agents, growth hormone and its secretagogues) and autologous blood transfusion remain difficult to detect.
INTRODUCTION
Across the world, sport is a ubiquitous human social activity that forms an unique intersection of health, recreation, entertainment, and industry (1). It is both a major economic activity as well as a profound influence on social behavior of individuals at home, work, and play. One practical and concise definition of sport is the organized playing of competitive games according to rules. In that context, rule breaking is cheating to achieve an unfair competitive advantage whether it involves using illegal equipment, match fixing, banned drugs, or any other prohibited means.
The illicit use of banned drugs (doping) to influence the outcome of a sporting contest, constitutes a fraud against competitors, spectators, sport, sponsors, and the public no different from other personal, professional, or commercial frauds. While performance enhancement is almost invariably the intent of cheating, impairing performance is also well known in horse racing and even, rarely, in human elite sports (e.g. drink-spiking of banned drugs, injurious physical assaults). Rules of sporting contest may change by agreement, but once set, represents the boundaries of fair competition. Nevertheless, fairness is an elastic, socially constructed concept which may change gradually over time. For example, a century ago deliberate training itself was considered an ungentlemanly breach of fairness as competition was then envisaged as a contest based solely on natural endowments. Similarly, some sports once maintained a distinction between amateurs and professionals. The philosophical foundations of the concept of fairness is a deep and complex issue (2,3) where the focus has been mainly on distributive justice with an implicit goal of equality of outcomes. Less attention has been given to the philosophical basis of fair competition in sport where the prior distribution of talent and training and the outcome of contest are intended to provide equality of opportunity, but not of outcome, between contestants.
Naïve arguments are made that deny doping is cheating, or unsafe or violates the spirit of sport and asserting that drugs should be freely available or under medical supervision (4-6). However, removing prohibition on doping would immediately render drug taking as pervasive as training in elite sport extending to promising underage and sub-elite athletes. Ensuing demands on doctors to prescribe excessive, often massive, drug doses without medical indications would be unprofessional, unethical, and unsafe. This could convert sporting participation into a potentially dangerous rather than a healthful activity. In practice, creating enforceable boundaries for drugs in sport is unavoidable whether it is prohibition or, even under the most idealistic libertarian scenarios, by age or dosage. Within the limitations of unverifiable self-report regarding an illicit activity (7,8), surveys indicate athletes support antidoping testing mainly to prevent cheating but also to promote safety (9-11). Motivating factors for, and routes by which athletes get involved in doping are complex but include the use of non-banned nutritional supplements as a gateway to doping (12) and the suspicion of athletes or their entourage that their rivals may be using illicit drugs, the so-called “false consensus belief” (7,13-15). These philosophical issues are not considered further here and, recognizing that sport requires concrete, practical decisions, the establishment and enforcement of agreed rules is the basis of fair competition. An excellent discussion of the logic and morality of a decisive antidoping approach from an ethicist with extensive experience in sports anti-doping is recommended (16).
It is well understood that individual human genetic endowments are unequal and, among these, sporting prowess is at least partly genetically determined (17). However, little is still known of the genotype-phenotype correlations that underlie beneficial genetic endowments for sports performance. Natural genetic advantages are recognized in height (tallness for basketball, shortness for jockeys and motor-cycle riders) and hereditary erythrocytosis where a high circulating hemoglobin due to a high affinity erythropoietin (EPO) receptor (18)) for endurance sports, or conversely genetic disadvantage such as the common α-actinin-3 deletion genetic polymorphism which limits anaerobic, explosive power (19). More examples of genetic (dis)advantages for sports performance are likely to be identified as genomics continues to expand our understanding of the biological basis of health, including natural human sporting prowess. In the context of sports doping, however, a person’s genetic endowment is a given creating a natural boundary whereby the use of exogenous drugs or chemicals (including DNA) may constitute drug cheating or doping.
WORLD ANTI-DOPING AGENCY (WADA) AND THE GLOBAL ANTI-DOPING REGULATORY ENVIRONMENT
Cheating is as old as sport itself, yet the present endemic of doping using pharmaceutical drugs to boost sports performance is largely a Cold War legacy. Eastern European national doping programs were established by governments aiming to achieve a short-cut propaganda victory over their Western rivals, a challenge quickly reciprocated and then taken up by individual coaches and athletes. Starting with power sports (20), the epidemic became entrenched as an endemic in sufficiently affluent circles. In 1967, following the introduction of anti-doping rules by some sports federations, the International Olympic Committee (IOC) established its Medical Commission, which published their first list of prohibited substances. During the 1970’s the IOC Medical Commission took an increasingly active role by banning androgens which required developing standardized, valid methods to detect and deter androgen doping. After discarding alternatives such as immunoassays and blood sampling, in the 1980s mass spectrometry (MS)-based tests became (21) and remain the standard for detecting synthetic androgens in urine.
In 1999, the IOC established the WADA based in Montreal to be equally supported by governments and sporting organizations with its charter, the WADA Code, representing a harmonized set of global anti-doping rules introduced in 2004, revised in 2009 and 2015 and will be again revised in 2021(22). WADA also publishes an annually updated Prohibited List of Substances and Methods, accredits national anti-doping labs together with their operational anti-doping testing framework, and established the Court for Arbitration in Sport (CAS) to settle anti-doping legal disputes as sport’s “Supreme Court”. The WADA Code has been adopted by over 660 sporting organizations including all Olympic and Paralympic organizations and National Anti-Doping Organizations as well as most non-Olympic International and National Sports Federations. The WADA Code prohibits substances or methods which meet 2 of 3 criteria comprising:
(i) enhance performance (cheating),
(ii) harmful to health (safety) or
(iii) violate the spirit of sport (unsporting).
Although the primacy of penalizing cheating is widely understood, implementing these criteria encounter ethical and practical difficulties in proving ergogenic effects of increasing numbers of illicit and/or non-approved substances. These substances have unknown safety so that human testing is not feasible making athlete safety an important consideration. Crucially, the Code imposes strict liability on individual athletes so that a positive anti-doping test (including refusal or avoidance of testing or possession, attempts, trading and tampering with banned drugs) constitutes an anti-doping rule violation (ADRV), regardless of intent or negligence. Sanctions involve suspension from any elite competitive sport and extend to support personnel and teams. Suspensions, once 2 years are now 4 years since adoption of the 2015 Code. This is generally believed to be longer than the ergogenic benefits of doping, although recent evidence suggests that androgen effects on muscle may create durable or even permanent effects (23) which might argue for much longer or permanent banning of androgen doping violators.
The Prohibited List bans, at any time either in or out of competition, the use of performance enhancing hormones, including androgens, EPO and growth hormone and related substances or drugs which stimulate endogenous production of these hormones (Table 1). Among the 15 categories of prohibited substances (12) and methods (3), hormones feature prominently in S1 (anabolic agents, mainly androgens), S2 (peptide hormones, growth factors, related substances and mimetics), S4 (hormone and metabolic modulators), and S9 (glucocorticoids) with S1 and S2 having important “catch-all” provision for unnamed but related substances “with similar chemical structure or biological effects”. In addition, the S0 category bans non-approved substances, those without current regulatory approval for human therapeutic use. The prominence of hormones is reinforced by the WADA laboratory statistics for anti-doping tests where hormones remain the most frequently detected banned drugs (Table 2). In 2017, of over 322,000 anti-doping tests ~1.5% were positive with 61% due to hormones, the vast majority (~99%) due to androgens. These findings confirm that the detection of androgen doping is effective whereas the low rate of detection of hemoglobin or growth hormone doping may reflect the limitations of available tests for peptides and peptide hormones which require blood rather than conventional urine sampling and feature low sensitivity and brief windows of detection, rather than their lack of abuse. Further use of out-of-competition testing and blood samples together with more sensitive detection tests with longer windows of detection are required particularly for peptide hormones.
Table 2. Performance Enhancing Hormone Tests in WADA Labs |
||||
|
2005 |
2009 |
2013 |
2017 |
ACCREDITED LABS |
33 |
35 |
33 |
31 |
TOTAL TESTS |
183,337 |
277,928 |
269,878 |
322,050 |
POSITIVES (% OF TOTAL) |
3,909 (2.1%) |
5,610 (2.0%) |
5962 (2.2%) |
4756 (1.5%) |
HORMONES (% OF +VE) |
55% |
73% |
57% |
61% |
ANDROGENS |
3893 |
5541 |
3352 |
1813 |
BLOOD/EPO |
16 |
68 |
63 |
85 |
GH/PEPTIDES |
0 |
1 |
0 |
19 |
Source: WADA website report on laboratory testing figures. See http://www.wada-ama.org/en/Science-Medicine/Anti-Doping-Laboratories/Laboratory-Testing-Figures/
The prevalence of doping in elite sports as an illicit activity with drastic consequences for athletes admitting guilt remains difficult to quantify using laboratory-based testing, inference from performance, or self-report questionnaires (24). The most promising methods appear to be questionnaires using the unrelated question random response methodology (25). This methodology was developed to estimate the prevalence of sensitive, disapproved, or illicit activities by asking the sensitive personal questions masked by mixing them with unrelated non-sensitive questions in an anonymized framework. This provides overall prevalence self-report estimates of the target activities without allowing for individual identification of answers. One study of two elite athletic competitions provided estimates of 43.6% and 57.1% for recent (last year) and 70.1% of ever use of banned doping methods (26). However, another study using the same methodology found markedly lower prevalence estimates of 0.7% to 11.9% for recent use of banned doping (27). The discrepancies between these prevalence estimates requires further clarification. A significant limitation of these methods is their reliance on athlete’s perception of banned methods. For example, whether “banned drugs” are interpreted as including the widely used (but non-banned) nutritional supplements which athletes are urged to avoid for fear of adulteration with unlabeled banned substances.
Therapeutic Use Exemption (TUE)
In rare cases, an elite athlete with a genuine medical need for therapeutic use of a prohibited drug may be granted a TUE (28). This exempts the athlete from the Code’s strict liability provision and permits them to compete during ongoing necessary medical treatment. WADA provides medical guidelines that standardize the evaluation and management of TUE applications for a range of medical illnesses. A TUE is granted by a national anti-doping organization based on an independent, expert review of valid, documented diagnosis, appropriate clinical indications and dose for hormonal treatment with a view to facilitating essential medical treatment but avoiding unjustified use or over-dosage. After stringent review TUE’s may be granted for treatment with testosterone, glucocorticoids, and insulin but there are very rarely any valid medical indications for EPO or, in adults, for growth hormone or IGF-1 in elite athletes. For example, TUE’s are usually justified for young male athletes with genuine androgen deficiency, occurring in ~1:200 men (29), due to organic pituitary-testicular disorders with an established pathological basis (e.g. bilateral orchidectomy, severe mumps orchitis, Klinefelter’s syndrome, etc.) who require life-long testosterone replacement therapy (30). The TUE will approve, subject to regular review, a standard testosterone replacement regimen, including dosage and monitoring, with changes to regimen requiring approval. TUEs are not granted for men with functional decreases in blood T due to non-reproductive disorders including stress (“over-training”) or ageing (“andropause”, “LowT” “late-onset hypogonadism”), or for women.
In principle, detection of prohibited substances is ideally aimed at identifying a xenobiotic substance or its distinctive chemical signature(s) which do not occur naturally in the body, thereby distinguishing it categorically from normal body constituents. Such identification of a non-natural substance that can’t be of endogenous origin is congruent with the strict liability onus in proving an anti-doping rule violation (ADRV). Proving an ADRV is more difficult to achieve with administration of natural hormones or their analogs which must be distinguished from their endogenous counterparts. In this situation, the alternative requires developing valid biomarkers to prove the use of banned substances through their distinctive effects on the body and tissues. It is a formidable challenge to validate an indirect biomarker as proof of an ADRV capable of withstanding vigorous medico-legal challenge when a proven ADRV would prevent an athlete from pursuing their profession. Proof of an ADRV based on a doping detection test requires rigorous standardization and harmonization of every stage of the anti-doping tests from sample collection, chain-of-custody, storage, and analysis including accounting for any fixed (genetic, gender, age, ethnicity) or variable (exercise, hydration, masking vulnerabilities) factors which may impact on proposed test metrics.
COMPONENTS OF SPORTS PERFORMANCE AND DOPING
Sports performance has 4 major dimensions – skill, strength, endurance and recovery (Figure 1). High performance in any sport requires a characteristic blend of these dimensions although individual sports differ widely in that balance. Similarly, the major ergogenic drug classes have distinctive effects aligned predominantly along one of these dimensions so that the most effective ergogenic drug classes used in doping are dictated by these dimensions of sports performance (Figure 2). While every sport requires an acquired skill, some are largely or solely based on skill and concentration (e.g. board games, target shooting, car driving, and motor-cycle riding) and may benefit from drugs that reduce anxiety, tremor, inattention or fatigue. Sports that are highly dependent on explosive, short-term anaerobic power (sprinting, throwing, boxing, wrestling), typically ones which favor a stocky, muscular build, are most susceptible to androgen-induced increases in muscle mass and strength. Other sports with an emphasis on aerobic effort and endurance (e.g. long distance or duration events), characteristically favored by a lean build, may be boosted by hemoglobin doping (blood transfusion, erythropoietin (EPO) and its analogs or mimetics. Finally, sports that depend on recovery from major injury or recurrent minor injury during intensive training, notably contact sports, may benefit from tissue proliferative and remodeling effects of growth hormone and various growth factors.
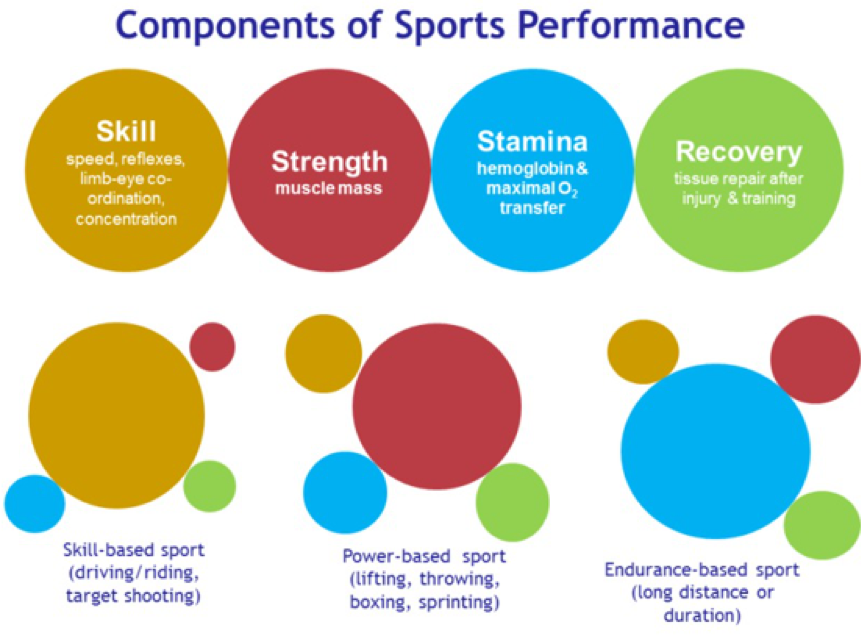
Figure 1. Components of Sports Performance
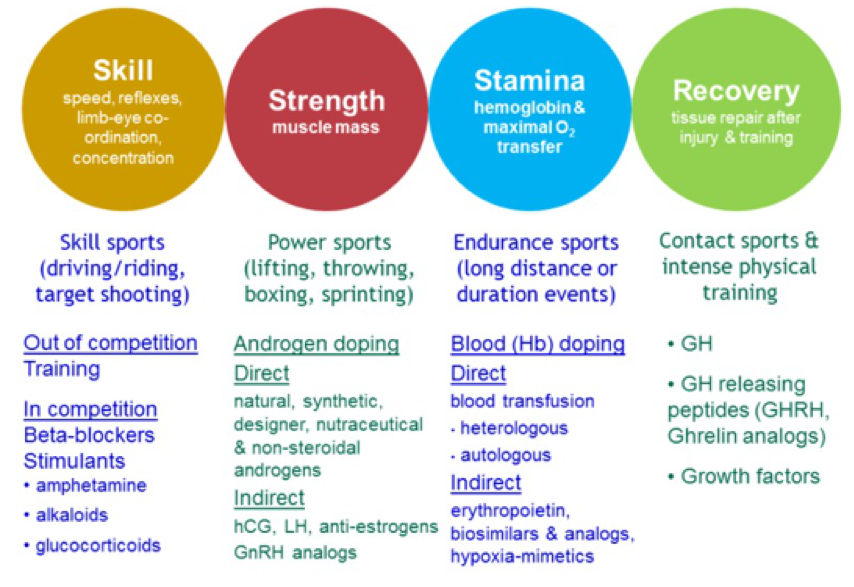
Figure 2. Drugs that Enhance Sports Performance
ANDROGENS
Although the ergogenic effects of androgens were discovered empirically soon after the identification of testosterone as the principal male androgen of testicular origin in 1935 (31), their applications to elite sport performance were mainly developed during the Cold War by trial and error experiments undertaken on unknowing elite athletes (20,32,33); however, the scientific basis of androgen doping was only objectively proven in the 1990’s. Until that time, the settled consensus was that exogenous androgens had no effect in eugonadal men whose androgen receptors were already saturated by endogenous testosterone (T) (20,34,35). The then alleged benefits of androgen doping were misattributed placebo responses together with training and nutritional effects. Using an exemplary placebo-controlled, randomized clinical trial design with a wide range of testosterone doses, Bhasin et al showed that T increased muscle mass and strength in eugonadal young men to a similar extent as exercise alone and with additive effects when combined with exercise (36) (figure 3). Subsequent dose-response studies showed that administration of T increased muscle mass and strength by 10% without and 20-37% with exercise (where exercise alone increased them by 10-20%) together with additive effects from 3% increase in circulating hemoglobin. These benefits extended from below to well above physiological T doses or blood levels without evidence of plateau (37,38) and regardless of age (39).
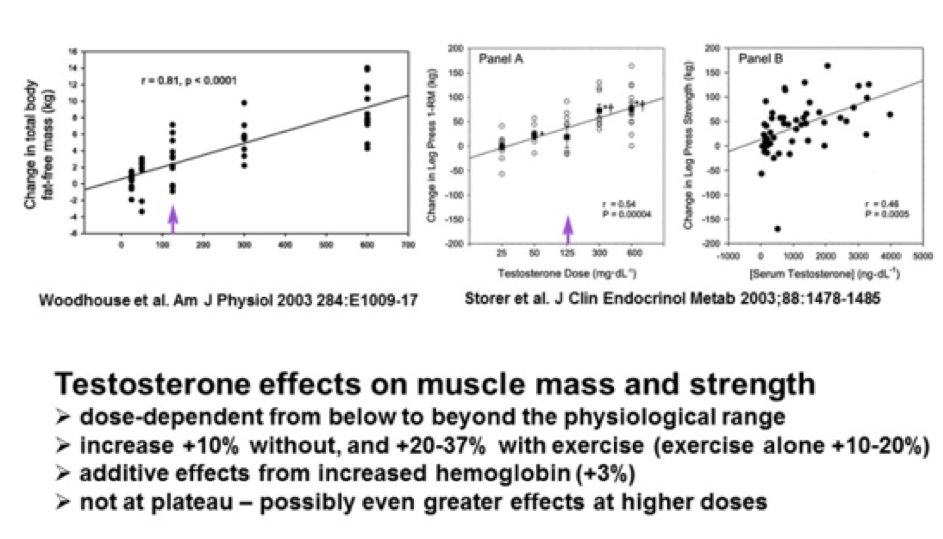
Figure 3. Biological Basis of Androgen Doping
Androgen doping may be either direct or indirect (Table 3, figure 4). Direct androgen doping involves administration of testosterone, natural or synthetic androgens whereas indirect androgen doping includes a variety of non-androgenic drugs which increase endogenous T. Direct androgen doping originally involved all pharmaceutically marketed natural (T, DHT, nandrolone) and synthetic androgens but has extended to non-marketed designer and nutraceutical androgens as well as pro-androgens (androstenedione, DHEA) and the new class of non-steroidal androgens (selective androgen receptor modulators, SARM (40)). Indirect androgen doping involves use of hCG, LH, anti-estrogens (estrogen receptor blockers, aromatase inhibitors), opioid antagonists, and neurotransmitters involved in neuroendocrine regulation of endogenous LH and T secretion (41-44).
Table 3– Direct and Indirect Androgen Doping and Detection Methods
|
|
Substance |
Detection method |
Direct |
|
Synthetic androgens |
L/GC-MS |
Natural androgens |
L/GC-MS, T/E, CIRMS |
Designer & nutraceutical androgens |
L/GC-MS (bioassay) |
Indirect |
|
hCG (urinary or recombinant) |
hCG immunoassay or LC-MS |
hLH (recombinant) |
hLH immunoassay or LC-MS |
Anti-estrogens |
L/GC-MS |
GnRH analogs |
L/C-MS |
Opioid antagonists & neurotransmitters |
L/C-MS |
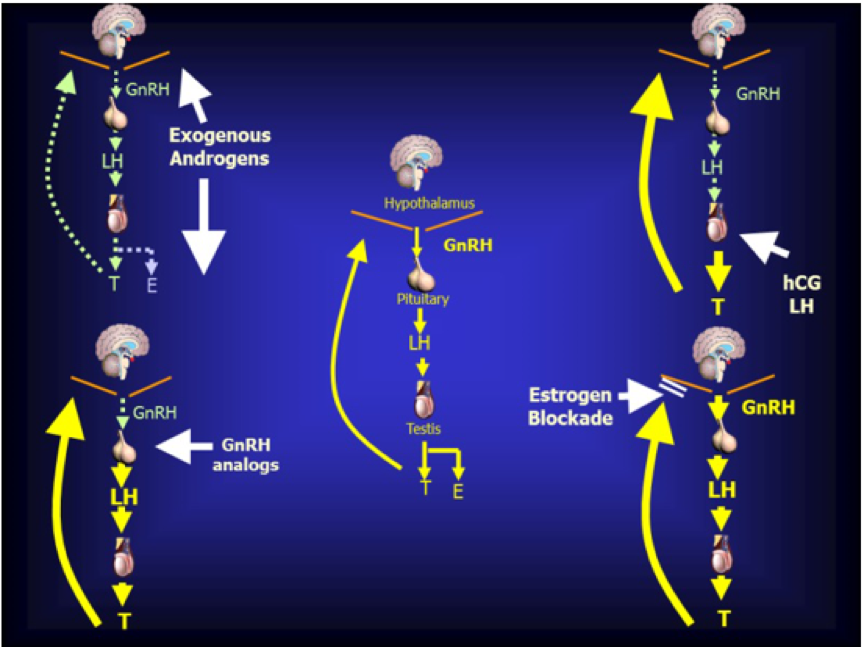
Figure 4. Direct and Indirect Androgen Doping
Detection of direct androgen doping using steroids of known chemical structure is highly effective using gas or liquid chromatography MS (45-47). Traces of synthetic androgens or their metabolites may remain detectable for periods up to months after last administration (48). Recent developments including the identification of long-term metabolites has further widened the detection windows for synthetic androgens (49-55). Challenges to detection of synthetic androgens have included the development of non-marketed designer and nutraceutical androgens, the use of natural androgens, and pro-androgens, masking methods, restricting use to out-of-competition training or micro-dosing. Designer and nutraceutical androgens are typically non-marketed synthetic androgens based on structures and synthesis methods recovered from expired patent literature of the 1960-70’s. These are now synthesized by unregulated chemical manufacturers without Good Manufacturing Practice (GMP) licensing advertising and sold over the internet or over-the-counter as nutritional supplements, which may contain undeclared steroids (56). However, once the chemical structures of any synthetic androgens are known, they are easily detectable although the sheer profusion of such chemicals represents an ongoing challenge. Nevertheless, despite their novelty, there is little evidence designer androgens have been used after they are discovered so that there is a high likelihood of detection. As a result, virtually all ongoing androgen ADRVs are still due to conventional marketed synthetic androgens.
Distinguishing Between the Exogenous and Endogenous Steroids
Administration of natural androgens (T or DHT) or pro-androgens (androstenedione, DHEA), raises the problem of distinguishing between the exogenous and endogenous steroids. Exogenous T administration can be detected by the urine T/E ratio, the ratio in urine of T to its 17α-epimer epitestosterone (E), operating as a sensitive screening test. In males, both T and E are co-secreted by Leydig cells and excreted in urine consistently so that the urine T/E is usually stable for any individual over time, being typically around 1. Administration of exogenous T, which is not converted to E, increases the urine T/E ratio and, when it exceeds a specified threshold, is evidence for administration of exogenous T. The urine T/E ratio thresholds were originally population-based, set initially at 6 and then subsequently lowered to 4. However, the urine T/E ratio is not an effective screening test for testosterone doping in females (57)because, unlike males whose circulating testosterone originates from as single source subject to strong negative hypothalamic feedback, circulating testosterone in females originates from three steroidogenic sources (adrenal, ovary, extraglandular conversion) none of which are subject to strong negative feedback by testosterone. Furthermore, the possibility of false negatives and false positives of population-based thresholds are limitations which may require further analysis to confirm or refute T doping in individual cases. These considerations have led to establishment of the steroid module of the Athletes Biological Passport (ABP), a compendium of serial observation of any individual’s tests which creates adaptive individual-specific T/E ratio threshold (58). This substitution of an individual’s own person-specific, in place of the population-based, thresholds allows for more sensitive and accurate detection of individual deviations in urine T/E ratio as evidence of T doping.
One limitation of the urine T/E ratio is a genetic polymorphism of the uridine 5'-diphospho-glucuronosyltransferase(UGT) 2B17 gene which encodes a phase II hepatic enzyme that glucuronidates T rendering it more hydrophilic to facilitate urinary excretion. This polymorphism comprises a genetic deletion which, in homozygotes, produces a non-functional enzyme that reduces urinary T (but not E) excretion to near zero producing an extremely low T/E ratio (<0.1). While this genetic polymorphism has no apparent biological effect on T action, it is unevenly distributed geographically being much more frequent in South East Asian populations (59). This biological false negative means that administration of exogenous T will be greatly reduced and may not exceed the usual population-based T/E ratio thresholds (60). On the other hand, it will exceed any individual’s own specific urine T/E ratio threshold so that genotyping and/or Bayesian profiling of serial T/E ratio in an ABP program provide complementary evidence (61-63).
Administration of exogenous T may also be identified by carbon isotope ratio MS (CIRMS) that can distinguish endogenous from exogenous T according to the C13/C12 ratio of urinary T (64,65). Commercially, steroids are manufactured from plant sterols produced by photosynthesis that exhibit distinctly lower C13/C12 ratio (typically, -26‰ to -36‰ relative to the global standard) compared with mammalian T biosynthesis (between -16‰ to -26‰) (66,67). Hence, a significantly lowered (“depleted”) C13/C12 ratio of urinary T, exceeding 3‰ relative to endogenous reference steroids, indicates that urinary T originates at least partly from exogenous chemical manufacture from plant sterols. CIRMS can also be applied to detect administration of other natural androgens or pro-androgens including DHT and DHEA (68), androstenedione, or even attempted masking by administering E (to lower urine T/E ratio) (66). A few T products (<5% (69)) have recently emerged with a lower, more mammalian-like C13/C12 ratio for urine T (70) creating a challenge for CIRMS detection. Nevertheless, extended isotope profiling of other steroid precursors and metabolites provides additional complementary reference biomarkers (71). A longitudinal application of CIRMS along the lines of another module of the ABP has been proposed (72). Furthermore, development of hydrogen ion ratio mass spectrometry has further enhanced the ability to distinguish between endogenous and exogenous steroids even when the carbon isotope ratio is non-informative (73-75). Suppression of urine (or serum) LH excretion may also provide corroborative evidence for the use of exogenous T or other synthetic androgens (63,76-78).
While MS is highly effective for detecting specific androgens, it requires knowledge of the chemical structure to be detected and otherwise cannot be applied. This principle applies to never-marketed designer or nutraceutical androgens sold over the internet or in unregulated over-the-counter nutritional supplements with unlabeled steroid content. A potential solution is the modern in vitro androgen bioassay that incorporates the human androgen receptor together with a convenient transactivation chemical read-out signal into a host yeast or mammalian cell (79). This has the generic capacity to detect all bioactive androgens regardless of structure due to their direct activation of the androgen receptor. Constructed in vitro androgen bioassays feature a sensitive dose-response signal proportional to the potency of the bioactive androgen (80-83). Yeast host cells have high specificity for detecting androgens but are less sensitive than mammalian cells, which express native steroid mechanisms including steroidogenic enzymes and/or other steroid receptors. Mammalian in vitro androgen bioassays can also detect pro-androgens, steroids lacking intrinsic androgenic bioactivity but which are converted into androgens within the mammalian cell. Hence, while mammalian host cells sacrifice specificity for higher sensitivity, they can also detect pro-androgens (79). Hence yeast and mammalian in vitro androgen bioassays are complementary in detecting both androgens and pro-androgens. The limitations of in vitro androgen bioassays are their susceptibility to matrix effects and difficulties in standardizing bioassay-based test. Consequently. they are best deployed to characterize products and substances for androgens or pro-androgen content rather than to detect androgens in complex biological samples. Hence the yeast androgen bioassay was decisive in the first conviction for use of a designer androgen by proving that tetrahydrogestrinone (THG) was a potent androgen (84) and has also been used to screen synthetic progestins to show that, unlike the original androgen-derived progestins, the modern generation of progestins are not androgenic (85).
Additional underutilized options to detect androgen doping is the use of alternative biological matrices such as hair, skin or nails as well as saliva and exhaled breath (86). Hair has the advantages of minimally invasive sampling with simple, convenient storage and the potential for very long window of detection, according hair growth rates (87). MS-based methods have been reported to detect exogenous (88-102) and endogenous (91,103-106) androgens in human hair following long-term, but not single dose (107), exposure. However, hair analysis tests have yet to undergo the rigorous standardization and validation required to become acceptable anti-doping tests in their medicolegal context. Problems that remain to be fully overcome include matrix effects, low recovery and limited sensitivity as well as the impact of age, hair color, alopecia, and shaving or passive chemical (cosmetic) contamination of hair. Additionally nails and skin could also provide analogous information on past androgen exposure with relatively long windows of detection but suitably rigorous tests are yet to be convincingly developed (108). Saliva sampling has also been considered (109,110) for anti-doping application analogous to the use of salivary cortisol measurement for diagnosis of hypercortisolism (111). While potentially applicable to xenobiotic drugs, salivary testosterone immunoassay is not sufficiently accurate (112) and is not suitable to detect testosterone doping because even microscopic blood contamination (e.g. gingivitis, chewing hard food, tooth brushing) produces anomalous high readings. The existence of these renders salivary testosterone testing for antidoping purposes as unreliable by providing opportunity for claims of false positive for any adverse findings. Exhaled breath testing has also been investigated for certain small molecular weight chemicals (113). In theory, androgen-induced gene expression in circulating leukocytes might provide an additional biomarker of androgen action if specific and reproducible signatures can be defined (114); however, as direct detection of androgens is feasible and preferable for proving an ADRV, a role for gene expression biomarkers of androgen action remains to be established for anti-doping.
Indirect Androgen Doping
This doping strategy aims to increase endogenous T production and thereby evades detection by routine screening tests for exogenous T such as urine T/E ratio or CIRMS. Urine hCG is detected by commercial hCG immunoassays using immunoassays specific for intact heterodimeric hCG (including its nicked variant) which, if positive by exceeding a detection threshold (>5 IU/L), requires confirmation by a second immunoassay for intact heterodimeric hCG which is required to prove hCG use. A highly sensitive LC-MS method to detect urine hCG (115) is more specific than immunoassays (116) and has a lower threshold for a positive result in male athletes (117). A key issue is to distinguish a positive hCG urine test, presumptively indicating hCG doping, from early trophoblastic tumor or immunoassay artefacts. As hCG doping is not effective in women and urine hCG screening can detect early pregnancy, an unwarranted privacy intrusion, hCG testing is restricted to male athletes (43). Although direct LH doping is an implausible doping threat (118), suppressed (63,76-78,118) or elevated urine LH may be useful for confirming direct or indirect androgen doping (42,43,76,119). Anti-estrogens (estrogen receptor antagonists) or aromatase inhibitors, which can cause reflex increases in serum and urine LH and testosterone (42), are detected by MS-based chemical detection methods.
Overall, detection of direct androgen doping is now so effective that in WADA-compliant elite competitions it is restricted to the ill-informed, often using counterfeit or unlabeled products (120). Yet the potency of androgen doping in power sports continues to prompt development of novel androgen doping strategies. These will include use of undocumented synthetic androgens, novel indirect androgen doping methods and micro-dosing of natural androgens during out of competition training. The retreat to using micro-dosing inherently reduces the dose-dependent ergogenic benefits of doping while maintaining the risk of detection and disqualification. There remains a need to maintain deterrence by effective detection methods for evolving new androgen doping threats.
HEMOGLOBIN (BLOOD) DOPING
Hemoglobin doping involves either direct blood transfusion or indirect methods of increasing hemoglobin via stimulating erythropoiesis by administration of erythropoietin, its analogs or mimetics (see excellent reviews (121,122)) (Table 4). Boosting hemoglobin is advantageous in aerobic, endurance sports such as road cycling, distance running and cross-country skiing. Maximal oxygen consumption (Vo2), a rate-limiting factor in aerobic exercise, principally determined by cardiac output and blood oxygen transfer with a lesser contribution from tissue oxygen transfer (123). Experiments on exercise tolerance and blood transfusion were first reported in 1945 (124,125)but the scientific basis of hemoglobin doping via enhanced tissue oxygen transfer was firmly established in 1972 by the work of Ekblom et al reporting experiments in healthy volunteers who underwent venesection and/or re-transfusion of 1, 2 or 3 units (400 mL) of blood with repeated testing of maximal exercise-induced oxygen consumption before and after each procedure (126). This proved unequivocally that the maximal oxygen consumption was highly correlated with acute changes in hemoglobin (figure 5). Subsequently, during the 1970-80’s before its banning in 1988, blood transfusion became a prevalent surreptitious practice in road cycling and cross-country skiing and the apparently low prevalence among distance runners may be an underestimate (127). Modelling of historical performance in European road cycling from 1993 onwards shows a unique progression averaging an improvement of 6.4% corresponding closely with the performance enhancement (6-7%) due to rhEPO administration, which is sustained for at least 4 weeks after administration (128-130).
Table 4. Direct and Indirect Hemoglobin Doping and Detection Tests |
|
Doping Mechanism |
Detection |
Direct (Blood transfusion) |
|
Heterologous |
Flow cytometry: bimodal population of blood group antigens |
Autologous |
No direct detection tests. Athletes Biological Passport Biomarkers: Urine phthalate excretion Total hemoglobin mass |
Indirect (Erythropoiesis stimulation) |
|
Direct |
|
rhEpo & biosimilars (>100) Epo analogs |
Urine double immunoblot, (LC-MS) |
Indirect |
|
Hypoxia altitude training, hypoxic sleep area |
Not banned |
Hypoxia-mimetics: hypoxia-inducible factor & stabilizers, iron chelation, cobalt, 2,3 DPG analogs |
LC-MS/MS |
Artificial O2 carriers: HbOC, perfluorocarbons |
LC-MS/MS |
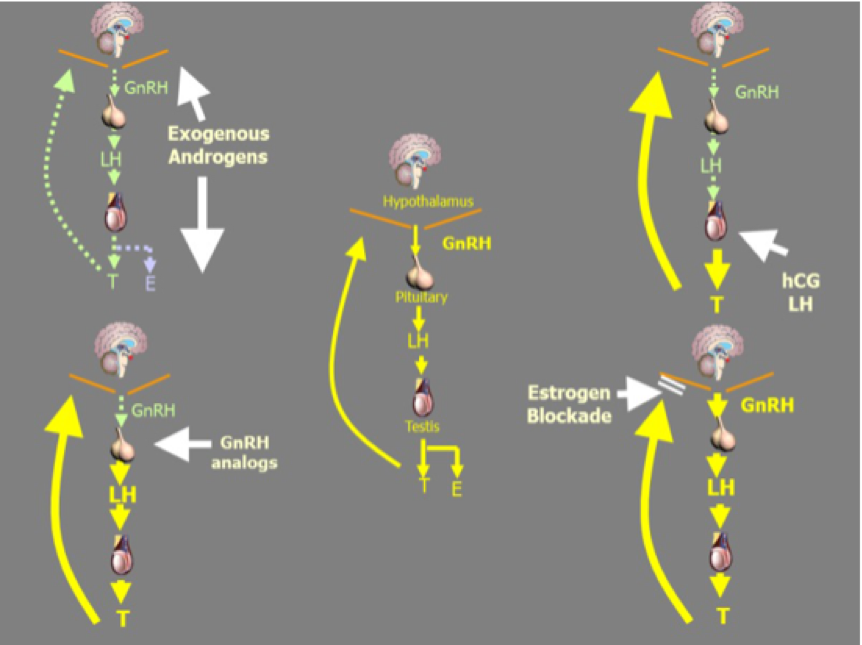
Figure 5. Direct and Indirect Hemoglobin Doping
Blood Transfusion
Transfusion may involve either another person’s (homologous) or the athlete’s own (autologous) blood administered prior to a contest to acutely increase circulating hemoglobin. Homologous blood can be transfused at any convenient time to enhance performance in competition but when performed by untrained personal in non-clinical environments risks transfusion reaction, blood-borne infectious disease, and iron overload. By contrast, autologous transfusion reduces health risks but requires complex coordination as venesection itself is detrimental to performance, and it requires balancing recovery from blood withdrawal and loss of erythrocyte viability during long-term cryostorage with training and competition schedules. Although blood transfusion was first banned by the IOC in 1986, the first practical approach to banning blood doping was the introduction of hematocrit testing in 1997 by the international skiing and cycling federations. These regulations excluded athletes on health grounds from entering competition on the day if their hematocrit exceed a safety threshold (0.50). However, this encouraged hematocrit titration to just below threshold and only prevented competing until hematocrit returned under that threshold, which could be quickly accomplished by venesection. The first ADRV’s for blood manipulation involving hematocrit threshold and titration were in 2001.
Homologous blood transfusion creates a bimodal population of blood group antigens which is detectable by flow cytometry using a panel of 12 minor blood group antigens (131), from the wider array of blood group antigens (132), which can detect a <5% contamination of exogenous erythrocytes. Subsequent refinements simplified and improved test sensitivity so that a panel of 8 antigens can detect contamination comprising a minor admixture population of 0.3-2.0% with no false positives but high sensitivity (~80%), the latter depending on the magnitude of the minor contaminating mixture (133,134). Alternatives based on genotyping for the admixture population of leukocytes have also been proposed (135,136). As a test proving unequivocally the presence of non-endogenous erythrocytes in the circulation, this method is definitive if performed to the required standard. A remotely hypothetical defense against a positive test, based on stable marrow chimerism from a vanished twin, was raised by a cyclist who subsequently admitted transfusion (135). Based on risk of detection as well as to health risks, homologous transfusion has now largely disappeared in favor of autologous transfusion (137).
Autologous Transfusion
The biggest gap in current anti-doping tests is the lack of a specific test to detect autologous transfusion (138). Research to identify robust physico-chemical or biological markers for direct identification of a subpopulation of ex-vivo aged erythrocytes is underway using flow cytometry (139) but the dilution and rapid clearance of effete erythrocytes make for challenging detection problems (140). In the interim, other indirect methods have been developed. These include measuring urinary excretion of phthalates, plasticizers that leach out from the polyvinylchloride blood packs used to store venesected blood (141). This test has brief window of detection (2 day) so will detect auto-transfusion during or immediately before events (characteristic in road cycling, according to convicted dopers) but may miss earlier auto-transfusion. Furthermore, the ubiquity of low-level environmental phthalate exposure requires establishing detection thresholds and non-plastic blood containers can be used. An alternative is the measurement of total hemoglobin mass (142), a measure with good stability and reproducibility even during exercise and circumvents influence of variations in plasma volume such as due to dehydration or dilutional masking (142,143). However, as this requires inhalation of carbon monoxide, which has transient detrimental effects on performance, it is not ideal for routine anti-doping use and its sensitivity may be insufficient to detect all EPO micro-dosing (144,145). Nevertheless, alternative methods for serial measurement of total hemoglobin mass remain attractive. Other hypothetical methods include the detection of microRNA (146) or immune reactions to transfusion (147) but the sensitivity and specificity of these proposed tests remains to be fully evaluated.
The best detection test for autologous hemoglobin doping at present is the hematological module of the ABP introduced in 2009 (148). Conceptually, it is a biomarker test which adopts a Bayesian approach of creating serially-adaptive, person-specific reference limits, based on using all prior testing, to supplant population-based thresholds. Combining all of an individual’s previously collected hematological data creates a probabilistic test of whether any new result deviates significantly from that individual’s personal reference limits (149). These person-specific thresholds allow for ongoing refinement and reinforcement by further testing. The thresholds are calculated by a variety of algorithms incorporating routine hematological parameters, notably hematocrit and reticulocyte counts. Those were developed over the last two decades to create the ABP hematological model which is sensitive to both direct and indirect hemoglobin doping (150). The first attempts to regulate hemoglobin doping in the late 1990’s sought to prevent road cyclists or cross-country skiing athletes competing on health risk grounds when their hematocrit exceeded pre-determined, population-based safety criteria (e.g. hematocrit 0.50 or hemoglobin 170 g/L for cycling). However, while this excluded extreme hemoglobin doping only until the short period when the safety threshold was no longer exceeded, it allowed an increase in an athlete’s natural hematocrit, typically averaging ~0.45, up to the permitted ceiling threshold which fostered titrated hemoglobin doping and manipulations like hemodilution by saline or plasma volume expander infusions to avoid detection (151). More sophisticated hematological algorithms were then developed to detect hemoglobin doping initially for the Sydney 2000 Olympics (152,153), the first generation of algorithms developing validated tests for ongoing and for recent cessation of hemoglobin doping, using a combination of biochemical variables related to erythropoiesis physiology. This approach was simplified by a second generation algorithm using only routine hematological parameters (hemoglobin, reticulocytes) (154), and was subsequently combined with the concept of a sequential development of individual-specific reference ranges (155) into a third generation algorithms (156,157) which were refined for the ABP (148,149). The hematological module of the ABP currently employs an algorithm involving 8 parameters derived from routine hematological profile (hemoglobin, hematocrit, erythrocyte count, reticulocyte count and percentage, mean corpuscular volume, mean corpuscular hemoglobin, mean corpuscular hemoglobin concentration) (158). This is capable of detecting any form of hemoglobin doping, whether direct or indirect, with good but imperfect sensitivity (143-145) and using only routine hematological tests. The reported increasing use of very low EPO doses (“micro-dosing”) would markedly reduce the magnitude of any dose-dependent ergogenic benefits (145) while still carrying risks of detection, disqualification, and disgrace.
Stimulation of Erythropoiesis
Indirect methods to increase hemoglobin include administration of recombinant human EPO or its analogs as well as hypoxia-mimetic drugs (hypoxia-inducible factor stabilizers, iron chelation, cobalt, 2,3 diphosphoglycerate analogs) or artificial oxygen carriers (perfluorocarbons, hemoglobin-based oxygen carriers). Related but non-banned methods include altitude training or its simulation by sleeping in hypoxic rooms which are less effective than hemoglobin doping (129).
The identification of the human EPO gene in 1985 led to the marketing of recombinant human EPO (rhEPO) between 1987-9. Despite the IOC’s prohibition of EPO’s use in sports in 1990, the commercial availability of rhEPO created powerful new opportunities for indirect hemoglobin doping which were soon proven experimentally (159). A drug, which circulates for hours to days, but with potent and long-lasting ergogenic effects after its disappearance due to the 4-month lifespan of erythrocytes, is both attractive for doping and a challenge to anti-doping testing. Expiry of the rhEPO patent in 2004 allowed marketing of a profusion of generic EPO (“biosimilar”) products, estimated globally at over 80 (160), as well as modified EPO analogs (darbepoeitin, pegylated EPO, peginesatide, EPO fusion proteins). A fatal cluster involving deaths of 18 Dutch and Belgian road cyclists, presumably due inadvertent over-dosage during empirical attempts to maximize ergogenic effects of illicit rhEPO, was reported (161), although difficult to verify (162). A similar excess of unexpected deaths of road cyclists was also reported again in 2003-5 when novel EPO analogs and EPO biosimilars were marketed.
Detection of EPO in urine is difficult because of the prevailing low concentrations and need to distinguish exogenous recombinant from endogenous EPO. The first effective method for rhEPO in urine was a double immunoblot (163,164)which was capable of detecting urinary excretion of a variety of exogenous EPO products and analogs according to their differences in glycosylation side-chains, and differences in primary amino acid sequence where they exist, while distinguishing them from endogenous EPO. Although further refined (165) and extended to other EPO analogs (166), the immune-electrophoresis test is sensitive but relatively laborious and provides only a short window of detection of up to a week post-administration (167). More sensitive methods based on proteomics (for EPO analogs with differences in primary structure) together with glycomics (for biosimilars and analogs which have host-cell specific variations in side-chain glycosylation but unchanged natural EPO primary structure (168)) are possible but not yet approved. Additional applications to detect EPO and analogs using dried blood spots have been reported (169). Similarly, preliminary investigations have proposed a EPO-induced gene expression signatures as a biomarker to detect EPO administration but specificity relative to exercise and other physiological effects remain to be clarified (170).
Other EPO mimetics such as hypoxia mimetic drugs including hypoxia-inducible factor (HIF) stabilizers and related small molecules represent growing threats as potential indirect hemoglobin doping agents (171). These non-peptide chemicals interfere with various steps of the molecular oxygen sensing mechanism to mimic renal hypoxia and thereby induce EPO secretion resulting in increased blood hemoglobin. As a convenient orally active alternative to the lucrative pharmaceutical market for injectable erythropoiesis-stimulating peptides (~$7-8 billion (172)) to counteract anemias of chronic renal failure or marrow failure due to myeloproliferative disease or cytotoxic cancer therapy, they constitute a very active area of pre-clinical patent-based clinical drug development (171). Experience suggests that such innovator products can enter the doping black market before marketing approval (150,171). Despite the profusion of pre-clinical leads, they represent families of related chemical structures disclosed in patents for which LC and/or GC-MS detection tests should, in principle, be effective. Understanding the metabolism of these drugs when they come to market may identify long-lasting metabolites that can extend the windows of detection. Coupled with evidence from the ABP, manipulation of the EPO pathway may be detected in conjunction with corroborative measurement of inappropriately suppressed or elevated endogenous EPO for the prevailing hemoglobin level.
HIF is a key generic biological mechanism for tissue sensing of hypoxia and triggering local (neovascularization, angiogenesis) and systemic (EPO) defensive reactions. The promoter of the EPO gene contains enhancer and inhibitor regions with the hypoxia-responsive element which binds HIF and a GATA binding site which enhance and inhibit, respectively, EPO gene transcription. HIF is a heterodimer formed by constitutively expressed subunits with the β subunit in excess and availability of α subunit limiting formation of bioactive HIF. The 3 HIFα subunit isoforms are subject to hydroxylation of specific proline residues by prolyl hydroxylase enzymes which inactivate HIFα by ubiquitination, a tag which targets it to proteasomal degradation. HIFα subunit inactivation is strongly dependent on tissue oxygenation being active during normoxia but reduced during hypoxia when persistence of HIFα stabilizes the HIF heterodimer. Notably, during hypoxia the expression of HIFα in renal cortical cells stimulates EPO gene expression so that HIF stabilization by prolyl hydroxylase inhibitors leads to increased EPO secretion and circulating hemoglobin. Hence inhibiting prolyl hydroxylase activity via blocking its required cofactors (ascorbate, ketoglutarate, iron) using cobalt, nickel, iron chelation, ketoglutarate analogs or mechanism-based chemical inhibitors can result in increased hemoglobin via stimulation of EPO secretion (171). Similarly, small molecule GATA inhibitors potently stimulate circulating EPO, hemoglobin and performance in mice (173) although none have yet been marketed so their human efficacy and safety remain to be determined.
Another approach to increase oxygen delivery to muscle has been to exploit the ability of 2,3 diphophoglycerate (2,3 DPG), whose binding to hemoglobin reduces its affinity for oxygen with the left-shift of its oxygen dissociation curve as an oxygen unloading mechanism in tissues. 2,3 DPG analogs, developed as radiation sensitizers for hypoxic radio-resistant tumors, enhance tissue oxygen delivery in vivo (174,175) but would feature only short-term, acute effects readily detectable by mass spectrometry (176,177).
Adverse effects from use of rhEPO or its analogs are well known in medicine but poorly recognized in doping. They include immunogenicity (with risk of EPO autoantibody mediated pure red cell aplasia) (178,179), cardiovascular complications (including venous thromboembolism, stroke, hypertension and myocardial infarction) and premature death (180-183). In routine clinical use of EPO to correct renal anemia, the goal is a gradual increase to subnormal hemoglobin targets so that the excessive and/or rapid rises in hematocrit and blood viscosity (184) may explain the excess unexplained deaths among young European road cyclists in the late 1980s. In addition, use of rhEPO may deplete iron stores which limits hemoglobin synthesis so that athletes may also use oral or injectable iron supplements, which carry their own risks such as iv iron supplementation’s potentially adverse effects in enhanced tissue oxidative damage and excess mortality in chronic kidney disease (185). Although clinical safety experience with ESAs is restricted to patients with serious medical disorders, there is evidence from the general community that higher natural hematocrit is associated with worse long-term cardiovascular health outcomes (186-188).
GROWTH HORMONE
Growth hormone (GH) is a tissue growth promoter in children but after puberty it is predominantly a metabolic hormone although latent tissue growth promoting effects may be unleashed under non-physiological circumstances, such as during recovery from tissue injury. There is consistent anecdotal evidence that GH has been used in elite sports for decades (189). Nevertheless, ergogenic effects of GH remain unproven and largely speculative as discussed in excellent recent reviews (190-192). Claims of GH benefits in sport have included increases in muscle mass and strength, especially in conjunction with androgens, and/or improved tissue healing with more rapid recovery from either major injuries or minor repetitive injuries, such as from intense physical training allowing for more effective training. The biological basis of ergogenic effects of GH have been tested in these two different scenarios with largely inconclusive findings.
Evidence for direct enhancement of athletic performance by GH has been investigated in two well controlled RCTs with a primary focus on athletic performance. In one study, 96 recreational sub-elite athletes (63 male, 33 female, mean age 28 years) were administered 8 weeks of daily sc injections of GH or placebo with the men also having weekly im injections of T enanthate or saline placebo for the last 5 weeks (193). GH increased lean (muscle) mass (by +2.7 kg) and reduced fat mass (by -1.4 kg) while T increased lean mass (alone by +2.4 kg, by +5.8 kg with GH). The effects of GH were marginally significant for anaerobic sprint capacity (by +3.9%, p=0.05) when pooling male and female participants but this was due to significant effects in men only (by +5.5% alone and +8.3% with GH). However, there were no significant effects on maximal Vo2 consumption, dead lift, or jump height (193). A second study involved 30 healthy non-athletes (15 male, 15 female, mean age 25 years) who were administered daily sc injections of GH at high (4.6 mg/day) or low (2.3 mg/day) doses or placebo (194). There was no significant effect on muscle mass or maximal Vo2 consumption. Additional controlled studies of GH effects but with less focus on athletic performance have also shown that (a) a single dose of GH (~0.8 mg) in 9 recreational athletes did not affect maximal Vo2 or power output in repeated 30 min bursts of bicycle ergometry (195), (b) short term (6 days), low dose GH (~1.7 mg/day) treatment of 48 male androgen abusers withdrawn from androgens for 12 weeks significantly increased maximal Vo2more than placebo (196), (c) daily sc injections of a GH receptor antagonist (pegvisomant) or placebo for 16 days to 20 sedentary men did not change maximal Vo2 although time to exhaustion at 90% maximal Vo2 was reduced (197)and (d) 4 weeks of daily sc injections of GH (~5 mg/day) increased whole body protein synthesis (198), lipolysis and glucose uptake (199) with uncertain significance for athletic performance. Overall, these studies indicate that GH has, at most, a modest ergogenic effect in men only and through enhancing T effects. That is consistent with the fact that young women have markedly greater growth hormone secretion than young men so that growth hormone cannot explain the sex differences in athletic performance (200).
It is also claimed that GH may enhance injury healing, thereby facilitating more intensive training and/or recovery from muscle, connective tissue or bone injury, notably in contact sports. This claim is difficult to evaluate and no well controlled studies of recovery from sports injuries or tolerance of training intensity in elite athletes are reported. The most germane surrogate evidence available arises from investigations on the use of GH in recovery from injuries due to burns, fracture, or for wound healing. A recent Cochrane meta-analysis review of GH treatment effects on recovery from burns injury and healing of donor skin graft sites suggests that GH has a small benefit in skin healing with large burns and reduced hospital stay but there was no benefit in reducing mortality or scarring and adverse effects, notably hyperglycemia, were increased (201). In practice, the increased mortality due to administration of high dose GH in critical illness (202) has led to GH treatment not being widely adopted in clinical practice of treatment of burns. Similarly, the only well controlled study of GH effects on bone healing from fracture reported that, among over 400 patients with tibial fractures treated for up to 16 weeks with GH (1, 2 or 4 mg/day) or placebo, there was no benefit of GH for overall healing (203). Finally, while there are numerous experimental studies of GH or growth factors on wound healing in animal models a wide variety of findings are reported with detrimental, neutral, or beneficial effects but no well-controlled human studies are available. In summary, the available evidence for improved tissue repair or regeneration is minimal.
Important caveats on interpreting these few well designed studies are that the effects of higher GH and T doses, as used in doping, have not been studied so that more potent higher dose and/or interactive effects cannot be excluded in the absence of well controlled, high dose, placebo-controlled studies. Nevertheless, the hypothesis that high dose GH exposure would enhance muscular function is inconsistent with the experience of acromegaly in which patients experience much higher (25-100 times) growth hormone exposure than doses that can be ethically administered to healthy human volunteers (204), yet characteristically display muscular weakness rather than increased muscle size or strength (205). Anti-doping science history suggests that caution is required before rejecting evidence for claimed ergogenic effects without investigations replicating the pharmacological doses used.
Furthermore, safety analysis is not feasible based on the few, small, short-term studies of GH’s potential ergogenic effects; however, there are significant safety concerns about the long-term risk of cancer following GH administration. Even standard therapeutic GH doses administered to GH deficient children are associated with increased risk of second cancers in some (206-208) but not all (209) follow-up studies although these risks appear largely confined to survivors of childhood cancers and its treatment which render them GH deficient (210-213). Although the significant cancer risk based on uncontrolled observational cohort data using standard GH doses remains contentious (214,215), the long-term risks of much higher GH doses used illicitly by athletes must be viewed with significant concern.
Detection of GH doping remains difficult (216). A major challenge is the non-glycosylated primary structure of recombinant and endogenous 22 kDa GH, that lack the distinctive side-chain carbohydrate differences of exogenous glycoproteins EPO or hCG which provide a convenient basis for sensitive molecular detection tests. Nevertheless, minor infidelities in commercial manufacturing of GH may incorporate distinctive non-natural chemical features proving an exogenous origin (217-219) although these findings have not been developed into detection tests. Challenges to the detection of GH doping arise from the physiological pattern of endogenous GH secretion with its intermittent, pulsatile pattern subject to prominent influence of exercise, stress, and nutritional effects together with GH’s brief circulating half-life and low urine concentrations (220,221). Like other major doping classes, there are both direct and indirect forms of GH doping, involving either direct administration of GH or IGF-I or their analogs and indirect GH doping involving drugs that aim to increase endogenous GH and IGF-I secretion (Table 5).
Table 5. Growth Factors, Growth Hormone Related and Other Peptides |
||||
Growth Factors |
Growth Hormone Related Peptides |
Other Peptides |
||
|
GHRH analogs |
Ghrelin analogs |
Other |
|
FGFs |
GHRH |
Lenomorelin (ghrelin) |
IGF-1 & analogs (MGF, long R3IGF-1) |
Thymosinß4 |
HGF |
CJC-1295 |
GHRP-1 |
IGF-2 |
|
MGF |
CJC-1293 |
GHRP-2 (pralmorelin) |
Insulin & analogs |
|
PDGF |
Sermorelin |
GHRP-3 |
AOD-9604 |
|
VEGF |
Tesamorelin |
GHRP-4 |
hGH 176-191 |
|
|
|
GHRP-5 |
|
|
|
|
GHRP-6 |
|
|
|
|
Hexarelin |
|
|
|
|
Ipamorelin |
|
|
|
|
Alexamorelin |
|
|
|
|
Anamorelin |
|
|
|
|
Macimorelin |
|
|
|
|
Tabimorelin |
|
|
|
|
Examorelin |
|
|
The first test to detect administration of exogenous GH, the 22kD recombinant form of human GH, was based on blood sampling to measure the ratio of circulating isoforms of GH recognizing the fact that the pituitary secretes not only the major 22 kD isoform (65-80%) but also a variety of minor isoforms including a wide variety of minor isoforms and their multimeric variants (222). Administration of exogenous GH suppresses endogenous pituitary GH secretion leading to a predominance of circulating 22 kD GH. This is the basis for the GH isoform ratio test whereby a serum sample is measured by two different GH immunoassays, one with predominant 22 kD GH specificity (“rec” assay) and the other recognizing the broad spectrum of pituitary GH isoforms (“pit” assay) and the ratio of results (“rec”/”pit” ratio) is an index to detect administration of exogenous recombinant GH (220,223). This ratio test then serves to detect administration of exogenous recombinant human 22kD GH analogous to detection of exogenous T by the urine T/E ratio and exogenous insulin by analysis of serum C peptide (224). The differential GH isoform ratio test has undergone extensive validation involving standardization of the two GH immunoassays with distinctive immunoreactivities to quantify 20kD and 22kD epitopes as well as its application to various populations of elite athletes and evaluating physiological factors which might impact on the validity of test read-out. A strength of this test is that it is aimed at the exogenous doping agent itself, although it cannot definitively distinguish it from its endogenous counterpart. The major limitations of this differential isotope ratio test are its narrow window of detection (24-36 hr post administration) and its inability to detect indirect GH doping. While pituitary-derived human GH might not be detected, human pituitary GH, once obtained from national scale pituitary collection and purification programs, has not been available since 1985 when its risks of Creutzfeldt-Jakob disease were identified (225,226) with recombinant human GH replacing pituitary-extracted GH worldwide. This differential isoform test was first introduced for the 2004 Olympics (227) and led in 2010 to the first successful detection of out of competition GH doping (228).
A complementary detection test with a wider window of detection has been developed based on biomarkers of GH action. This uses two serum biomarkers of tissue GH effects, circulating IGF-1 as a short-term marker of hepatic GH action, and N-terminal peptide of procollagen type III (PIII-NP) as a long-term marker of GH-dependent collagen synthesis. In a study of 102 recreational athletes (53 male, 49 female, mean age 25 years, from 4 different European cities) randomly assigned to self-inject 2.7 mg or 5.4 mg GH or placebo once daily, measurement of serum IGF-1 and PIII-NP by specific immunoassays were able to correctly classify 86% of samples from males and 60% of samples from female using an empirical linear discriminant analysis of log-transformed serum IGF-1 and PIII-NP at the specificity of 1:10,000 required for a WADA biomarker threshold (229). Subsequent studies have shown that additional collagen biomarkers, N-terminal propeptide and C-terminal telopeptide of type I collagen, further widen the window of detection for GH administration (230,231). This multiplex biomarker test, based on using standardized immunoassay antibodies, requires establishment of reliable reference range with specificity (false positive detection rate) of no more than 1:10,000 incorporating the impact of gender and age, although exercise, injury, ethnicity and sports type appear not to be confounding influences but is not yet in routine use by WADA anti-doping labs. The two GH doping test, the differential isoform and biomarker approaches, are considered ultimately complementary (232).
IGF and Insulin Doping
IGF-1 is a circulating marker of hepatic GH effects and mediator of GH action so the marketing in 2005 of recombinant human IGF-I alone, and later with its major binding protein recombinant human IGF binding protein 3 (IGF-BP3) (233), for treatment of diabetes, insulin or GH insensitivity or motor neuron disease, together with the availability of IGF-1 analogs for laboratory use, creates the possibility of IGF doping (234). Time-series analysis of elite sports performance (235) is consistent with the occurrence of IGF-1 doping but its prevalence is unknown (56). As the biological basis for ergogenic effects of IGFs is due to its GH-like effects, this remains largely speculative and accompanied by the same safety concerns. IGF-1, IGF-2 and their analogs (236) as well as insulin and its analogs (237) are all readily detectable by LC-tandem MS and preliminary evidence suggests that biomarkers for IGF-1 administration (IGF-2, IGFBP2) may widen the window of detection (238). However, a specific test to detect IGF doping remains to be established (239).
MGF is a splice variant of IGF-I which, although not known to appear in the circulation, have any pharmacological effects, or be approved for human use (240), is advertised on the black-market and internet (241) for alleged anabolic or tissue repair/regeneration benefits. Like other short peptide with known structure, it is readily detectable using LC- tandem MS (241).
Insulin has long been used in doping and was prohibited in sports since 1999 (242,243). Other than its proper medical use in diabetics, the use of insulin and its analogs for doping is based solely on its easy availability coupled with anecdotal information from other drug users. There are no clinical studies showing any ergogenic effects of insulin or its analogs in non-diabetic individuals. The doping folklore appears to arise from the classification of insulin as being “anabolic”, in a loose generalization and mistaken analogy to androgens. In healthy non-diabetic individuals, insulin and its synthetic analogs stimulate weight gain via hypoglycemia and increasing appetite, but produce fat rather than muscle gain. The adverse effects include hypoglycemia, hypokalemia, injection-related infections and weight (fat) gain. Doping detection tests for insulin and its analogs continue to evolve and focus on highly sensitive and specific quantitative MS-based proteomics (244).
Growth Factors, GH Releasing and Other Peptides
For the unscrupulous in pursuit of the unlawful, the increasingly stringent detection of androgen and hemoglobin doping, the two most potent classes of ergogenic drugs, has led to new, highly speculative form of doping involving peptide growth factors and GH releasing peptides. These are within the size range of automated bulk custom peptide synthesis and are marketed cheaply by chemical manufacturers. While notionally sold solely for laboratory research, these unregulated products are available for purchase over the internet. Promoted by speculative fantasies on their mode of action coupled with testimonials to their efficacy but without objective testing or assurance of safety in humans, they are believed to be widely used by gullible and/or desperate athletes and their trainers. As unregistered drugs, this growing range of peptides appears to constitute a greater threat to athlete’s health than a risk of effective cheating.
The S2 category of Prohibited Substances lists, in addition to GH and IGF-1, GH fragments and releasing peptides, a wide array of growth factors and modulators and, crucially, a generic catch-all provision for unnamed growth factors and peptides which may affect connective, vascular, muscular, or regenerative tissues or energy utilization and other substances with similar chemical structure or biological effects.
The major category of oligopeptides used for doping is the class of GH releasing peptides analogs of the endogenous GH releasing peptides, GHRH and ghrelin, whereby their analogs aim to increase endogenous GH secretion and are therefore banned (table 5). Most of these peptide were developed in the pharmaceutical industry from the 1990s aiming to provide cheaper, orally active, non-peptide agonists with capacity for sustained stimulation of endogenous GH secretion to “rejuvenate the GH/IGF-1 axis” (245), an unusually explicit acknowledgment of the regular nexus between hormonal rejuvenation and doping (246). However, none of these hormonal peptides have been registered for human therapeutic use with only one (pralmorelin) registered for single-dose, diagnostic use (for GH deficiency) in Japan and unacetylated cyclin ghrelin marketed in Europe for Prader-Willi syndrome. Although they may stimulate GH release initially, many failed to achieve sustained GH release due to desensitization and none achieved meaningful clinical improvements in any target diseases. If their unproven ergogenic benefits are due to sustained GH release this renders them unlikely to be beneficial; nevertheless, the caveat on not accepting negative conclusions without direct testing are also relevant to this class of peptides. Like other short peptides, once chemical structures are known, detection is readily feasible using LC-MS (247,248). The illicit nature of this market raises the risks of counterfeit and unsafe products with attendant risks of infection and residual toxic contaminants unlike the purity pharmaceutical product manufacturers are required to demonstrate by batch release testing.
PROGRESS, GAPS, AND FUTURE PROSPECTS
Anti-doping science continues to make major progress over recent decades especially since the advent of WADA with its harmonization and focus on deterrence through standardized testing. Progress from improved MS-based testing methodologies and instrumentation, summarized annually by the editor (M Thevis) of the major antidoping science journal, Drug Testing and Analysis (249), is evident from the increasing numbers of ADRV findings among frozen stored urine samples now banked for 10 years from previous Olympics. Like any efforts to combat human malfeasance, the quest for drug-free and safe sport requires ongoing vigilance and continual renewal of intelligence-based detection testing. While great progress has been made in the two canonical forms of doping, androgen and hemoglobin doping, human ingenuity continually finds way to challenge the testing just as traditional frauds are supplanted by cyber-crime and ingenious computer hacking. It is important to bear in mind that the winning margin (defined as the difference in performance between gold and silver medals, getting a medal or not, making a final or not in the Olympic athletic or swimming events) is <1% (200) so even small systematic advantages may be important motives and unfair advantages for doping.
The major gaps remaining in anti-doping science are (a) the lack of a definitive test for autologous blood transfusion, (b) need for more sensitive detection tests for peptide doping with wider windows of detection and (c) more economical, affordable and robust sample handling and storage procedures including dried blood spot sampling. These challenges must be met by adapting novel technologies such as quantitative proteomics, genomics, and metabolomics as well as implementing more out of competition and blood testing. Such progress depends on innovative applied research which is supported by WADA, Partnership for Clean Competition and certain national anti-doping organizations together with regular peer-review research granting agencies. Finally, the development of effective forensic intelligence investigations, a slow, complex and costly process but which can have salutary effects (e.g. for road cycling in the Lance Armstrong case), is proving a valuable complementary approach as an adjunct to effective laboratory testing.
REFERENCES
- Handelsman DJ, Gooren LJ. Hormones and sport: physiology, pharmacology and forensic science. Asian J Androl 2008; 10:348-350
- Rawls J. Justice as Fairness: A Restatement. Cambridge, MA: Belknap, Harvard University Press.
- Ryan A. Fairness and philosopy. Social Research 2006; 73:597-606
- Wiesing U. Should performance-enhancing drugs in sport be legalized under medical supervision? Sports Med 2011; 41:167-176
- Shuster S, Devine JW. The banning of sportsmen and women who fail drug tests is unjustifiable. The Journal of the Royal College of Physicians of Edinburgh 2013; 43:39-43
- Savulescu J, Creaney L, Vondy A. Should athletes be allowed to use performance enhancing drugs? BMJ 2013; 347:f6150
- Uvacsek M, Nepusz T, Naughton DP, Mazanov J, Ranky MZ, Petroczi A. Self-admitted behavior and perceived use of performance-enhancing vs psychoactive drugs among competitive athletes. Scandinavian journal of medicine & science in sports 2011; 21:224-234
- Petroczi A, Uvacsek M, Nepusz T, Deshmukh N, Shah I, Aidman EV, Barker J, Toth M, Naughton DP. Incongruence in doping related attitudes, beliefs and opinions in the context of discordant behavioural data: in which measure do we trust? PLoS One 2011; 6:e18804
- Dunn M, Thomas JO, Swift W, Burns L, Mattick RP. Drug testing in sport: the attitudes and experiences of elite athletes. Int J Drug Policy 2010; 21:330-332
- Bloodworth AJ, Petroczi A, Bailey R, Pearce G, McNamee MJ. Doping and supplementation: the attitudes of talented young athletes. Scandinavian journal of medicine & science in sports 2012; 22:293-301
- Lamberti N, Malagoni AM, Felisatti M, Caracciolo S, Resch N, Litmanen H, Dalfollo D, Jeannier P, Zhukovskaja L, Carrabre JE, Manfredini F. Antidoping attitudes among elite athletes: a cross sectional study in biathlon using a suitably developed questionnaire. J Sports Med Phys Fitness 2017; 57:610-623
- Backhouse SH, Whitaker L, Petroczi A. Gateway to doping? Supplement use in the context of preferred competitive situations, doping attitude, beliefs, and norms. Scandinavian journal of medicine & science in sports 2013; 23:244-252
- Morente-Sanchez J, Zabala M. Doping in sport: a review of elite athletes' attitudes, beliefs, and knowledge. Sports Med 2013; 43:395-411
- Petroczi A, Mazanov J, Nepusz T, Backhouse SH, Naughton DP. Comfort in big numbers: Does over-estimation of doping prevalence in others indicate self-involvement? J Occup Med Toxicol 2008; 3:19
- Dunn M, Thomas JO, Swift W, Burns L. Elite athletes' estimates of the prevalence of illicit drug use: evidence for the false consensus effect. Drug Alcohol Rev 2012; 31:27-32
- Thomas H. Murray. Good Sport. Oxford University Press.
- Eynon N, Ruiz JR, Oliveira J, Duarte JA, Birk R, Lucia A. Genes and elite athletes: a roadmap for future research. J Physiol 2011; 589:3063-3070
- de la Chapelle A, Traskelin AL, Juvonen E. Truncated erythropoietin receptor causes dominantly inherited benign human erythrocytosis. Proc Natl Acad Sci U S A 1993; 90:4495-4499
- Berman Y, North KN. A gene for speed: the emerging role of alpha-actinin-3 in muscle metabolism. Physiology (Bethesda) 2010; 25:250-259
- Wade N. Anabolic Steroids: Doctors Denounce Them, but Athletes Aren't Listening. Science 1972; 176:1399-1403
- Ljungqvist A. Half a century of challenges. Bioanalysis 2012; 4:1531-1533
- WADA. World Anti-Doping Code. Montreal: https://www.wada-ama.org/en/resources/the-code/world-anti-doping-code;2015.
- Egner IM, Bruusgaard JC, Eftestol E, Gundersen K. A cellular memory mechanism aids overload hypertrophy in muscle long after an episodic exposure to anabolic steroids. J Physiol 2013; 591:6221-6230
- de Hon O, Kuipers H, van Bottenburg M. Prevalence of doping use in elite sports: a review of numbers and methods. Sports Med 2015; 45:57-69
- Lensveldt-Mulders GJL, Hox JJ, van der Heijden PGM, Maas CJM. Meta-analysis of randomized response research. Socieological Methods & Research 2005; 33:319-348
- Ulrich R, Pope HG, Jr., Cleret L, Petroczi A, Nepusz T, Schaffer J, Kanayama G, Comstock RD, Simon P. Doping in Two Elite Athletics Competitions Assessed by Randomized-Response Surveys. Sports Med 2018; 48:211-219
- Schroter H, Studzinski B, Dietz P, Ulrich R, Striegel H, Simon P. A Comparison of the Cheater Detection and the Unrelated Question Models: A Randomized Response Survey on Physical and Cognitive Doping in Recreational Triathletes. PLoS One 2016; 11:e0155765
- WADA. International Standard for Therapeutic Use Exemptions. 2014. https://wada-main-prod.s3.amazonaws.com/resources/files/WADA-2015-ISTUE-Final-EN.pdf. Accessed Oct 2014
- Allan CM, Couse JF, Simanainen U, Spaliviero J, Jimenez M, Rodriguez K, Korach KS, Handelsman DJ. Estradiol induction of spermatogenesis is mediated via an estrogen receptor-{alpha} mechanism involving neuroendocrine activation of follicle-stimulating hormone secretion. Endocrinology 2010; 151:2800-2810
- WADA. Medical Information to Support the Decisions of TUECs - Androgen Deficiency-Male Hypogonadism. Montreal: WADA; April 20015 2015.
- David K, Dingemanse E, Freud J, Laqueur E. Uber krystallinisches mannliches Hormon aus Hoden (Testosteron), wirksamer als aus Harn oder aus Cholestrin bereitetes Androsteron. Hoppe Seylers Zeischrift Physiologische Chemie 1935; 233:281-282
- Yesalis CE, Courson SP, Wright JE. History of anabolic steroid use in sport and exercise. In: Yesalis CE, ed. Anabolic Steroids in Sports and Exercise. Champaign, IL: Human Kinetics; 2000:51-71.
- Franke WW, Berendonk B. Hormonal doping and androgenization of athletes: a secret program of the German Democratic Republic government. Clin Chem 1997; 43:1262-1279
- Ryan AJ. Anabolic steroids are fool's gold. Fed Proc 1981; 40:2682-2688
- Elashoff JD, Jacknow AD, Shain SG, Braunstein GD. Effects of anabolic-androgenic steroids on muscular strength. Ann Intern Med 1991; 115:387-393
- Bhasin S, Storer TW, Berman N, Callegari C, Clevenger B, Phillips J, Bunnell TJ, Tricker R, Shirazi A, Casaburi R. The effects of supraphysiologic doses of testosterone on muscle size and strength in normal men. N Engl J Med 1996; 335:1-7
- Woodhouse LJ, Reisz-Porszasz S, Javanbakht M, Storer TW, Lee M, Zerounian H, Bhasin S. Development of models to predict anabolic response to testosterone administration in healthy young men. Am J Physiol Endocrinol Metab 2003; 284:E1009-1017
- Storer TW, Magliano L, Woodhouse L, Lee ML, Dzekov C, Dzekov J, Casaburi R, Bhasin S. Testosterone dose-dependently increases maximal voluntary strength and leg power, but does not affect fatigability or specific tension. J Clin Endocrinol Metab 2003; 88:1478-1485
- Bhasin S, Woodhouse L, Casaburi R, Singh AB, Mac RP, Lee M, Yarasheski KE, Sinha-Hikim I, Dzekov C, Dzekov J, Magliano L, Storer TW. Older men are as responsive as young men to the anabolic effects of graded doses of testosterone on the skeletal muscle. J Clin Endocrinol Metab 2005; 90:678-688
- Thevis M, Schanzer W. Detection of SARMs in doping control analysis. Mol Cell Endocrinol 2018; 464:34-45
- Stenman UH, Hotakainen K, Alfthan H. Gonadotropins in doping: pharmacological basis and detection of illicit use. Br J Pharmacol 2008; 154:569-583
- Handelsman DJ. Indirect androgen doping by oestrogen blockade in sports. Br J Pharmacol 2008; 154:598-605
- Handelsman DJ. Clinical review: The rationale for banning human chorionic gonadotropin and estrogen blockers in sport. J Clin Endocrinol Metab 2006; 91:1646-1653
- Mauras N, Rogol AD, Veldhuis JD. Appraising the instantaneous secretory rates of luteinizing hormone and testosterone in response to selective mu opiate receptor blockade in late pubertal boys. J Androl 1987; 8:203-209
- Kicman AT. Pharmacology of anabolic steroids. Br J Pharmacol 2008; 154:502-521
- Thevis M, Schanzer W. Synthetic anabolic agents: steroids and nonsteroidal selective androgen receptor modulators. Handb Exp Pharmacol 2010:99-126
- Ponzetto F, Boccard J, Baume N, Kuuranne T, Rudaz S, Saugy M, Nicoli R. High-resolution mass spectrometry as an alternative detection method to tandem mass spectrometry for the analysis of endogenous steroids in serum. J Chromatogr B Analyt Technol Biomed Life Sci 2017; 1052:34-42
- Palonek E, Ericsson M, Garevik N, Rane A, Lehtihet M, Ekstrom L. Atypical excretion profile and GC/C/IRMS findings may last for nine months after a single dose of nandrolone decanoate. Steroids 2016; 108:105-111
- Sobolevsky T, Rodchenkov G. Detection and mass spectrometric characterization of novel long-term dehydrochloromethyltestosterone metabolites in human urine. J Steroid Biochem Mol Biol 2012; 128:121-127
- Sobolevsky T, Rodchenkov G. Mass spectrometric description of novel oxymetholone and desoxymethyltestosterone metabolites identified in human urine and their importance for doping control. Drug Test Anal 2012; 4:682-691
- Thevis M, Piper T, Beuck S, Geyer H, Schanzer W. Expanding sports drug testing assays: mass spectrometric characterization of the selective androgen receptor modulator drug candidates RAD140 and ACP-105. Rapid Commun Mass Spectrom 2013; 27:1173-1182
- Rzeppa S, Viet L. Analysis of sulfate metabolites of the doping agents oxandrolone and danazol using high performance liquid chromatography coupled to tandem mass spectrometry. J Chromatogr B Analyt Technol Biomed Life Sci 2016; 1029-1030:1-9
- Liu J, Chen L, Joseph JF, Nass A, Stoll A, de la Torre X, Botre F, Wolber G, Parr MK, Bureik M. Combined chemical and biotechnological production of 20betaOH-NorDHCMT, a long-term metabolite of Oral-Turinabol (DHCMT). J Inorg Biochem 2018; 183:165-171
- Esquivel A, Alechaga E, Monfort N, Ventura R. Sulfate metabolites improve retrospectivity after oral testosterone administration. Drug Test Anal 2019; 11:392-402
- Esquivel A, Alechaga E, Monfort N, Yang S, Xing Y, Moutian W, Ventura R. Evaluation of sulfate metabolites as markers of intramuscular testosterone administration in Caucasian and Asian populations. Drug Test Anal 2019; 11:1218-1230
- Kohler M, Thomas A, Geyer H, Petrou M, Schanzer W, Thevis M. Confiscated black market products and nutritional supplements with non-approved ingredients analyzed in the Cologne Doping Control Laboratory 2009. Drug Test Anal 2010; 2:533-537
- Handelsman DJ, Bermon S. Detection of testosterone doping in female athletes. Drug Test Anal 2019; 11:1566-1571
- Saugy M, Lundby C, Robinson N. Monitoring of biological markers indicative of doping: the athlete biological passport. Br J Sports Med 2014; 48:827-832
- Xue Y, Sun D, Daly A, Yang F, Zhou X, Zhao M, Huang N, Zerjal T, Lee C, Carter NP, Hurles ME, Tyler-Smith C. Adaptive evolution of UGT2B17 copy-number variation. Am J Hum Genet 2008; 83:337-346
- Schulze JJ, Lundmark J, Garle M, Skilving I, Ekstrom L, Rane A. Doping test results dependent on genotype of uridine diphospho-glucuronosyl transferase 2B17, the major enzyme for testosterone glucuronidation. J Clin Endocrinol Metab 2008; 93:2500-2506
- Schulze JJ, Lundmark J, Garle M, Ekstrom L, Sottas PE, Rane A. Substantial advantage of a combined Bayesian and genotyping approach in testosterone doping tests. Steroids 2009; 74:365-368
- Sottas PE, Saugy M, Saudan C. Endogenous steroid profiling in the athlete biological passport. Endocrinol Metab Clin North Am 2010; 39:59-73, viii-ix
- Martin-Escudero P, Munoz-Guerra JA, Garcia-Tenorio SV, Garde ES, Soldevilla-Navarro AB, Galindo-Canales M, Prado N, Fuentes-Ferrer ME, Fernandez-Perez C. Impact of the UGT2B17 polymorphism on the steroid profile. Results of a crossover clinical trial in athletes submitted to testosterone administration. Steroids 2019; 141:104-113
- Shackleton CH, Roitman E, Phillips A, Chang T. Androstanediol and 5-androstenediol profiling for detecting exogenously administered dihydrotestosterone, epitestosterone, and dehydroepiandrosterone: potential use in gas chromatography isotope ratio mass spectrometry. Steroids 1997; 62:665-673
- Piper T, Thevis M. Applications of Isotope Ratio Mass Spectrometry in Sports Drug Testing Accounting for Isotope Fractionation in Analysis of Biological Samples. Methods Enzymol 2017; 596:403-432
- Piper T, Mareck U, Geyer H, Flenker U, Thevis M, Platen P, Schanzer W. Determination of 13C/12C ratios of endogenous urinary steroids: method validation, reference population and application to doping control purposes. Rapid Commun Mass Spectrom 2008; 22:2161-2175
- Cawley AT, Trout GJ, Kazlauskas R, Howe CJ, George AV. Carbon isotope ratio (delta13C) values of urinary steroids for doping control in sport. Steroids 2009; 74:379-392
- Van Renterghem P, Van Eenoo P, Sottas PE, Saugy M, Delbeke F. Subject-based steroid profiling and the determination of novel biomarkers for DHT and DHEA misuse in sports. Drug Test Anal 2010; 2:582-588
- Brooker L, Cawley A, Drury J, Edey C, Hasick N, Goebel C. Stable carbon isotope ratio profiling of illicit testosterone preparations--domestic and international seizures. Drug Test Anal 2014; 6:996-1001
- Cawley A, Collins M, Kazlauskas R, Handelsman DJ, Heywood R, Longworth M, Arenas-Queralt A. Stable isotope ratio profiling of testosterone preparations. Drug Test Anal 2010; 2:557-567
- Van Renterghem P, Van Eenoo P, Sottas PE, Saugy M, Delbeke F. A pilot study on subject-based comprehensive steroid profiling: novel biomarkers to detect testosterone misuse in sports. Clin Endocrinol (Oxf) 2011;
- Jardines D, Botre F, Colamonici C, Curcio D, Procida G, de la Torre X. Longitudinal evaluation of the isotope ratio mass spectrometric data: towards the 'isotopic module' of the athlete biological passport? Drug Test Anal 2016; 8:1212-1221
- Piper T, Emery C, Saugy M. Recent developments in the use of isotope ratio mass spectrometry in sports drug testing. Anal Bioanal Chem 2011; 401:433-447
- Piper T, Emery C, Thomas A, Saugy M, Thevis M. Combination of carbon isotope ratio with hydrogen isotope ratio determinations in sports drug testing. Anal Bioanal Chem 2013; 405:5455-5466
- Thevis M, Piper T, Horning S, Juchelka D, Schanzer W. Hydrogen isotope ratio mass spectrometry and high-resolution/high-accuracy mass spectrometry in metabolite identification studies: detecting target compounds for sports drug testing. Rapid Commun Mass Spectrom 2013; 27:1904-1912
- Kicman AT, Brooks RV, Collyer SC, Cowan DA, Nanjee MN, Southan GJ, Wheeler MJ. Criteria to indicate testosterone administration. Br J Sports Med 1990; 24:253-264
- Palonek E, Gottlieb C, Garle M, Bjorkhem I, Carlstrom K. Serum and urinary markers of exogenous testosterone administration. J Steroid Biochem Mol Biol 1995; 55:121-127
- Goebel C, Howe CJ, Ho KK, Nelson A, Kazlauskas R, Trout GJ. Screening for testosterone abuse in male athletes using the measurement of urinary LH, a revision of the paradigm. Drug Test Anal 2009; 1:511-517
- Akram ON, Bursill C, Desai R, Heather AK, Kazlauskas R, Handelsman DJ, Lambert G. Evaluation of androgenic activity of nutraceutical-derived steroids using mammalian and yeast in vitro androgen bioassays. Anal Chem 2011; 83:2065-2074
- Handelsman DJ, Heather A. Androgen abuse in sports. Asian J Androl 2008; 10:403-415
- Zierau O, Lehmann S, Vollmer G, Schanzer W, Diel P. Detection of anabolic steroid abuse using a yeast transactivation system. Steroids 2008; 73:1143-1147
- Houtman CJ, Sterk SS, van de Heijning MP, Brouwer A, Stephany RW, van der Burg B, Sonneveld E. Detection of anabolic androgenic steroid abuse in doping control using mammalian reporter gene bioassays. Anal Chim Acta 2009; 637:247-258
- Cadwallader AB, Lim CS, Rollins DE, Botre F. The androgen receptor and its use in biological assays: looking toward effect-based testing and its applications. J Anal Toxicol 2011; 35:594-607
- Death AK, McGrath KC, Kazlauskas R, Handelsman DJ. Tetrahydrogestrinone is a potent androgen and progestin. J Clin Endocrinol Metab 2004; 89:2498-2500
- McRobb L, Handelsman DJ, Kazlauskas R, Wilkinson S, McLeod MD, Heather AK. Structure-activity relationships of synthetic progestins in a yeast-based in vitro androgen bioassay. Journal of Steroid Biochemistry and Molecular Biology 2008; 110:39-47
- Thevis M, Geyer H, Tretzel L, Schanzer W. Sports drug testing using complementary matrices: Advantages and limitations. J Pharm Biomed Anal 2016; 130:220-230
- Kintz P. Testing for anabolic steroids in hair: a review. Legal medicine 2003; 5 Suppl 1:S29-33
- Deng XS, Kurosu A, Pounder DJ. Detection of anabolic steroids in head hair. J Forensic Sci 1999; 44:343-346
- Kintz P, Cirimele V, Sachs H, Jeanneau T, Ludes B. Testing for anabolic steroids in hair from two bodybuilders. Forensic Sci Int 1999; 101:209-216
- Kintz P, Cirimele V, Jeanneau T, Ludes B. Identification of testosterone and testosterone esters in human hair. J Anal Toxicol 1999; 23:352-356
- Hold KM, Borges CR, Wilkins DG, Rollins DE, Joseph RE, Jr. Detection of nandrolone, testosterone, and their esters in rat and human hair samples. J Anal Toxicol 1999; 23:416-423
- Gaillard Y, Vayssette F, Balland A, Pepin G. Gas chromatographic-tandem mass spectrometric determination of anabolic steroids and their esters in hair. Application in doping control and meat quality control. J Chromatogr B Biomed Sci Appl 1999; 735:189-205
- Gaillard Y, Vayssette F, Pepin G. Compared interest between hair analysis and urinalysis in doping controls. Results for amphetamines, corticosteroids and anabolic steroids in racing cyclists. Forensic Sci Int 2000; 107:361-379
- Cirimele V, Kintz P, Ludes B. Testing of the anabolic stanozolol in human hair by gas chromatography-negative ion chemical ionization mass spectrometry. J Chromatogr B Biomed Sci Appl 2000; 740:265-271
- Kintz P, Cirimele V, Ludes B. Discrimination of the nature of doping with 19-norsteroids through hair analysis. Clin Chem 2000; 46:2020-2022
- Kintz P, Cirimele V, Dumestre-Toulet V, Ludes B. Doping control for nandrolone using hair analysis. J Pharm Biomed Anal 2001; 24:1125-1130
- Dumestre-Toulet V, Cirimele V, Ludes B, Gromb S, Kintz P. Hair analysis of seven bodybuilders for anabolic steroids, ephedrine, and clenbuterol. J Forensic Sci 2002; 47:211-214
- Kintz P, Cirimele V, Dumestre-Toulet V, Villain M, Ludes B. Doping control for methenolone using hair analysis by gas chromatography-tandem mass spectrometry. J Chromatogr B Analyt Technol Biomed Life Sci 2002; 766:161-167
- Bresson M, Cirimele V, Villain M, Kintz P. Doping control for metandienone using hair analyzed by gas chromatography-tandem mass spectrometry. J Chromatogr B Analyt Technol Biomed Life Sci 2006; 836:124-128
- Gambelunghe C, Sommavilla M, Ferranti C, Rossi R, Aroni K, Manes N, Bacci M. Analysis of anabolic steroids in hair by GC/MS/MS. Biomedical chromatography : BMC 2007; 21:369-375
- Deshmukh N, Hussain I, Barker J, Petroczi A, Naughton DP. Analysis of anabolic steroids in human hair using LC-MS/MS. Steroids 2010; 75:710-714
- Strano-Rossi S, Castrignano E, Anzillotti L, Odoardi S, De-Giorgio F, Bermejo A, Pascali VL. Screening for exogenous androgen anabolic steroids in human hair by liquid chromatography/orbitrap-high resolution mass spectrometry. Anal Chim Acta 2013; 793:61-71
- Kintz P, Cirimele V, Ludes B. Physiological concentrations of DHEA in human hair. J Anal Toxicol 1999; 23:424-428
- Kintz P, Cirimel V, Devaux M, Ludes B. Dehydroepiandrosterone (DHEA) and testosterone concentrations in human hair after chronic DHEA supplementation. Clin Chem 2000; 46:414-415
- Rambaud L, Monteau F, Deceuninck Y, Bichon E, Andre F, Le Bizec B. Development and validation of a multi-residue method for the detection of a wide range of hormonal anabolic compounds in hair using gas chromatography-tandem mass spectrometry. Anal Chim Acta 2007; 586:93-104
- Deshmukh NI, Barker J, Petroczi A, Naughton DP. Detection of testosterone and epitestosterone in human hair using liquid chromatography-tandem mass spectrometry. J Pharm Biomed Anal 2012; 67-68:154-158
- Segura J, Pichini S, Peng SH, de la Torre X. Hair analysis and detectability of single dose administration of androgenic steroid esters. Forensic Sci Int 2000; 107:347-359
- Brown HG, Perrett D. Detection of doping in sport: detecting anabolic-androgenic steroids in human fingernail clippings. The Medico-legal journal 2011; 79:67-69
- Anizan S, Huestis MA. The potential role of oral fluid in antidoping testing. Clin Chem 2014; 60:307-322
- Schonfelder M, Hofmann H, Schulz T, Engl T, Kemper D, Mayr B, Rautenberg C, Oberhoffer R, Thieme D. Potential detection of low-dose transdermal testosterone administration in blood, urine, and saliva. Drug Test Anal 2016; 8:1186-1196
- Chiodini I, Ramos-Rivera A, Marcus AO, Yau H. Adrenal Hypercortisolism: A Closer Look at Screening, Diagnosis, and Important Considerations of Different Testing Modalities. J Endocr Soc 2019; 3:1097-1109
- Welker KM, Lassetter B, Brandes CM, Prasad S, Koop DR, Mehta PH. A comparison of salivary testosterone measurement using immunoassays and tandem mass spectrometry. Psychoneuroendocrinology 2016; 71:180-188
- Thevis M, Krug O, Geyer H, Schanzer W. Expanding analytical options in sports drug testing: Mass spectrometric detection of prohibited substances in exhaled breath. Rapid Commun Mass Spectrom 2017; 31:1290-1296
- Schonfelder M, Hofmann H, Anielski P, Thieme D, Oberhoffer R, Michna H. Gene expression profiling in human whole blood samples after controlled testosterone application and exercise. Drug Test Anal 2011; 3:652-660
- Woldemariam GA, Butch AW. Immunoextraction-tandem mass spectrometry method for measuring intact human chorionic gonadotropin, free beta-subunit, and beta-subunit core fragment in urine. Clin Chem 2014; 60:1089-1097
- Butch AW, Woldemariam GA. Urinary human chorionic gonadotropin isoform concentrations in doping control samples. Drug Test Anal 2016; 8:1147-1151
- Butch AW, Ahrens BD, Avliyakulov NK. Urine reference intervals for human chorionic gonadotropin (hCG) isoforms by immunoextraction-tandem mass spectrometry to detect hCG use. Drug Test Anal 2018; 10:956-960
- Handelsman DJ, Goebel C, Idan A, Jimenez M, Trout G, Kazlauskas R. Effects of recombinant human LH and hCG on serum and urine LH and androgens in men. Clinical Endocrinology 2009; 71:417-428
- Handelsman DJ, Idan A, Grainger J, Goebel C, Turner L, Conway AJ. Detection and effects on serum and urine steroid and LH of repeated GnRH analog (leuprolide) stimulation. J Steroid Biochem Mol Biol 2014; 141:113-120
- Graham MR, Ryan P, Baker JS, Davies B, Thomas NE, Cooper SM, Evans P, Easmon S, Walker CJ, Cowan D, Kicman AT. Counterfeiting in performance- and image-enhancing drugs. Drug Test Anal 2009; 1:135-142
- Elliott S. Erythropoiesis-stimulating agents and other methods to enhance oxygen transport. Br J Pharmacol 2008; 154:529-541
- Reichel C, Gmeiner G. Erythropoietin and analogs. Handb Exp Pharmacol 2010:251-294
- di Prampero PE, Ferretti G. Factors limiting maximal oxygen consumption in humans. Respiration physiology 1990; 80:113-127
- Pace N, Consolazio WV, Lozner EL. The effect of transfusions of red blood cells on the hypoxia tolerance of normal men. Science 1945; 102:589-591
- Pace N, Lozner EL, et al. The increase in hypoxia tolerance of normal men accompanying the polycythemia induced by transfusion of erythrocytes. Am J Physiol 1947; 148:152-163
- Ekblom B, Goldbarg AN, Gullbring B. Response to exercise after blood loss and reinfusion. J Appl Physiol 1972; 33:175-180
- Hoberman J. History and prevalence of doping in the marathon. Sports Med 2007; 37:386-388
- Birkeland KI, Stray-Gundersen J, Hemmersbach P, Hallen J, Haug E, Bahr R. Effect of rhEPO administration on serum levels of sTfR and cycling performance. Med Sci Sports Exerc 2000; 32:1238-1243
- Ashenden MJ, Hahn AG, Martin DT, Logan P, Parisotto R, Gore CJ. A comparison of the physiological response to simulated altitude exposure and r-HuEpo administration. J Sports Sci 2001; 19:831-837
- Russell G, Gore CJ, Ashenden MJ, Parisotto R, Hahn AG. Effects of prolonged low doses of recombinant human erythropoietin during submaximal and maximal exercise. Eur J Appl Physiol 2002; 86:442-449
- Nelson M, Popp H, Sharpe K, Ashenden M. Proof of homologous blood transfusion through quantification of blood group antigens. Haematologica 2003; 88:1284-1295
- Cartron JP, Colin Y. Structural and functional diversity of blood group antigens. Transfusion clinique et biologique : journal de la Societe francaise de transfusion sanguine 2001; 8:163-199
- Giraud S, Robinson N, Mangin P, Saugy M. Scientific and forensic standards for homologous blood transfusion anti-doping analyses. Forensic Sci Int 2008; 179:23-33
- Voss SC, Thevis M, Schinkothe T, Schanzer W. Detection of homologous blood transfusion. Int J Sports Med 2007; 28:633-637
- Manokhina I, Rupert JL. A DNA-based method for detecting homologous blood doping. Anal Bioanal Chem 2013;
- Stampella A, Di Marco S, Pirri D, de la Torre X, Botre F, Donati F. Application of DNA-based forensic analysis for the detection of homologous transfusion of whole blood and of red blood cell concentrates in doping control. Forensic Sci Int 2016; 265:204-210
- Giraud S, Sottas PE, Robinson N, Saugy M. Blood transfusion in sports. Handb Exp Pharmacol 2010:295-304
- Segura J, Monfort N, Ventura R. Detection methods for autologous blood doping. Drug Test Anal 2012; 4:876-881
- Donati F, Acciarini R, De Benedittis I, de la Torre X, Pirri D, Prete M, Stampella A, Vernucci E, Botre F. Detecting Autologous Blood Transfusion in Doping Control: Biomarkers of Blood Aging and Storage Measured by Flow Cytofluorimetry. Curr Pharm Biotechnol 2018; 19:124-135
- Doctor A, Spinella P. Effect of processing and storage on red blood cell function in vivo. Seminars in perinatology 2012; 36:248-259
- Monfort N, Ventura R, Balcells G, Segura J. Determination of five di-(2-ethylhexyl)phthalate metabolites in urine by UPLC-MS/MS, markers of blood transfusion misuse in sports. J Chromatogr B Analyt Technol Biomed Life Sci 2012; 908:113-121
- Pottgiesser T, Umhau M, Ahlgrim C, Ruthardt S, Roecker K, Schumacher YO. Hb mass measurement suitable to screen for illicit autologous blood transfusions. Med Sci Sports Exerc 2007; 39:1748-1756
- Morkeberg J, Sharpe K, Belhage B, Damsgaard R, Schmidt W, Prommer N, Gore CJ, Ashenden MJ. Detecting autologous blood transfusions: a comparison of three passport approaches and four blood markers. Scandinavian journal of medicine & science in sports 2011; 21:235-243
- Pottgiesser T, Echteler T, Sottas PE, Umhau M, Schumacher YO. Hemoglobin mass and biological passport for the detection of autologous blood doping. Med Sci Sports Exerc 2012; 44:835-843
- Ashenden M, Gough CE, Garnham A, Gore CJ, Sharpe K. Current markers of the Athlete Blood Passport do not flag microdose EPO doping. Eur J Appl Physiol 2011; 111:2307-2314
- Kannan M, Atreya C. Differential profiling of human red blood cells during storage for 52 selected microRNAs. Transfusion 2010; 50:1581-1588
- Pottgiesser T, Schumacher YO, Funke H, Rennert K, Baumstark MW, Neunuebel K, Mosig S. Gene expression in the detection of autologous blood transfusion in sports--a pilot study. Vox sanguinis 2009; 96:333-336
- Sottas PE, Robinson N, Saugy M. The athlete's biological passport and indirect markers of blood doping. Handb Exp Pharmacol 2010:305-326
- Sottas PE, Robinson N, Rabin O, Saugy M. The athlete biological passport. Clin Chem 2011; 57:969-976
- Pottgiesser T, Schumacher YO. Current strategies of blood doping detection. Anal Bioanal Chem 2013;
- Cazzola M. A global strategy for prevention and detection of blood doping with erythropoietin and related drugs. Haematologica 2000; 85:561-563
- Parisotto R, Gore CJ, Emslie KR, Ashenden MJ, Brugnara C, Howe C, Martin DT, Trout GJ, Hahn AG. A novel method utilising markers of altered erythropoiesis for the detection of recombinant human erythropoietin abuse in athletes. Haematologica 2000; 85:564-572
- Parisotto R, Wu M, Ashenden MJ, Emslie KR, Gore CJ, Howe C, Kazlauskas R, Sharpe K, Trout GJ, Xie M. Detection of recombinant human erythropoietin abuse in athletes utilizing markers of altered erythropoiesis. Haematologica 2001; 86:128-137
- Gore CJ, Parisotto R, Ashenden MJ, Stray-Gundersen J, Sharpe K, Hopkins W, Emslie KR, Howe C, Trout GJ, Kazlauskas R, Hahn AG. Second-generation blood tests to detect erythropoietin abuse by athletes. Haematologica 2003; 88:333-344
- Malcovati L, Pascutto C, Cazzola M. Hematologic passport for athletes competing in endurance sports: a feasibility study. Haematologica 2003; 88:570-581
- Sharpe K, Ashenden MJ, Schumacher YO. A third generation approach to detect erythropoietin abuse in athletes. Haematologica 2006; 91:356-363
- Sottas PE, Robinson N, Giraud S, Tarioni F, Kamber M, Mangin P, Saugy M. Statistical classificiation of abnormal blood profiles inathletes. International Journal of Biostatistics 2006; 2:3
- WADA. Athlete Biological Passport: Operating guidelines and compilation of required elements. Verson 3.1. World Anti-Doping Agency;2012.
- Ekblom B, Berglund B. Effect of erythropoeitin administration on maximal aerobic power in man. Scandanavian Journal of Medicine and Science in Sport 1991; 1:88-93
- Jelkmann W. Biosimilar recombinant human erythropoietins ("epoetins") and future erythropoiesis-stimulating treatments. Expert Opin Biol Ther 2012; 12:581-592
- Catlin DH, Fitch KD, Ljungqvist A. Medicine and science in the fight against doping in sport. J Intern Med 2008; 264:99-114
- Lopez B. The invention of a 'drug of mass destruction': deconstructing the EPO myth. Sport in History 2011; 31:84-109
- Lasne F, de Ceaurriz J. Recombinant erythropoietin in urine. Nature 2000; 405:635
- Lasne F, Martin L, Crepin N, de Ceaurriz J. Detection of isoelectric profiles of erythropoietin in urine: differentiation of natural and administered recombinant hormones. Anal Biochem 2002; 311:119-126
- Lasne F, Thioulouse J, Martin L, de Ceaurriz J. Detection of recombinant human erythropoietin in urine for doping analysis: interpretation of isoelectric profiles by discriminant analysis. Electrophoresis 2007; 28:1875-1881
- Leuenberger N, Reichel C, Lasne F. Detection of erythropoiesis-stimulating agents in human anti-doping control: past, present and future. Bioanalysis 2012; 4:1565-1575
- Breidbach A, Catlin DH, Green GA, Tregub I, Truong H, Gorzek J. Detection of recombinant human erythropoietin in urine by isoelectric focusing. Clin Chem 2003; 49:901-907
- Wickramasinghe S, Medrano JF. Primer on genes encoding enzymes in sialic acid metabolism in mammals. Biochimie 2011; 93:1641-1646
- Reverter-Branchat G, Ventura R, Ezzel Din M, Mateus J, Pedro C, Segura J. Detection of erythropoiesis-stimulating agents in a single dried blood spot. Drug Test Anal 2018; 10:1496-1507
- Durussel J, Haile DW, Mooses K, Daskalaki E, Beattie W, Mooses M, Mekonen W, Ongaro N, Anjila E, Patel RK, Padmanabhan N, McBride MW, McClure JD, Pitsiladis YP. Blood transcriptional signature of recombinant human erythropoietin administration and implications for antidoping strategies. Physiol Genomics 2016; 48:202-209
- Beuck S, Schanzer W, Thevis M. Hypoxia-inducible factor stabilizers and other small-molecule erythropoiesis-stimulating agents in current and preventive doping analysis. Drug Test Anal 2012; 4:830-845
- La Merie Publishing. Blockbuster Biologics 2012. La Merie Business Intlligence;2013.
- Imagawa S, Matsumoto K, Horie M, Ohkoshi N, Nagasawa T, Doi T, Suzuki N, Yamamoto M. Does k-11706 enhance performance and why? Int J Sports Med 2007; 28:928-933
- Richardson RS, Tagore K, Haseler LJ, Jordan M, Wagner PD. Increased VO2 max with right-shifted Hb-O2 dissociation curve at a constant O2 delivery in dog muscle in situ. Journal of applied physiology (Bethesda, Md : 1985) 1998; 84:995-1002
- Pagel PS, Hettrick DA, Montgomery MW, Kersten JR, Steffen RP, Warltier DC. RSR13, a synthetic modifier of hemoglobin-oxygen affinity, enhances the recovery of stunned myocardium in anesthetized dogs. J Pharmacol Exp Ther 1998; 285:1-8
- Breidbach A, Catlin DH. RSR13, a potential athletic performance enhancement agent: detection in urine by gas chromatography/mass spectrometry. Rapid Commun Mass Spectrom 2001; 15:2379-2382
- Thevis M, Krug O, Schanzer W. Mass spectrometric characterization of efaproxiral (RSR13) and its implementation into doping controls using liquid chromatography-atmospheric pressure ionization-tandem mass spectrometry. J Mass Spectrom 2006; 41:332-338
- Casadevall N, Nataf J, Viron B, Kolta A, Kiladjian JJ, Martin-Dupont P, Michaud P, Papo T, Ugo V, Teyssandier I, Varet B, Mayeux P. Pure red-cell aplasia and antierythropoietin antibodies in patients treated with recombinant erythropoietin. N Engl J Med 2002; 346:469-475
- Macdougall IC, Roger SD, de Francisco A, Goldsmith DJ, Schellekens H, Ebbers H, Jelkmann W, London G, Casadevall N, Horl WH, Kemeny DM, Pollock C. Antibody-mediated pure red cell aplasia in chronic kidney disease patients receiving erythropoiesis-stimulating agents: new insights. Kidney Int 2012; 81:727-732
- Unger EF, Thompson AM, Blank MJ, Temple R. Erythropoiesis-stimulating agents--time for a reevaluation. N Engl J Med 2010; 362:189-192
- Bennett CL, Silver SM, Djulbegovic B, Samaras AT, Blau CA, Gleason KJ, Barnato SE, Elverman KM, Courtney DM, McKoy JM, Edwards BJ, Tigue CC, Raisch DW, Yarnold PR, Dorr DA, Kuzel TM, Tallman MS, Trifilio SM, West DP, Lai SY, Henke M. Venous thromboembolism and mortality associated with recombinant erythropoietin and darbepoetin administration for the treatment of cancer-associated anemia. JAMA 2008; 299:914-924
- Strippoli GF, Navaneethan SD, Craig JC. Haemoglobin and haematocrit targets for the anaemia of chronic kidney disease. Cochrane Database Syst Rev 2006:CD003967
- Tonia T, Mettler A, Robert N, Schwarzer G, Seidenfeld J, Weingart O, Hyde C, Engert A, Bohlius J. Erythropoietin or darbepoetin for patients with cancer. Cochrane Database Syst Rev 2012; 12:CD003407
- Rampling MW. Hyperviscosity as a complication in a variety of disorders. Semin Thromb Hemost 2003; 29:459-465
- Kovesdy CP, Kalantar-Zadeh K. Iron therapy in chronic kidney disease: current controversies. Journal of renal care 2009; 35 Suppl 2:14-24
- Gagnon DR, Zhang TJ, Brand FN, Kannel WB. Hematocrit and the risk of cardiovascular disease--the Framingham study: a 34-year follow-up. Am Heart J 1994; 127:674-682
- Danesh J, Collins R, Peto R, Lowe GD. Haematocrit, viscosity, erythrocyte sedimentation rate: meta-analyses of prospective studies of coronary heart disease. Eur Heart J 2000; 21:515-520
- Braekkan SK, Mathiesen EB, Njolstad I, Wilsgaard T, Hansen JB. Hematocrit and risk of venous thromboembolism in a general population. The Tromso study. Haematologica 2010; 95:270-275
- Holt RI, Erotokritou-Mulligan I, Sonksen PH. The history of doping and growth hormone abuse in sport. Growth Horm IGF Res 2009; 19:320-326
- Liu H, Bravata DM, Olkin I, Friedlander A, Liu V, Roberts B, Bendavid E, Saynina O, Salpeter SR, Garber AM, Hoffman AR. Systematic review: the effects of growth hormone on athletic performance. Ann Intern Med 2008; 148:747-758
- Baumann GP. Growth hormone doping in sports: a critical review of use and detection strategies. Endocr Rev 2012; 33:155-186
- Holt RIG, Ho KKY. The Use and Abuse of Growth Hormone in Sports. Endocr Rev 2019; 40:1163-1185
- Meinhardt U, Nelson AE, Hansen JL, Birzniece V, Clifford D, Leung KC, Graham K, Ho KK. The effects of growth hormone on body composition and physical performance in recreational athletes: a randomized trial. Ann Intern Med 2010; 152:568-577
- Berggren A, Ehrnborg C, Rosen T, Ellegard L, Bengtsson BA, Caidahl K. Short-term administration of supraphysiological recombinant human growth hormone (GH) does not increase maximum endurance exercise capacity in healthy, active young men and women with normal GH-insulin-like growth factor I axes. J Clin Endocrinol Metab 2005; 90:3268-3273
- Irving BA, Patrie JT, Anderson SM, Watson-Winfield DD, Frick KI, Evans WS, Veldhuis JD, Weltman A. The effects of time following acute growth hormone administration on metabolic and power output measures during acute exercise. J Clin Endocrinol Metab 2004; 89:4298-4305
- Graham MR, Baker JS, Evans P, Kicman A, Cowan D, Hullin D, Davies B. Short-term recombinant human growth hormone administration improves respiratory function in abstinent anabolic-androgenic steroid users. Growth Horm IGF Res 2007; 17:328-335
- Goto K, Doessing S, Nielsen RH, Flyvbjerg A, Kjaer M. Growth hormone receptor antagonist treatment reduces exercise performance in young males. J Clin Endocrinol Metab 2009; 94:3265-3272
- Healy ML, Gibney J, Russell-Jones DL, Pentecost C, Croos P, Sonksen PH, Umpleby AM. High dose growth hormone exerts an anabolic effect at rest and during exercise in endurance-trained athletes. J Clin Endocrinol Metab 2003; 88:5221-5226
- Healy ML, Gibney J, Pentecost C, Croos P, Russell-Jones DL, Sonksen PH, Umpleby AM. Effects of high-dose growth hormone on glucose and glycerol metabolism at rest and during exercise in endurance-trained athletes. J Clin Endocrinol Metab 2006; 91:320-327
- Handelsman DJ, Hirschberg AL, Bermon S. Circulating Testosterone as the Hormonal Basis of Sex Differences in Athletic Performance. Endocr Rev 2018; 39:803-829
- Breederveld RS, Tuinebreijer WE. Recombinant human growth hormone for treating burns and donor sites. Cochrane Database Syst Rev 2012; 12:CD008990
- Takala J, Ruokonen E, Webster NR, Nielsen MS, Zandstra DF, Vundelinckx G, Hinds CJ. Increased mortality associated with growth hormone treatment in critically ill adults N Engl J Med 1999; 341:785-792
- Raschke M, Rasmussen MH, Govender S, Segal D, Suntum M, Christiansen JS. Effects of growth hormone in patients with tibial fracture: a randomised, double-blind, placebo-controlled clinical trial. Eur J Endocrinol 2007; 156:341-351
- van den Berg G, Frolich M, Veldhuis JD, Roelfsema F. Growth hormone secretion in recently operated acromegalic patients. J Clin Endocrinol Metab 1994; 79:1706-1715
- Fuchtbauer L, Olsson DS, Bengtsson BA, Norrman LL, Sunnerhagen KS, Johannsson G. Muscle strength in patients with acromegaly at diagnosis and during long-term follow-up. Eur J Endocrinol 2017; 177:217-226
- Sklar CA, Mertens AC, Mitby P, Occhiogrosso G, Qin J, Heller G, Yasui Y, Robison LL. Risk of disease recurrence and second neoplasms in survivors of childhood cancer treated with growth hormone: a report from the Childhood Cancer Survivor Study. J Clin Endocrinol Metab 2002; 87:3136-3141
- Ergun-Longmire B, Mertens AC, Mitby P, Qin J, Heller G, Shi W, Yasui Y, Robison LL, Sklar CA. Growth hormone treatment and risk of second neoplasms in the childhood cancer survivor. J Clin Endocrinol Metab 2006; 91:3494-3498
- Carel JC, Ecosse E, Landier F, Meguellati-Hakkas D, Kaguelidou F, Rey G, Coste J. Long-term mortality after recombinant growth hormone treatment for isolated growth hormone deficiency or childhood short stature: preliminary report of the French SAGhE study. J Clin Endocrinol Metab 2012; 97:416-425
- Mo D, Hardin DS, Erfurth EM, Melmed S. Adult mortality or morbidity is not increased in childhood-onset growth hormone deficient patients who received pediatric GH treatment: an analysis of the Hypopituitary Control and Complications Study (HypoCCS). Pituitary 2013;
- Swerdlow AJ, Higgins CD, Adlard P, Preece MA. Risk of cancer in patients treated with human pituitary growth hormone in the UK, 1959-85: a cohort study. Lancet 2002; 360:273-277
- Wilton P, Mattsson AF, Darendeliler F. Growth hormone treatment in children is not associated with an increase in the incidence of cancer: experience from KIGS (Pfizer International Growth Database). J Pediatr 2010; 157:265-270
- Mackenzie S, Craven T, Gattamaneni HR, Swindell R, Shalet SM, Brabant G. Long-term safety of growth hormone replacement after CNS irradiation. J Clin Endocrinol Metab 2011; 96:2756-2761
- Woodmansee WW, Zimmermann AG, Child CJ, Rong Q, Erfurth EM, Beck-Peccoz P, Blum WF, Robison LL. Incidence of second neoplasm in childhood cancer survivors treated with GH: an analysis of GeNeSIS and HypoCCS. Eur J Endocrinol 2013; 168:565-573
- Jenkins PJ, Mukherjee A, Shalet SM. Does growth hormone cause cancer? Clin Endocrinol (Oxf) 2006; 64:115-121
- Holly J, Perks C. Growth hormone and cancer: are we asking the right questions?*. Clin Endocrinol (Oxf) 2006; 64:122-124
- Ho KK, Nelson AE. Growth hormone in sports: detecting the doped or duped. Hormone research in paediatrics 2011; 76 Suppl 1:84-90
- Hepner F, Cszasar E, Roitinger E, Lubec G. Mass spectrometrical analysis of recombinant human growth hormone (Genotropin(R)) reveals amino acid substitutions in 2% of the expressed protein. Proteome science 2005; 3:1
- Hepner F, Csaszar E, Roitinger E, Pollak A, Lubec G. Mass spectrometrical analysis of recombinant human growth hormone Norditropin reveals amino acid exchange at M14_V14 rhGH. Proteomics 2006; 6:775-784
- Jiang H, Wu SL, Karger BL, Hancock WS. Mass spectrometric analysis of innovator, counterfeit, and follow-on recombinant human growth hormone. Biotechnology progress 2009; 25:207-218
- Bidlingmaier M, Strasburger CJ. Growth hormone. Handb Exp Pharmacol 2010:187-200
- Bosch J, Luchini A, Pichini S, Tamburro D, Fredolini C, Liotta L, Petricoin E, Pacifici R, Facchiano F, Segura J, Garaci E, Gutierrez-Gallego R. Analysis of urinary human growth hormone (hGH) using hydrogel nanoparticles and isoform differential immunoassays after short recombinant hGH treatment: preliminary results. J Pharm Biomed Anal 2013; 85:194-197
- Bidlingmaier M, Suhr J, Ernst A, Wu Z, Keller A, Strasburger CJ, Bergmann A. High-sensitivity chemiluminescence immunoassays for detection of growth hormone doping in sports. Clin Chem 2009; 55:445-453
- Bidlingmaier M, Manolopoulou J. Detecting growth hormone abuse in athletes. Endocrinol Metab Clin North Am 2010; 39:25-32, vii
- Marks V. Murder by insulin: suspected, purported and proven-a review. Drug Test Anal 2009; 1:162-176
- Will RG. Acquired prion disease: iatrogenic CJD, variant CJD, kuru. Br Med Bull 2003; 66:255-265
- Brown P, Preece M, Brandel JP, Sato T, McShane L, Zerr I, Fletcher A, Will RG, Pocchiari M, Cashman NR, d'Aignaux JH, Cervenakova L, Fradkin J, Schonberger LB, Collins SJ. Iatrogenic Creutzfeldt-Jakob disease at the millennium. Neurology 2000; 55:1075-1081
- Barroso O, Schamasch P, Rabin O. Detection of GH abuse in sport: Past, present and future. Growth Horm IGF Res 2009; 19:369-374
- Travis J. Pharmacology. Growth hormone test finally nabs first doper. Science 2010; 327:1185
- Powrie JK, Bassett EE, Rosen T, Jorgensen JO, Napoli R, Sacca L, Christiansen JS, Bengtsson BA, Sonksen PH, Group GHPS. Detection of growth hormone abuse in sport. Growth Horm IGF Res 2007; 17:220-226
- Nelson AE, Meinhardt U, Hansen JL, Walker IH, Stone G, Howe CJ, Leung KC, Seibel MJ, Baxter RC, Handelsman DJ, Kazlauskas R, Ho KK. Pharmacodynamics of growth hormone abuse biomarkers and the influence of gender and testosterone: a randomized double-blind placebo-controlled study in young recreational athletes. Journal of Clinical Endocrinology and Metabolism 2008; 93:2213-2222
- Krusenstjerna-Hafstrom T, Rasmussen MH, Raschke M, Govender S, Madsen J, Christiansen JS. Biochemical markers of bone turnover in tibia fracture patients randomly assigned to growth hormone (GH) or placebo injections: Implications for detection of GH abuse. Growth Horm IGF Res 2011; 21:331-335
- Barroso O, Handelsman DJ, Strasburger C, Thevis M. Analytical challenges in the detection of peptide hormones for anti-doping purposes. Bioanalysis 2012; 4:1577-1590
- Williams RM, McDonald A, Savage MO, Dunger DB. Mecasermin rinfabate: rhIGF-I/rhIGFBP-3 complex: iPLEX. Expert Opin Drug Metab Toxicol 2008; 4:311-324
- Guha N, Dashwood A, Thomas NJ, Skingle AJ, Sonksen PH, Holt RI. IGF-I abuse in sport. Current drug abuse reviews 2009; 2:263-272
- Ernst S, Simon P. A quantitative approach for assessing significant improvements in elite sprint performance: has IGF-1 entered the arena? Drug Test Anal 2013; 5:384-389
- Thomas A, Kohler M, Schanzer W, Delahaut P, Thevis M. Determination of IGF-1 and IGF-2, their degradation products and synthetic analogues in urine by LC-MS/MS. Analyst 2011; 136:1003-1012
- Hess C, Thomas A, Thevis M, Stratmann B, Quester W, Tschoepe D, Madea B, Musshoff F. Simultaneous determination and validated quantification of human insulin and its synthetic analogues in human blood serum by immunoaffinity purification and liquid chromatography-mass spectrometry. Anal Bioanal Chem 2012; 404:1813-1822
- Guha N, Erotokritou-Mulligan I, Nevitt SP, Francis M, Bartlett C, Cowan DA, Bassett EE, Sonksen PH, Holt RI. Biochemical markers of recombinant human insulin-like growth factor-I (rhIGF-I)/rhIGF binding protein-3 (rhIGFBP-3) misuse in athletes. Drug Test Anal 2013;
- Guha N, Cowan DA, Sonksen PH, Holt RI. Insulin-like growth factor-I (IGF-I) misuse in athletes and potential methods for detection. Anal Bioanal Chem 2013; 405:9669-9683
- Matheny RW, Jr., Nindl BC, Adamo ML. Minireview: Mechano-growth factor: a putative product of IGF-I gene expression involved in tissue repair and regeneration. Endocrinology 2010; 151:865-875
- Esposito S, Deventer K, Van Eenoo P. Characterization and identification of a C-terminal amidated mechano growth factor (MGF) analogue in black market products. Rapid Commun Mass Spectrom 2012; 26:686-692
- Thevis M, Thomas A, Schanzer W. Insulin. Handb Exp Pharmacol 2010:209-226
- Anderson LJ, Tamayose JM, Garcia JM. Use of growth hormone, IGF-I, and insulin for anabolic purpose: Pharmacological basis, methods of detection, and adverse effects. Mol Cell Endocrinol 2018; 464:65-74
- Thomas A, Thevis M. Recent advances in the determination of insulins from biological fluids. Adv Clin Chem 2019; 93:115-167
- Smith RG. Development of growth hormone secretagogues. Endocr Rev 2005; 26:346-360
- Hersch EC, Merriam GR. Growth hormone (GH)-releasing hormone and GH secretagogues in normal aging: Fountain of Youth or Pool of Tantalus? Clin Interv Aging 2008; 3:121-129
- Thomas A, Walpurgis K, Krug O, Schanzer W, Thevis M. Determination of prohibited, small peptides in urine for sports drug testing by means of nano-liquid chromatography/benchtop quadrupole orbitrap tandem-mass spectrometry. J Chromatogr A 2012; 1259:251-257
- Thomas A, Delahaut P, Krug O, Schanzer W, Thevis M. Metabolism of growth hormone releasing peptides. Anal Chem 2012; 84:10252-10259
- Thevis M, Kuuranne T, Geyer H. Annual banned-substance review: Analytical approaches in human sports drug testing. Drug Test Anal 2019; 11:8-26