ABSTRACT
Men continue to have a strong interest and commitment to effective family planning. Traditional methods of male contraception have long included periodic abstinence, non-vaginal ejaculation, condoms, and vasectomy, the latter two representing physical methods to prevent sperm from reaching the site of fertilization. However, for male contraception the reversible methods are not reliable, and the only reliable method, vasectomy, is not intended as reversible. During the 20th century, a wide array of reversible and highly reliable female hormonal contraceptive methods was marketed; however, no new methods for male fertility regulation have been introduced for centuries. For men to share more equally the burdens as well as the benefits of family planning, more effective reversible male contraceptive methods need to be available. Most studies into male contraception have been conducted with hormonal methods, analogous to well-known female hormonal contraceptives, making them the closest to introducing a reliable, reversible male contraceptive method. The most promising hormonal approach is the combination of an androgen (usually testosterone) with a progestin and multiple studies have shown such combinations of depot steroids displays high contraceptive efficacy, based on reliable and reversible suppression of sperm output, with few side effects. While research into novel methods for male fertility regulation has continued in the public sector, private sector research into male contraception, essential for effective commercial product development, has stalled in recent decades.
BACKGROUND
A male contraceptive must reduce the number of fertile sperm ejaculated into the vagina to levels that reliably prevent fertilization (1). Conception can be prevented by diverting or suppressing sperm output and/or inhibiting sperm fertilizing capacity. So far, all male methods depend on mechanical means to reduce female exposure to sperm using either traditional drug and device-free methods (abstinence, withdrawal, non-vaginal intercourse) or contemporary male methods comprising condoms and vasectomy. Unfortunately, among current male contraceptive options, the reversible methods are not reliable, and the reliable method is not intended as reversible. No new male contraceptive methods were introduced during the 20th century contrasting with highly reliable, reversible hormonal contraceptives developed for women over the last half century. Yet male involvement in family planning remains extensive. Globally, one third of couples using contraception rely on methods requiring active male participation (2) and current male methods remain (together with medicated intrauterine devices) the most cost-effective contraceptive methods in the USA (3). While reflecting traditional and ongoing reliance of family planning on male involvement, greater participation by men in sharing the burdens as well as the benefits of effective family planning require developing more effective, reversible male methods. Such greater participation could ameliorate the still large unmet need for contraception globally as 40% of pregnancies are still unintended with half of unintended pregnancies ending in abortion and those rates are higher among developed countries (4). Family planning must always remain a matter of personal choice by couples. This is highlighted by the human rights abuses of coercive national family planning programs enforcing family limitation (“one-child family”), including through forced sterilization and abortion, in India and China (5, 6). Such coercive national programs originated in the 1970’s prompted by uncritical belief in the “population explosion”, notably from influential publications such as the Club of Rome’s “Limits to Growth” and Ehrlich’s “Population Bomb”, now discredited by their failed, alarmist predictions. India’s forced sterilization program was overturned by popular democratic action within a few years of its imposition whereas China’s one-child family policy operated for nearly 4 decades before its ending in 2016. These issues echo in medical mistrust as a barrier to acceptance of male contraception among the Black American minority (7).
Extensive public sector clinical research over the last 4 decades (8), despite minimal pharmaceutical industry involvement, has proven in principle that hormonal male contraception is feasible. Proof-of-principle is established for androgen-based hormonal suppression of spermatogenesis (9) achieving full reversibility (10), sufficient short-term safety (11) and acceptability to men and women (12, 13) culminating in the crucial proof of reliable contraceptive efficacy using androgen alone (14-17) or depot (18) or daily use (19) androgen-progestin combinations. Demographic modelling shows that introducing new male contraceptive methods, even if adopted by only a minority of men, would significantly reduce unintended pregnancies in Nigeria, South Africa, and the USA (20). Surveys of participants in clinical male contraceptive studies suggest that men may prefer a male contraceptive to be provided by their family doctor rather than specialists (21); however, the practical knowledge of non-specialists remains unsatisfactory and would require specific training (22). In addition to boutique contraception for adventurous early adopters, practical niches for male contraception include contraception after birth, during breast-feeding, or after abortion (23). Nevertheless, despite the proof-of-principle, strong community interest, and medical priority on the need for new, reversible male contraceptives, pharmaceutical industry development has ceased. This market failure reflects pharmaceutical industry perceptions of low profitability coupled with high risk in the context where a better return on investment is expected for developing drugs in more lucrative, less risky areas of chronic disease compared with the higher product liability risk of developing novel contraceptives for healthy men to compete with existing low-cost female methods. The possibility that Western market failure might be overcome if the growing perception of needs for voluntary family planning as a national priority in rapidly industrializing countries such as China, India and Brazil (24), has proved a failed hope. Novel approaches to finance further research such as philanthropic private sector funding (25) and public-private partnerships (26) have been developed. The 2022 overturning by the US Supreme Court of what was long accepted as a constitutional right to abortion has refreshed interest in developing wider coverage of effective contraception, including for men, to reduce the demand for abortion.
NON-HORMONAL METHODS
Traditional Methods
PERIODIC ABSTINENCE
Although theoretically effective, neither celibacy nor castration is an acceptable or practical contraceptive method. Periodic abstinence, the limiting of sexual intercourse to "safe" days (27), has high contraceptive efficacy if the rules are followed perfectly but the failure rates rise steeply with rule breaking (28). This cost- and device-free method is used by >40 million couples world-wide for family planning (2). The typical use 1st year failure rate is ~25% with ~47% of couples continuing use at 1 year in USA (29) but 8% and 52%, respectively, in France (30, 31). While inherently safe, it has limited acceptability due to low reliability, inflexibility, and interference in the spontaneity of sex.
EX-VAGINAL EJACULATION
Withdrawal is a traditional male method of contraception whereby intercourse culminates in extra-vaginal ejaculation (32). Often overlooked as a contraceptive method, withdrawal, together with abortion, was the major pre-industrial method of family planning largely responsible for the demographic transition from high to relatively low birth rates in industrial nation states and continues to be used by 40 million couples (2). This cost- and device-free method has limited reliability in its demanding requirement for skill and self-control. The typical use 1st year failure rate is 18-22% with 46% continuing use at 1 year in the USA (29, 33) and 10% and 55%, respectively, in France (30, 31). While safe and reasonably effective for experienced users, interfering with the pleasure of coitus leads to a correspondingly high failure rate in practice. Other sexual practices that avoid intravaginal ejaculation have also been used traditionally to avoid conception. These include masturbation, oral and anal intercourse, deliberate anejaculation and retrograde ejaculation (34).
CONDOM
After centuries of use in preventing sexually transmitted infections and pregnancy, now over 50 million couples rely on condoms for contraception (2). Condoms provide safe, cheap, widely available, user-controlled, and reversible contraception with few side-effects. In case of latex allergy, non-rubber (polyurethane, natural membrane) condoms can be substituted. Latex condoms are moderately effective at preventing pregnancy with a typical 1st year failure rate of 18% with 43% continuing use at 1 year in the USA (29, 33) and 3.3% and 47%, respectively, in France (30, 31). Recent US data suggest a reduction in failure rate to 13% (35). The discrepancy from the estimated 2% perfect-use failure rate (29) is mainly to human error, notably rushed use (36), misuse, or non-use rather than mechanical failure (breakage or slippage) (37). Differences in contraceptive use behaviors may explain lower reported 1st year failure rates for condoms in France (31). The major limitations of condoms for contraception are relatively high failure rates and interference with sexuality. The requirement for regular and correct application during foreplay disturbs the spontaneity of sex and dulls erotic sensation. These aesthetic drawbacks limit the popularity of condoms especially among stable couples (38). Latex condoms are perishable through tears or snagging on fingernails, clothing, or jewelry as well as deterioration from exposure to light, heat, humidity, or organic oils.
Polyurethane condoms with improved tactile sensitivity were developed in the 1990’s to enhance acceptability (39), but they have shown reduced efficacy and mechanical performance compared with latex condoms in prospective randomized controlled clinical trials (40). Although the theoretical requirements for condom use to protect against sexually transmitted infections differ from those to prevent pregnancy, in practice the protections are similar (41). Laboratory testing of condoms standardizes integrity and durability for strength and leakage. Although viral penetration is not routinely tested, synthetic (latex or polyurethane) but not natural membrane condoms are not perfect at preventing passage of prototype human pathogenic viruses (42). Using a sensitive, objective biochemical marker (PSA) for seminal plasma exposure in vaginal swabs, vaginal exposure to semen was reduced by 50-80% even after mechanical condom failure (breakage, slippage) (43). Novel spermicides with virucidal properties that are being developed to provide dual antimicrobial and contraceptive protection (44) might not enhance condom efficacy for either function because non-compliance is the major cause of failure for both (37).
VASECTOMY
Vasectomy, used by over 40 million couples for family planning (2), varies widely in prevalence between countries depending upon cultural factors, public education, and availability of male-oriented facilities (45). Recent data suggests a steady decline in vasectomy in the US over the first two decades of the 21st century (46). Having overcome a sordid history of applications to eugenics and other historical misadventures into the 20th century (47), vasectomy is now more widely used than female sterilization in some economically developed countries although female sterilization remain ~4 times more frequent worldwide (48). For men having completed their family and fit for minor surgery, vasectomy is a very safe and highly effective office procedure (49, 50). Relative contraindications include risks from office-type surgery (bleeding disorders, allergy to local anesthetic) or scrotal pathology (post-inguinal surgery scarring, keloid-proneness, active genitourinary or groin infections). Vasectomy is performed under local anesthesia via scrotal incisions and usually involves excising a segment of both vasa deferentia. Interposing a fascial barrier between the occluded cut ends significantly reduces the risk of failure due to recanalization (51, 52). The Chinese-developed "no-scalpel" technique (53)minimizes skin incision and reduces immediate side-effects (bleeding, infection) 10-fold to 0.3% compared with conventional vasectomy (54, 55). Chronic post-vasectomy pain (56) occurs with an estimated prevalence of ~15% at 7 months after vasectomy (57). It may be reported as groin or testicular pain precipitated or aggravated by intercourse, ejaculation, or exertion and accompanied by tender, distended epididymides (56, 57). Fascial interposition significantly reduces failure rate (52, 58) so that, in the hands of an experienced practitioner, no-scalpel vasectomy with fascial interposition is now the method of choice (55, 59). Additional studies suggest that cautery may further enhances reliability (60, 61) and that leaving an open testicular end reduces retrograde pressure-related complications (pain, sperm granuloma, epididymal and testicular damage) thereby better preserving reversibility (62-64).
Vasectomy is highly effective once sperm are cleared from the distal vasa deferentia. However, flushing with saline or water (65-69) or spermicides (nitrofurazone (70), euflavine (71, 72) or chlorhexidine (73)) during surgery does not accelerate sperm clearance, but the evidence remains weak (74). Non-irritant spermicides that inhibit sperm function without chemical sclerosis of the vasa that would impair potential reversibility, might have promise (75). Immediately following vasectomy, additional contraception must continue until azoospermia or near azoospermia (<0.1 million non-motile sperm per mL) is demonstrated usually at 3 months post-vasectomy when at least 95% of men reach this clearance criterion (76-79). Although azoospermia might occur sooner (80), reliable evidence is lacking to support the reliability of earlier time points as recanalization, where motile sperm persist in the ejaculate, may occur within the first few weeks after vasectomy (81) and persistence of motile sperm in the ejaculate indicates technical failure. Although detailed information on the rate of sperm clearance from the ejaculate after vasectomy remains sparse, time since vasectomy rather than number of ejaculations is more predictive of sperm clearance (80). Contraceptive failures are rare; early failures are most often due to not awaiting sperm clearance (82) or occasionally misidentification or duplication of the vasa deferentia whereas late failures are due to spontaneous vas(a) recanalization (~0.1%) (83). Complications of vasectomy include post-surgical bleeding, wound or genito-urinary infections and fistulae, and chronic scrotal pain (50, 84) with risk of death estimated at ~1 per million vasectomies in developed countries although higher in developing countries (85). Vasectomy causes no consistent changes in circulating hormones (86), sexual function, or risk of cardiovascular or other diseases (49, 87, 88) including testicular cancer (89-91). A small increased risk of prostate cancer after vasectomy has been observed in case-control studies (92-94), but the increased risk is unaffected by vasectomy reversal (95, 96). This risk seems to be attributable to surveillance and detection bias, rather than a biological effect as indicated by consistent evidence from numerous cohort studies (96-102). Sperm antibodies develop in most vasectomized men but have no known deleterious health effects apart from a possible role in reducing fertility after vasectomy reversal (103) when sperm antibody titers are very high (>512) (104).
Vasectomy is a quick, simple, highly effective, and convenient method of permanent sterilization; its major drawback as a male contraceptive is its limited reversibility. Elective sperm cryostorage is occasionally useful but may reflect ambivalence about the irreversible intent of vasectomy. Cumulative rate of requests for reversal, mostly prompted by remarriage, are 2.4% at 10 year post-vasectomy but exceed 10% for young men (aged <25 years at vasectomy) (105). Failed vasectomy reversal is now a significant cause of male infertility. Following microsurgical vasovasostomy, 80-100% have any sperm in the ejaculate ("patency") but normal sperm concentration is less common, and cumulative conception rate at 12 months is only ~50% compared to 80-85% at 12 months in healthy young couples not using contraception (105). This discrepancy is most probably attributable to technical limitations of microsurgery as even the lowest reported rates of azoospermia (bilateral non-patency) after microsurgical vasovasostomy indicate that nearly half such men have at least one non-patent vas deferens (106) so that re-operation should be a prominent consideration if pregnancy does not ensue. After technical failure, the wife’s age and time since vasectomy appear to be the dominant predictors of successful reversal (107, 108) Whether robotic microsurgery can improve the technical success of vasovasostomy to become a cost-effective and widely available alternative to human microsurgery remains to be established (109-111). Reversibility is better with microsurgery (106), presence of sperm in the vasal fluids from testicular end of stump (112), in younger men with shorter duration since vasectomy (105) and possibly with longer testicular vasal stump (113, 114); unfavorable predictors include non-microsurgical techniques, older age of wife (especially after 40 yr) (106, 115), high titers of sperm antibodies (104), and long duration (>10 years) since vasectomy (116-118) due to long-term epididymal (119), vasal (120) and testicular damage (116, 117, 121, 122). Experimentally in a variety of mammalian species, open-ended vasectomy is preferable to complete vas occlusion in preventing the back pressure-induced damage to spermatogenesis and seminiferous tubular integrity which are important contributors to failure of vasectomy reversal (123, 124). An alternative to surgical vasectomy reversal either instead of, or after failed vaso-vasostomy, is sperm harvesting from epididymis or testis in conjunction with intracytoplasmic sperm injection (ICSI)/in-vitro fertilization. Currently cost-benefit analyses suggest that microsurgical vaso-vasostomy is more cost-effective and safer in both North America (125) and Europe (126), with the wife’s age being a key determinant (107). However, optimal management depends on local clinical expertise as well as access to microsurgery and reproductive technologies. The feasibility of successful vasectomy reversal has led to the proposal that, although vasectomy is intended as permanent, some individuals may consider it a reversible contraceptive method (127). USA national economic indicators strikingly influence rates of vasectomy and of vasectomy reversal, which are increased and decreased, respectively, according to the unemployment rate and personal income (128); whether this applies in countries with national health schemes that reduce financial limitations on access to elective health care remains unknown.
Modern Methods
VAS OCCLUSION
The efficacy, safety, simplicity, and acceptability of vasectomy suggest that a reversible mechanical method of vasa occlusion would be an attractive male contraceptive option. Since vasectomy reversal is neither cheap nor widely available, more reversible vasa occlusion methods are needed (129). A nonsurgical, potentially reversible technique involving percutaneous injections of polymers that harden in-situ to form occluding plugs which might be later removed to restore fertility was reported (130) but, despite preliminary positive findings (131), formal evaluation showed vasa occlusion had lower efficacy (inducing azoospermia) than vasectomy (132). In a phase II randomized clinical trial, urethane-coated nylon thread intra-vasa devices were more acceptable with fewer complications but were less effective in producing azoospermia, compared with no-scalpel vasectomy (133). A hydrophilic gel, composed of co-polymer of styrene and maleic anhydride delivered in dimethyl sulfoxide, forms a charged spermicidal biopolymer when injected into the vas deferens that is stable but potentially removable. Experiments in rats favor sodium bicarbonate over dimethyl sulphoxide for intravasal injection for effective reversal of occlusion (134). Preliminary non-comparative clinical evaluation showed azoospermia in 12 men with no pregnancies in their wives for 12 months following intravasal injection suggesting effective vasal occlusion (135); however, some morphologically damaged and non-functional sperm persist in the ejaculate (136) but the mechanisms of the deleterious effects on sperm structure and function remain unexplained(137). In an extended multi-center phase III study of 139 fertile married men who had a single, bilateral intravasal injection of a styrene maleic anhydride copolymer in 120 µl of dimethyl sulphoxide solution (Reversible Inhibition of Sperm under Guidance, RISUG) were followed for six months after injection. In six men, the injections failed to be delivered, but in the remainder, 110 achieved azoospermia within 1 month and 23 achieved azoospermia 3 to 6 months after the procedure. After ceasing condom use in the first 2 months after injection, there were no pregnancies in the 133 men with successful occlusion over the next 4 months whereas pregnancies were reported in two of six men who had failed injections; however, reversibility was not reported (138). Another phase III randomized controlled clinical trial has shown that a prototype implantable non-occlusive intra-vasal device, comprising a nylon thread encased in barium-impregnated polyurethane, is as effective for sterilization as standard no-scalpel sterilization with no more adverse effects (139); however the reversibility of this device remains to be demonstrated. A promising development for a reversible vas occlusion is a solution for transcutaneous intravasal injection which forms a hydrogel in the vas to reversibly occlude it. The injection solution, comprising sodium alginate conjugated with thioketal and including titanium dioxide and calcium chloride, transforms after injection into the vas deferens into an occluding hydrogel. Subsequently, the occluding hydrogel can be cleared in vivo by non-invasive application of ultrasound that generates reactive oxygen species in the hydrogel causing cleavage of the thioketal and recanalizing the vas deferens. Experimental studies in rats demonstrated fully effective contraception and reversibility (140). Other technical developments including percutaneous injection of sclerosants and transcutaneous delivery of physical agents (ultrasound, lasers) continue to be developed slowly (141).
HEATING
It has long been known (142) that even brief elevations of testicular temperature can profoundly suppress spermatogenesis (143) while sustained elevation may contribute to testicular pathology in cryptorchidism, varicocele, and occupational male infertility (144). A highly novel experimental approach has been reported using intravenously injected iron oxide nanoparticles that accumulate in the testis where they can be activated by an external alternating magnet field to generate testicular heating and damage to spermatogenesis (145); however, safe clinical application, and notably reversibility, remain to be demonstrated. Clinical studies evaluating the potential for tight scrotal supports as a practical male contraceptive method (146, 147) showed a reversible decrease in sperm output but of inadequate magnitude for reliable contraception. Experience of small numbers of men utilizing thermal male contraception has been favorable, apart from discomfort (148) but wider uptake would require substantial population education (149). Given the dubious acceptability and safety (150) of heat-induced suppression of sperm output, the feasibility of a male contraceptive method based on testicular heating remains to be established.
IMMUNOCONTRACEPTION
Sperm vaccines to interrupt fertility have long been of interest (151) with a 1937 patent issued for vaccination with semen (152). Sperm express unique epitopes within the immunologically protected adluminal compartment of the seminiferous tubules at puberty, long after the definition of immune self-tolerance hence explaining their potential autoimmunogenicity. Sperm autoimmunity may contribute to subfertility after vasectomy reversal and in ~7% of infertile otherwise healthy men (other than orchitis). Experimental models for an effective multivalent chimeric protein sperm vaccine targeting surface-expressed antigens involved in fertilization have been reported (153) but remain untested in men. Practical application of this method requires resolving problems of the millions of sperm ejaculated, representing a large antigenic load requiring virtually complete functional blockade, variability of individual immune responses, restricted access of antibodies into the seminiferous tubules and epididymis and the risks of autoimmune orchitis or immune-complex disease. Passive immunization may overcome the present limited predictability of active immunization with sperm antigens to reach quickly and maintain, as well as allowing for volitionally controllable offset, of effective immunocontraceptive titers (154). The smaller antigenic burden in the female reproductive tract requiring complete neutralization suggests that a sperm vaccine, using modern genetic engineering of sperm epitopes (155), may be better targeted for administration to women. However, the most suitable targets for contraceptive vaccines might be feral and wild animals (156, 157).
CHEMICAL (NON-HORMONAL) METHODS
The lack of commercial product development for hormonal male contraceptives has made attractive the alternative option of innovative non-hormonal mechanisms to inhibit sperm production and/or function. These approaches have focused on developing feasible, druggable targets lacking off-target adverse effects for novel product development using either opportunistic or planned approaches to identify novel leads.
Opportunistic approaches include the identification through fortuitous pharmacological observation of male reproductive effects of drugs or natural products. Among older drugs, an orally active spermicide concentrated in semen (158), drugs inhibiting spermatogenesis (159), ejaculation (160) or epididymal sperm function (161) have been identified. Further, among numerous plant products and natural medicines reputed to inhibit male fertility, the most widely tested was gossypol, a polyphenolic yellow pigment identified in China as causing epidemic infertility among workers ingesting raw cottonseed oil. In over 10,000 men purified gossypol reduced sperm output to <4 million/ml in >98% within 75 days with suppression maintained by a lower weekly maintenance dose (162). Although an effective male contraceptive, the systemic toxicity of gossypol, due to mitochondrial apoptosis (163), and irreversibility of sperm suppression precluded further clinical development (164) although it has potential anti-cancer applications (163). Subsequently, extracts of Tripterygium wilfordii, a traditional Chinese herbal medicine for rheumatoid arthritis and skin disorders, decrease sperm output and function and inhibit fertility in rodents and men. Studies aiming to characterize triptolide, an active alkaloid as a potential lead for an orally effective sperm function inhibitor, reveal prominent induction of germ cell apoptosis (165) at testicular (166) in addition to a post-testicular site of action (167). Additional opportunistic approaches include recognizing that the rapidly proliferating germinal epithelium is highly susceptible to cytotoxins such as drugs, heat, or ionizing irradiation which damage germ cell replication, resulting in inhibition of spermatogenesis. However, complete elimination of sperm by non-specific toxicity compromises full reversibility and the accompanying mutagenic risk from direct interference with DNA replication precludes safe use for reversible male contraception.
Alternatively, potential non-hormonal approaches to developing chemical male contraception focus on sperm development maturation and function (168) ensuring that targets are specific for sperm (169). Such approaches may exploit the numerous biological processes required for developing functionally mature, fertile sperm that create abundant targets for reversible inhibition of male fertility (170) . Prominent targets include either reducing sperm output via non-hormonal mechanisms regulating spermatogenesis or post-testicular inhibition of sperm functions. One approach to reduce sperm output is by exploiting the essential requirement for retinoic acid in spermatogenesis (171). Experimental genetic or pharmacological inhibition of vitamin A action inhibits the generation of mature sperm and male fertility (172, 173); however, the ubiquitous roles of vitamin A in cellular replication and differentiation requires thorough safety evaluation for off-target effects in clinical trials. More specific focus on the retinoic acid alpha receptor by developing alpha-specific inhibitors continue in pre-clinical development (174). Another series of orally active indazole carboxylic acid analogs, adjudin, gamendazole and indenopyridine derivatives (175-178), have been developed from the compound lonidamine but aiming to eliminating its non-specific muscle and liver toxicity while retaining its mechanism of action in causing reversible male infertility. The indazole carboxylic acid analogs cause reversible subfertility by disrupting highly specialized intercellular junctions between elongating spermatids and Sertoli cells leading to precocious detachment of immature, elongating spermatids that are then shed prematurely from the germinal epithelium. Clinical evaluation of such drugs remains at an early pre-clinical stage. Other discoveries of molecules with specific expression in sperm and contributions to sperm development and/or fertilizing ability may provide clues to novel leads for chemical non-hormonal male contraceptive drug development. These include protein phosphatase complex (179), cyclin dependent kinase 2 (180), testis-specific bromodomain (181), homeodomain-interacting protein kinase 4 (182) and histone demethylase KDM5B (183).
The seclusion of functionally immature post-meiotic, haploid sperm during their transit through seminiferous tubules and epididymis offers targets for chemical methods to regulate male fertility as sperm are stored and mature functionally. Post-testicular targets offer the advantages of fast onset and offset of action compared with hormonal methods; however, specific target identification, selective drug targeting to the epididymis or testis and human dose optimization remain challenging problems. A model, rapid-onset oral spermicide was first provided by the chlorosugars that showed rapid, irreversible effect on rodent epididymal sperm (184) but proved too toxic for clinical development. A recent promising drug lead was the recognition that an alkylated iminosugar drugs that inhibit glucosyltransferase, used therapeutically to reduce lysosomal glycosphingolipid accumulation in storage disorder type 1 (Gaucher's disease), miglustat, was a potent and reversible oral inhibitor of male mouse fertility but free from apparent systemic toxicity (161, 185). Miglustat treatment produced structural malformation of sperm acrosome, head and mid-piece with consequential impaired motility although sperm retain the ability to fertilize oocytes in-vitro and produce normal offspring. However, miglustat effects were species- and mouse strain-dependent and were not effective in rabbits (186) or men (187). Numerous proteins identified as specifically or uniquely expressed in the epididymis provide additional opportunities for development of novel non-hormonal male contraceptive targets (188-192). Signaling enzymes involved in the epididymal acquisition of sperm motility and capacity for hyperactivation and fertilization such as sperm-specific protein phosphatase PPIγ2, glycogen synthase kinase 3 (GSK3), and calcium regulated phosphatase calcineurin (PP2B) and protein kinase A (PKA) have key role in sperm maturation, activation and fertilization that might provide clues to novel chemical, non-hormonal male contraceptive drugs (193). Post-testicular inhibition of purinergic and adrenergic receptors in the vas deferens to inhibit sperm movement through the ejaculatory ducts are feasible (194, 195), and a series of these receptor antagonists have been developed (196). However, interference with ejaculation is unlikely to be an acceptable form of male contraception. An opportunistic approach based on a FDA-approved excipient, N,N dimethylacetamide that produced a reversible reduction in fertility of rats without hormonal effects has been reported (197).
The most rapidly growing area of opportunity arises from serendipitous discoveries of genes found to be necessary for normal fertility, often from gene knock-out mouse models displaying unexpected male sterility or subfertility (170). This rapidly expanding list includes distinctive steps in spermatogenesis involving either biological processes unique to spermatogenesis, notably meiosis (two-stage reductive division of diploid germinal cells into haploid gametes) and spermiogenesis (metamorphosis of haploid round spermatids into spermatozoa) or inhibiting functions essential to sperm fertilizing ability such as flagellar motility and sperm motion (including hyperactivation (198)), excurrent ductular transport, acrosome reaction (199), chemoattraction to and fertilization of oocytes (194, 200), acrosomal function (201)and testis-specific serine/threonine kinases involved in development of normal sperm morphology and function (202, 203). The most frequent mechanisms involves inhibition of ion channels in sperm (200, 204-211) or vas deferens smooth muscle (194, 195) leading to inhibition of sperm motility, hyperactivation and/or transport required for fertilization. One leading candidate is CatSper, the principal calcium channel activated by progesterone (212-216) and essential for the sperm hyperactivation required for fertilization (217). Experimental vaccines against CatSper epitopes inhibit murine fertility (218, 219).
In addition, drug screening programs using extensive high-throughput chemical libraries can be employed to identify suitable lead compounds using selective sperm function targets for further development including target optimization (220). For example, the inhibitor analogs of CatSper using patch clamping to identify channel blockade have identified probes suitable for drug discovery development (221). Similarly, screening of a chemical library identified phosducin-like 2, a testis specific phosphoprotein, which when knocked-out in mice, induced sterility due to globozoospermia with impaired sperm head formation (222) as well as screening of natural plant (223-225) and microbial (226) products continue as a source for lead compounds continues. Other products of drug screening that may interfere with DNA replication by targeting meiosis, incur additional theoretical safety risks of mutagenesis if intended for male contraception (227).
HORMONAL METHODS
Hormonal methods are the closest to meeting the requirement for a reliable, reversible, safe, and acceptable male contraceptive. Although reliability is judged by the efficacy in preventing pregnancy in fertile female partners, as a hormonal male contraceptive aims to prevent pregnancy by reversible inhibition of sperm output, the suppression of spermatogenesis constitutes a useful surrogate marker for development and evaluation of prototype male contraceptive regimens. This makes defining the degree of suppression of sperm output required a key strategic issue in developing a hormonal male contraceptive (228).
Two landmark WHO studies, the first ever male contraceptive efficacy studies, involving 671 men from 16 centers in 10 countries established the proof of principle that hormonally-induced azoospermia provides highly reliable, reversible contraception (14, 15). Among the minority (~25%) of men who remained severe oligozoospermic (0.1-3 million sperm/ml) using weekly testosterone enanthate injections, contraceptive failure rate (~8% per annum) was directly proportional to their sperm output. Hence to achieve highly effective contraception, azoospermia is analogous to anovulation as a sufficient, but not necessary, requirement. Nevertheless, reliable contraception by modern standards (29) requires uniform azoospermia as the desirable target for male contraceptive regimens (229). No regimen yet achieves this consistently in all men, although in some Asian countries (e.g. China (15), Indonesia (230, 231)) an approximation to uniform azoospermia can be achieved by a variety of regimens. A study involving 308 Chinese men in 6 centers has shown that monthly injections of testosterone undecanoate provides highly effective and reversible contraception (17). No pregnancies were recorded among men who were azoospermic or severely oligozoospermic (≤3 million sperm per mL) providing a 95% upper confidence limit of pregnancy (contraceptive failure) rate of 2.5% per annum. The overall failure rate based on suppression of spermatogenesis was <4%. The prototype regimen was well tolerated apart from injection site discomfort due to large oil injection volume (4 mL) and reversible androgenic effects (acne, weight gain, hemoglobin, lipids). Nevertheless, despite these promising findings, non-Chinese men require combination hormonal regimens involving a 2nd gonadotropin suppressing agent, notably progestins, together with testosterone to ensure uniformly adequate spermatogenic suppression. Proof-of-principle for this combination approach was provided by a depot androgen/progestin regimen that observed no pregnancies among 55 couples during 35.5 person-years of exposure (95% upper limit of failure rate ~8%) in a study with satisfactory tolerability and reversibility for a prototype regimen (18). Hence, contraceptive efficacy studies show that highly effective contraception can be achieved with suppression of sperm output to near azoospermia (≤1 million per mL) (229). Ultimately, the efficacy of male contraception must be established by enumerating pregnancies prevented, and not counting sperm. The present paucity of male contraceptive efficacy studies, for which placebo controls are ethically impossible (232), makes systematic evaluations comparing practical clinical regimens a task for the future (233).
The reversibility of hormonal male contraceptive regimens is clearly established by an integrated re-analysis that pooled primary data from over 90% of all hormonal male contraceptive studies reported to show that all regimens show full reversibility within a predictable time course (234). This comprehensive review of the recovery of 1549 healthy eugonadal men, aged 18-51 years, who underwent 1283.5 man-years of treatment and 705 man-years of post-treatment recovery, showed the median times for recover to sperm densities of 10 and 20 million per mL were 2.5 months (2.4-2.7) and 3.0 months (2.9-3.1), respectively. Covariables such as age, ethnicity and hormonal or sperm output kinetics had significant but minor influence on the rate, but not the extent, of recovery.
Acceptability of a hormonal male contraceptive is high across a wide range of countries and cultures in potential male as well as female users (12). Willingness to use a hypothetical hormonal male contraceptive averaged 55% (range 29-71%) in an extensive, population representative survey of 9342 men aged 18-50 yr from 9 countries (4 Europe, 3 South America, Indonesia and USA) with consistency across a wide range of socio-economic and cultural settings (235, 236). Similar findings are reported in a 4-country study (UK, South Africa, Hong Kong, Shanghai) with 44-83% in each center (237) as well as 75% in Australia willing to try a hormonal male contraceptive (238). A majority of men in a US study were satisfied with and would recommend a transdermal gel-based hormonal contraceptive (239) and a majority of Chinese men were satisfied with monthly injections (240). Female partners from a variety of cultures also indicate high acceptability in a survey of 1894 women in 4 countries, among whom 40-78% would support and trust their male partners in stable relationships to use a hormonal male contraceptive (241). Corroborating the acceptability of hormonal male contraception are findings from experimental studies of prototype regimens for up to 12 months usage in which most participants confirm high levels of satisfaction and willingness to try a commercial product (239, 240, 242, 243).
Modern safety evaluation for hormonal contraceptive methods requires long-term, large scale studies of marketed products (244). Consequently, in the absence of any marketed male contraceptive, no long-term safety profile can be discerned. Nevertheless, 4 decades of clinical trials have consistently identified mainly minor adverse effects in short- or medium-term studies of prototype male hormonal contraception regimens (1, 8, 228). This was verified in an unique, placebo-controlled study of a combined androgen-progestin regimen which again proved highly effective at reversible suppression of sperm output although the inclusion of a placebo arm rendered it ethically impossible to evaluate contraceptive efficacy but provided insight into drug-related side effects (11). The study confirmed the benign medium-term safety profile for this prototype regimen in observing few serious adverse effects, none attributable to the steroid regimen, and, among the non-serious adverse effects reported, expected androgenic effects (acne, weight gain, sweating, changes in mood or libido) were more frequent than placebo but mild and rarely led to discontinuation (11). Nevertheless, the long-term safety evaluation of novel contraceptives requires large scale observational studies of extensively used drugs to define low frequency risks but can only occur after the marketing of suitable products.
Hence, prototype hormonal methods have proven reliability and reversibility and reasonable prospects for being well accepted and safe. Although they are the most likely opportunity in the foreseeable future to develop a practical contraceptive method for men, progress depends on pharmaceutical industry development. However, leadership in male contraceptive development has come almost exclusively from university-based investigators working with public sector organizations (8), notably WHO (245), CONRAD (246), and the Population Council (247). By contrast, commitment from pharmaceutical companies, including those that flourished in the post-war decades through developing female hormonal contraception, continued to languish over recent decades (24, 248) and is effectively ceased (249).
Steroidal Methods
ANDROGEN ALONE
Testosterone provides both gonadotropin suppression and androgen replacement making it an obvious first choice as a single agent for a reversible hormonal male contraceptive. Although androgen-induced, reversible suppression of human spermatogenesis has long been known (250-253), systematic studies of androgens for male contraception began in the 1970's (254, 255). Feasibility and dose-finding studies (256), mostly using testosterone enanthate (TE) in an oil vehicle as a prototype, showed that weekly im injections of 100-200 mg TE induce azoospermia in most Caucasian men (257)but less frequent or lower doses fail to sustain suppression (258-261). The largest experience with an androgen alone regimen arises from the two WHO studies in which over 670 men from 16 centers in 10 countries received weekly injections of 200 mg TE. In these studies ~60% of non-Chinese and >90% of Chinese men became azoospermic and the remainder were severely oligozoospermic (14, 15) (Figure 1). The high efficacy among Chinese men has also been replicated using monthly TU injections (16, 17). Effective gonadotropin suppression is a prerequisite for effective testosterone-induced spermatogenic suppression in human (18, 262-266) and non-human primates (267, 268). However, the reasons for within and between population differences in susceptibility to hormonally-induced azoospermia remain largely unexplained (269). Possible factors include population differences in reproductive physiology of environmental (270, 271), genetic (272-274) or uncertain (275, 276) origin that may lead to differences in rate and extent of suppression of circulating gonadotropins and/or depletion of intratesticular androgens. Limited invasive studies measuring intratesticular testosterone (and DHT) suggest that the degree of depletion may not predict reliably complete suppression of sperm output (277-279) but other more widely applicable, non-invasive markers of endogenous Leydig cell function such as circulating epitestosterone (262) or 17-hydroxyprogesterone (280) or non-steroidal testicular products such as INSL3 (281) may be more analytically informative as to the relative roles of gonadotropin suppression and intratesticular androgen depletion. Exogenous testosterone causes suppression of sperm output with an average of 13 weeks to reach severe oligozoospermia (<1 million per mL) or azoospermia with suppression being maintained consistently during ongoing treatment (282) (Figure 2). Following cessation of treatment, sperm reappear within 3 months to reach sperm densities of 10 and 20 million per mL at an average of 11.5 and 13.6 weeks, respectively (282)with ultimately full recovery (10) (Figure 3). Apart from intolerance of weekly injections, there were few discontinuations due to acne, weight gain, polycythemia, or behavioral effects and these were reversible as were changes in hemoglobin, testis size, and plasma urea. There was no evidence of liver, prostate, or cardiovascular disorders (14, 15, 283).
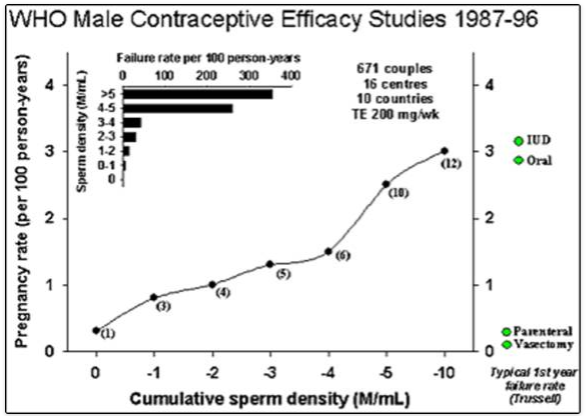
Figure 1. Pooled summary of contraceptive efficacy from two WHO male contraceptive efficacy studies (14, 15) where contraceptive failure rate (pregnancy rate) is plotted against the current sperm concentration in the ejaculate. This illustrates a summation of all data pooled from both studies. Data comprise monthly observations of the mean sperm concentration (averaging monthly sperm counts) and whether a pregnancy occurred in that month or not. Pregnancy rate (per 100 person-years, Pearl index) on the y-axis is plotted against the cumulative sperm density (in million sperm per ml) indicating that contraceptive failure rates are proportional to sperm output. The inset is the same data re-plotted in discrete sperm concentration bands rather than cumulatively. For comparison, the average contraceptive failure rates in the first year of use (33, 407) of modern reliable contraceptive methods are indicated by diamond symbols.
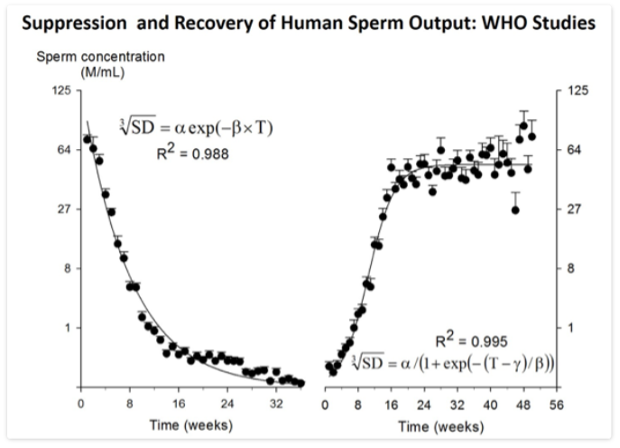
Figure 2. Plot of suppression (left panel) and recovery (right panel) of sperm output from the WHO Studies 85921 (15) and 89903 (14) involved pooling ~14,000 semen samples from 16 centers in 10 countries (282). Data-points represent mean and SE (error bar) of semen samples grouped within weeks with between six and 383 samples per time-point. Note cube-root scale on y-axis. For suppression, the smooth line is the two-parameter, single term exponential decay function plotted to fit the data by non-linear regression. For recovery, the smooth line is the three-parameter sigmoidal curve plotted to fit the data by non-linear regression.
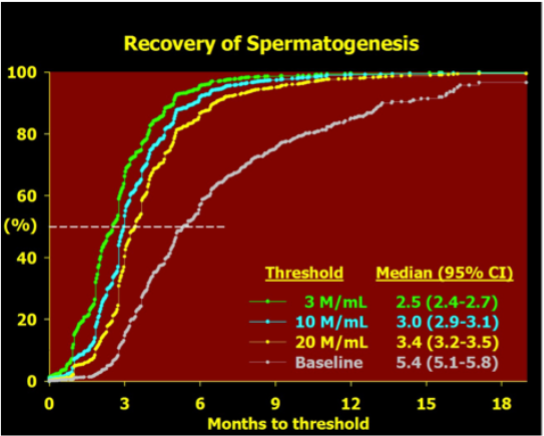
Figure 3. Recovery of spermatogenesis after cessation of treatment with hormonal male contraceptive regimens modified after an integrated re-analysis of over 90% of all reported studies (234). Data is plotted as a Kaplan-Meier survival plot of the increasing proportion of men recovering to various thresholds over time since last treatment. The data of last treatment is defined as the time elapsed from the end of the last treatment cycle, that is the latest date of the first missed treatment dose. The thresholds are a sperm concentration of 3, 10 or 20 million sperm per mL in the ejaculate or a return to their own pre-treatment baseline sperm concentration. The median time to achieving each threshold is tabulated together with its 95% confidence interval.
The pharmacokinetics of testosterone products are crucial for suppressing sperm output. Oral androgens have major first-pass hepatic effects producing prominent route-dependent effects on hepatic protein secretion (e.g., SHBG, HDL cholesterol) and inconsistent bioavailability. Short-acting testosterone products requiring daily or more frequent administration (oral, transdermal patches or gels) which may be acceptable for androgen replacement therapy present serious feasibility challenges in compliance for effective long-term hormonal contraception. Weekly TE injections required for maximal suppression of spermatogenesis (256) are far from ideal (284) and cause supraphysiological blood testosterone levels risking both excessive androgenic side effects and preventing maximal depletion of intratesticular testosterone for optimal efficacy (285, 286). Other currently available oil-based testosterone esters (cypionate, cyclohexane-carboxylate, propionate) do not differ from the enanthate ester (287), but longer-acting depot preparations such as injectable testosterone undecanoate are a substantial improvement. Subdermal testosterone pellets sustain physiological testosterone levels for 4-6 months (288) and the newer injectable preparations testosterone undecanoate (17), testosterone-loaded biodegradable microspheres (289) and testosterone buciclate (290) provide 2-3 months duration of action. Depot androgens suppress spermatogenesis faster, at lower doses and with fewer metabolic side effects than TE injections but azoospermia is still not achieved uniformly (291) although when combined with a depot progestin, this goal is achievable (18).
Oral synthetic 17-a alkylated androgens such as methyltestosterone (292), fluoxymesterone (293), methandienone (294)and danazol (295, 296) suppress spermatogenesis but azoospermia is rarely achieved and the inherent hepatotoxicity of the 17-a alkyl substitutent (297, 298) renders them unsuitable for long-term use. Athletes self-administering supratherapeutic doses of androgens also exhibit suppression of spermatogenesis (294, 299). Synthetic androgens lacking the 17-a alkyl substituent have been little studied although injectable nandrolone esters produce azoospermia in 88% of European men (300, 301) whereas oral mesterolone is ineffective (302). On the other hand, nandrolone hexyloxyphenylpropionate alone was unable to maintain spermatogenic suppression induced by a GnRH antagonist (303) in a prototype hybrid regime (where induction and maintenance treatment differ) whereas testosterone appears more promising (304). A 7-methyl derivative of nandrolone (MeNT), which is partly aromatisable but resistant to 5α-reductive amplification of androgenic potency, has been studied as a non-oral androgen for hormonal male contraceptive regimens (305). While its non-amplification by 5α reduction may be theoretically prostate-sparing (306), dose titration to achieve essential androgen replacement at each relevant tissue might be more difficult to achieve than for testosterone (307) or impossible. Non-aromatisable androgens which lack estrogen- receptor-mediated effects such as on bone density maintenance (308) and sexual function (309, 310) may not be ideal for long-term hormonal contraception. More potent, synthetic androgens lacking 17-a alkyl groups (311, 312) remain to be evaluated.
Adverse effects due to testosterone administration in prototype hormonal contraceptive regimens include (asymptomatic) polycythemia, weight (muscle) gain, and acne as well as changes in mood or sexual behavior. These are usually minor in severity, reversible upon cessation of treatment and of minimal clinical significance (11).
The safety of androgen administration concerns mainly potential effects on cardiovascular and prostatic disease As the explanation for the higher male susceptibility to cardiovascular disease is not well understood, the risks of exogenous androgens are not clear (313, 314). In clinical trials, lipid changes are minimal with depot (non-oral) hormonal regimens (18, 291, 315, 316). Changes in blood cholesterol fractions observed during high hepatic exposure to testosterone and/or progestins, due to either oral first pass effects or high parenteral doses, have unknown clinical significance but, in any case, maintenance of physiological blood testosterone concentrations is the prudent and preferred objective. The real cardiovascular risks or benefits of hormonal male contraception will require long-term surveillance of cardiovascular outcomes (317).
The long-term effects of exogenous androgens on the prostate also require monitoring since prostatic diseases are both age and androgen dependent. Exposure to adult testosterone levels is required for prostate development and disease (318-320). The precise relationship of androgens to prostatic disease and in particular any influence of exogenous androgens remains poorly understood. Ambient blood testosterone or DHT levels do not predict development of prostatic cancer over future decades in prospective studies of adults (321). A genetic polymorphism, the CAG (polyglutamine) triplet repeat in exon 1 of the androgen receptor, is an important determinant of prostate sensitivity to circulating testosterone with short repeat lengths leading to increased androgen sensitivity (322), however the relationship of the CAG triplet repeat length polymorphism to late-life prostate diseases remains unclear (323). Among androgen deficient men, prostate size and PSA concentrations are reduced and returned towards normal by testosterone replacement without exceeding age-matched eugonadal controls (322, 324-326). In healthy middle-aged men without known prostate disease, very high doses of the potent natural androgen DHT for 2 years did not increase prostate size or age-related growth rate compared with placebo indicating that effects of even high dose exogenous androgen treatment has much less effect than age on the human prostate (308). Similarly, self-administration of massive androgen over-dosage does not increase total prostate volume or PSA in anabolic steroid abusers although central prostate zone volumes increases (327). In-situ prostate cancer is common in all populations of older men whereas rates of invasive prostate cancer differ many-fold between populations despite similar blood testosterone concentrations. This suggests that early and prolonged exposure to androgens may initiate in-situ prostate cancer, but later androgen-independent environmental factors promote the outbreak of invasive prostate cancer. Therefore, it is prudent to maintain physiological androgen levels with exogenous testosterone, which then might be no more hazardous than exposure to endogenous testosterone. Prolonged surveillance comparable with that for cardiovascular and breast disease in users of female hormonal contraception would be equally essential to monitor both cardiovascular and prostatic disease risk in men receiving exogenous androgens for hormonal contraception.
Extensive experience with testosterone in doses equivalent to replacement therapy in normal men indicates minimal effects on mood or behavior (14, 15, 256, 328-330). A careful placebo-controlled, cross-over study showed that a 1000 mg TU injection in healthy young men produces minor mood changes without any detectable increase in self or partner-reported aggressive, non-aggressive or sexual behaviors (331). By contrast, extreme androgen doses used experimentally in healthy men can produce idiosyncratic hypomanic reactions in a minority (332). Aberrant behavior in observational studies of androgen-abusing athletes or prisoners are difficult to interpret particularly to distinguished genuine androgen effects from the influence of self-selection for underlying psychological morbidity (333).
ANTI-ANDROGENS
Antiandrogens have been used to selectively inhibit epididymal and testicular effects of testosterone without impeding systemic androgenic effects (334). Cyproterone acetate, a steroidal antiandrogen with progestational activity, suppresses gonadotropin secretion without achieving azoospermia but leads to androgen deficiency when used alone (335). In contrast, pure non-steroidal antiandrogens lacking androgenic or gestagenic effects such as flutamide, nilutamide and casodex fail to suppress spermatogenesis when used alone (336, 337). Two studies evaluating the hypothesis that incomplete suppression of spermatogenesis is due to persistence of testicular DHT have reported no additional suppression from administration of finasteride, a type II 5a reductase inhibitor (338, 339); however as testes express predominantly the type I isoforms (340), further studies are required to conclusively test this hypothesis using an inhibitor of type I 5-a reductase (341).
ANDROGEN COMBINATION REGIMENS
Combination steroid regimens use non-androgenic steroids (estrogens, progestins) to suppress gonadotropins, in conjunction with testosterone for androgen replacement, have shown the most promising efficacy with enhanced rate and extent of spermatogenic suppression compared with androgen alone regimens (315, 342, 343). Synergistic combinations reduce the effective dose of each steroid and minimizing testosterone dosage could enhance spermatogenic suppression if high blood testosterone levels counteract the necessary maximal depletion of intratesticular testosterone (344, 345) as well as reducing androgenic side-effects (Figure 4).
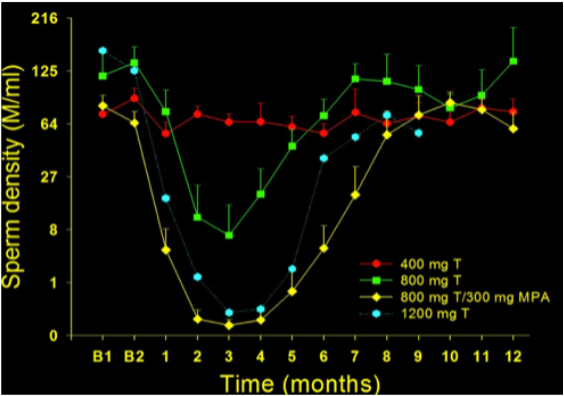
Figure 4. Plot of time course of sperm output (expressed as sperm density in millions of sperm per mL) before and after implantation of two 200-mg testosterone pellets (400 mg total; closed circles), four 200-mg testosterone pellets (800 mg total; closed squares), four testosterone pellets plus depot progestin (800 mg total testosterone plus 300 mg DMPA; closed diamonds) and six 200 mg testosterone pellets mg (1200 mg total; open hexagons) in healthy fertile men (262). Results expressed as the mean and SEM. Note the cube root transformed scale on the y-axis.
Progesterone is a key precursor and steroidogenic intermediate for all bioactive natural steroids and the progesterone receptors A and B are structurally and evolutionarily the closest members of the nuclear receptor superfamily to the androgen receptor. Yet, although progesterone has crucial gestational and lactational roles in female reproductive physiology, it has no well-established role in male reproductive physiology apart from a possible role in sperm function (200, 346), possibly via non-genomic rather than a classically genomic mechanism (347). Nevertheless functional nuclear progesterone receptors are expressed in male brain, smooth muscle and reproductive, but not most non-reproductive, tissues (348). Synthetic progestins, steroidal structural agonistic analogs of progesterone, are potent inhibitors of pituitary gonadotropin secretion used widely for female contraception and hormonal treatment of disorders such as endometriosis, uterine myoma and mastalgia. Used alone, progestins suppress spermatogenesis but cause androgen deficiency including impotence (349, 350) so androgen replacement is necessary. Non-human primate studies indicate that this is mediated via a central hypothalamic-pituitary site of action rather than direct effects on the testis (351). Extensive feasibility studies concluded that progestin-androgen combination regimens had promise as hormonal male contraceptives if more potent and durable agents were developed (256, 352). The largest and most detailed multi-center study of the contraceptive efficacy of a androgen-progestin combination involving 320 healthy men aged 18-45 years together with their 18-38 year old female partners, both without known fertility problems, studied for up to 56 weeks while having intramuscular injections of 200 mg norethisterone acetate and testosterone undecanoate every 8 weeks that produced near-complete suppression (96% declined to <1 million/ml by 24 weeks) and reversible recovery (95% recovered by 52 weeks) of sperm output (353). Four pregnancies occurred in partners of 266 men with a pregnancy failure rate of 1.6% (95% confidence interval 0.6-4.1%). Prior information on androgen/progestin regimens derives from studies with medroxyprogesterone acetate (MPA) combined with testosterone. Monthly injections of both agents or daily oral progestin with dermal androgen gels produce azoospermia in ~60% of fertile men of European background with the remainder having severe oligozoospermia and impaired sperm function (256, 352, 354). Nearly uniform azoospermia is produced in men treated with depot MPA and either of two injectable androgens in Indonesian men (230, 231) or testosterone depot implants in Caucasian men (315). Smaller studies with other oral progestins such as levonorgestrel (342, 355, 356) and norethisterone (357, 358) combined with testosterone demonstrate similar efficacy to oral MPA whereas cyproterone acetate with its additional anti-androgenic activity has higher efficacy in conjunction with TE (343, 359) but not oral testosterone undecanoate (360). Highly effective suppression of spermatogenesis is reported with depot progestins in the form of non-biodegradable implants of norgestrel (361-363) or etonorgestrel (364, 365) or depot injectable medroxyprogesterone acetate (18, 315, 366, 367) or norethisterone enanthate (368, 369)coupled with testosterone (370). A comparative study showed that all four progestins (cyproterone acetate, levonorgestrel, norethisterone acetate and nesterone) displayed significant short-term gonadotrophin suppression when combined with testosterone (371). The pharmacokinetics of the testosterone preparation is critical to efficacy of spermatogenic suppression with long-acting depots being most effective while transdermal delivery is less effective than injectable testosterone (361). Progestin side-effects are few if sexual function and well-being are maintained by adequate doses of testosterone replacement. The metabolic effects depend on specific regimen with oral administration and higher testosterone doses exhibiting more prominent hepatic effects such as lowering SHBG and HDL cholesterol. After treatment ceases with depletion or withdrawal of hormonal depots, spermatogenesis recovers completely but gradually consistent with the time-course of the spermatogenic cycle (234, 282). Steroids with dual androgen and progestin properties may be potentially well suited to male contraception if the balance between these two bioactivities is properly balanced (372). A study comparing a gel combining nesterone (8.3 mg) with testosterone (62.5 mg) with the same dose of testosterone alone for daily transdermal application for 28 days demonstrated suppression of sperm output and serum gonadotropin to levels consistent with contraceptive efficacy and superior to testosterone alone indicating the suitability of the combination gel for further product development (373). Two orally active nandrolone derivatives, dimethandrolone (374-376) and 11-methyl nandrolone dodecylcarbonate (377), are undergoing promising, early stage clinical development showing high acceptability in a short-term, placebo-controlled clinical trials (378, 379). Whether such daily use oral or transdermal gel (239, 380)-based male contraceptives will have sufficient user compliance to attain high contraceptive efficacy remains to be evaluated.
Estradiol augments testosterone-induced suppression of primate spermatogenesis (381) and fertility (382) but estrogenic side-effects (gynecomastia) and modest efficacy at tolerable doses make estradiol-based combinations impractical for male contraception (383). The efficacy and tolerability of newer estrogen analogs in combination with testosterone remain to be evaluated.
GONADOTROPIN-RELEASING HORMONE BLOCKADE
The pivotal role of GnRH in the hormonal control of testicular function makes it an attractive target for biochemical regulation of male fertility. Blockade of GnRH action by GnRH receptor blockade with synthetic analogs or GnRH immunoneutralization would eliminate LH and testosterone secretion requiring testosterone replacement. Many superactive GnRH agonists are used to induce reversible medical castration for androgen-dependent prostate cancer by causing a sustained, paradoxical inhibition of gonadotropin and testosterone secretion and spermatogenesis due to pituitary GnRH receptor downregulation. When combined with testosterone, GnRH agonists suppress spermatogenesis but rarely achieve azoospermia (344, 345, 384) being less effective than androgen/progestin regimens. By contrast, pure GnRH antagonists create and sustain immediate competitive blockade of GnRH receptors (385, 386) and, in combination with testosterone, are highly effective at suppressing spermatogenesis. Early hydrophobic GnRH antagonists were difficult to formulate and irritating, causing injection site mast cell histamine release. Newer more potent but less irritating GnRH antagonists produce rapid, reversible and complete inhibition of spermatogenesis in monkeys (387-389) and men (390, 391) when combined with testosterone. The striking superiority of GnRH antagonists over agonists may be due to more effective and immediate inhibition of gonadotropin secretion and thereby more effective depletion of intratesticular testosterone. Due to their highly specific site of action, GnRH analogs have few unexpected side-effects. Depot GnRH antagonist plus testosterone formulations suitable for administration at up to 3-month intervals could be promising as a hormonal male contraceptive regimen. Whether GnRH antagonists are more cost-effective than progestins as the second, non-androgenic component of combination male hormonal contraceptive regimens remains to be established (278, 303, 367, 392). The drawback of higher cost might be overcome by hybrid regimens using GnRH antagonists to initiate suppression followed by a switch to more economical steroids for maintenance of spermatogenic suppression in humans (304). A GnRH vaccine could intercept GnRH in the pituitary-portal bloodstream preventing its reaching pituitary GnRH receptors. A limitation of a GnRH vaccine is its uncertain and/or unpredictable reversibility. If it is not irreversible, the offset of contraceptive efficacy may vary between individuals and create practical difficulties in ensuring reliable contraception. Gonadotropin-selective immunocastration would require androgen replacement in men (393) and pilot feasibility studies in advanced prostate cancer are underway (394)but the prospects for acceptably safe application for male contraception remain doubtful (395). By contrast there are growing applications for anti-hormonal contraceptive vaccines in control of companion (pet), agricultural, zoo, feral and wild animal populations (156, 396).
FOLLICLE STIMULATING HORMONE BLOCKADE
Selective FSH blockade theoretically offers the opportunity to reduce spermatogenesis without inhibiting endogenous testosterone secretion. FSH action could be abolished by selective inhibition of pituitary FSH secretion with inhibin (397)or novel steroids (398), by FSH vaccine (399, 400) or by FSH receptor blockade with peptide antagonists (401). Although FSH was considered essential to human spermatogenesis, spermatogenesis and fertility persist in rodents (402-404) and humans (405) lacking FSH bioactivity. Even complete FSH blockade alone might produce insufficient reduction in sperm output and function required for adequate contraceptive efficacy (406). In addition to the usual safety concerns of contraceptive vaccines including autoimmune hypophysitis, orchitis or immune-complex disease, an FSH vaccine might be overcome by reflex increases in pituitary FSH secretion. Hence, FSH suppression is a necessary but not sufficient for a hormonal male contraceptive regimen.
REFERENCES
1. Anderson RA, Baird DT 2002 Male contraception. Endocrine Reviews 23(6):735-762
2. United Nations 2006 Levels and trends of contraceptive use as assessed in 2002. New York: Department of International Economic and Social Affairs
3. Trussell J, Lalla AM, Doan QV, Reyes E, Pinto L, Gricar J 2009 Cost effectiveness of contraceptives in the United States. Contraception 79(1):5-14
4. Sedgh G, Singh S, Hussain R 2014 Intended and unintended pregnancies worldwide in 2012 and recent trends. Stud Fam Plann 45(3):301-314
5. Connelly M 2008 Fatal Misconception: The Struggle to Control World Population. Cambridge, Mass., United States: Harvard University Press
6. Mosher S 2008 Population Control: Real Costs, Illusory Benefits. Somerset, NJ, United States: Transaction Publishers
7. Nguyen BT, Brown AL, Jones F, Jones L, Withers M, Ciesielski KM, Franks JM, Wang C 2021 "I'm not going to be a guinea pig:" Medical mistrust as a barrier to male contraception for Black American men in Los Angeles, CA. Contraception 104(4):361-366
8. Nieschlag E 2010 Clinical trials in male hormonal contraception. Contraception 82(5):457-470
9. Liu PY, Swerdloff RS, Anawalt BD, Anderson RA, Bremner WJ, Elliesen J, Gu YQ, Kersemaekers WM, McLachlan RI, Meriggiola MC, Nieschlag E, Sitruk-Ware R, Vogelsong K, Wang XH, Wu FC, Zitzmann M, Handelsman DJ, Wang C 2008 Determinants of the rate and extent of spermatogenic suppression during hormonal male contraception: an integrated analysis. Journal of Clinical Endocrinology and Metabolism 93(5):1774-1783
10. Liu PY, Swerdloff RS, Christenson PD, Handelsman DJ, Wang C 2006 Rate, extent, and modifiers of spermatogenic recovery after hormonal male contraception: an integrated analysis. Lancet 367(9520):1412-1420
11. Mommers E, Kersemaekers WM, Elliesen J, Kepers M, Apter D, Behre HM, Beynon J, Bouloux PM, Costantino A, Gerbershagen HP, Gronlund L, Heger-Mahn D, Huhtaniemi I, Koldewijn EL, Lange C, Lindenberg S, Meriggiola MC, Meuleman E, Mulders PF, Nieschlag E, Perheentupa A, Solomon A, Vaisala L, Wu FC, Zitzmann M 2008 Male hormonal contraception: a double-blind, placebo-controlled study. J Clin Endocrinol Metab 93(7):2572-2580
12. Glasier A 2010 Acceptability of contraception for men: a review. Contraception 82(5):453-456
13. Reynolds-Wright JJ, Cameron NJ, Anderson RA 2021 Will Men Use Novel Male Contraceptive Methods and Will Women Trust Them? A Systematic Review. J Sex Res 58(7):838-849
14. WHO Task Force on Methods for the Regulation of Male Fertility 1996 Contraceptive efficacy of testosterone-induced azoospermia and oligozoospermia in normal men. Fertility and Sterility 65(821-829
15. WHO Task Force on Methods for the Regulation of Male Fertility 1990 Contraceptive efficacy of testosterone-induced azoospermia in normal men. Lancet 336(955-959
16. Gu Y, Liang X, Wu W, Liu M, Song S, Cheng L, Bo L, Xiong C, Wang X, Liu X, Peng L, Yao K 2009 Multicenter contraceptive efficacy trial of injectable testosterone undecanoate in Chinese men. J Clin Endocrinol Metab 94(6):1910-1915
17. Gu YQ, Wang XH, Xu D, Peng L, Cheng LF, Huang MK, Huang ZJ, Zhang GY 2003 A multicenter contraceptive efficacy study of injectable testosterone undecanoate in healthy Chinese men. Journal of Clinical Endocrinology and Metabolism 88(2):562-568
18. Turner L, Conway AJ, Jimenez M, Liu PY, Forbes E, McLachlan RI, Handelsman DJ 2003 Contraceptive efficacy of a depot progestin and androgen combination in men. J Clin Endocrinol Metab 88(10):4659-4667
19. Soufir JC, Meduri G, Ziyyat A 2011 Spermatogenetic inhibition in men taking a combination of oral medroxyprogesterone acetate and percutaneous testosterone as a male contraceptive method. Hum Reprod 26(7):1708-1714
20. Dorman E, Perry B, Polis CB, Campo-Engelstein L, Shattuck D, Hamlin A, Aiken A, Trussell J, Sokal D 2018 Modeling the impact of novel male contraceptive methods on reductions in unintended pregnancies in Nigeria, South Africa, and the United States. Contraception 97(1):62-69
21. Jacobsohn T, Nguyen BT, Brown JE, Thirumalai A, Massone M, Page ST, Wang C, Kroopnick J, Blithe DL 2022 Male contraception is coming: Who do men want to prescribe their birth control? Contraception 115(44-48
22. Tcherdukian J, Mieusset R, Netter A, Lechevallier E, Bretelle F, Perrin J 2022 Knowledge, professional attitudes, and training among health professionals regarding male contraceptive methods. Eur J Contracept Reprod Health Care 27(5):397-402
23. Nguyen BT, Jacobsohn TL 2022 Post-abortion contraception, an opportunity for male partners and male contraception. Contraception 115(69-74
24. Handelsman DJ 2003 Hormonal male contraception--lessons from the East when the Western market fails. Journal of Clinical Endocrinology and Metabolism 88(2):559-561
25. Nickels LM, Shane K, Vahdat HL 2022 Catalyzing momentum in male contraceptive development†. Biol Reprod 106(1):1-3
26. Balbach M, Fushimi M, Huggins DJ, Steegborn C, Meinke PT, Levin LR, Buck J 2020 Optimization of lead compounds into on-demand, nonhormonal contraceptives: leveraging a public-private drug discovery institute collaboration†. Biol Reprod 103(2):176-182
27. WHO Task Force on Methods for the Determination of the Fertile Period 1983 A prospective multicentre trial of the ovulation method of natural family planning. III Characteristics of the menstrual cycle and of the fertile phase. Fertility and Sterility 40(773-778
28. Trussell J, Grummer-Strawn L 1990 Contraceptive failure of the ovulation method of periodic abstinence. Family Planning Perspectives 22(2):65-75
29. Trussell J 2011 Contraceptive failure in the United States. Contraception 83(5):397-404
30. Moreau C, Bouyer J, Bajos N, Rodriguez G, Trussell J 2009 Frequency of discontinuation of contraceptive use: results from a French population-based cohort. Hum Reprod 24(6):1387-1392
31. Moreau C, Trussell J, Rodriguez G, Bajos N, Bouyer J 2007 Contraceptive failure rates in France: results from a population-based survey. Hum Reprod 22(9):2422-2427
32. Rogow D, Horowitz S 1995 Withdrawal: a review of the literature and an agenda for research. Studies in Family Planning 26(3):140-153
33. Kost K, Singh S, Vaughan B, Trussell J, Bankole A 2008 Estimates of contraceptive failure from the 2002 National Survey of Family Growth. Contraception 77(1):10-21
34. Potts M, Diggory P 1983 Textbook of Contraceptive Practice. 2nd ed. Cambridge: Cambridge University Press
35. Sundaram A, Vaughan B, Kost K, Bankole A, Finer L, Singh S, Trussell J 2017 Contraceptive Failure in the United States: Estimates from the 2006-2010 National Survey of Family Growth. Perspect Sex Reprod Health 49(1):7-16
36. Crosby R, Graham C, Milhausen R, Sanders S, Yarber W, Shrier LA 2015 Associations between rushed condom application and condom use errors and problems. Sex Transm Infect 91(4):275-277
37. Steiner MJ, Cates W, Jr., Warner L 1999 The real problem with male condoms is nonuse. Sex Transm Dis 26(8):459-462
38. Grady WR, Klepinger DH, Nelson-Wally A 1999 Contraceptive characteristics: the perceptions and priorities of men and women. Fam Plann Perspect 31(4):168-175
39. Rosenberg MJ, Waugh MS, Solomon HM, Lyszkowski AD 1996 The male polyurethane condom: a review of current knowledge. Contraception 53(3):141-146
40. Gallo MF, Grimes DA, Lopez LM, Schulz KF 2006 Non-latex versus latex male condoms for contraception. Cochrane Database Syst Rev1):CD003550
41. Cates W, Jr., Steiner MJ 2002 Dual protection against unintended pregnancy and sexually transmitted infections: what is the best contraceptive approach? Sex Transm Dis 29(3):168-174
42. Carey RF, Lytle CD, Cyr WH 1999 Implications of laboratory tests of condom integrity. Sex Transm Dis 26(4):216-220
43. Walsh TL, Frezieres RG, Peacock K, Nelson AL, Clark VA, Bernstein L, Wraxall BG 2003 Use of prostate-specific antigen (PSA) to measure semen exposure resulting from male condom failures: implications for contraceptive efficacy and the prevention of sexually transmitted disease. Contraception 67(2):139-150
44. Doncel GF 2006 Exploiting common targets in human fertilization and HIV infection: development of novel contraceptive microbicides. Hum Reprod Update 12(2):103-117
45. Liskin L, Benoit E, Blackburn R 1992 Vasectomy: New Opportunities. Baltimore: Population Information Program, Johns Hopkins University, Baltimore
46. Zhang X, Eisenberg ML 2022 Vasectomy utilization in men aged 18-45 declined between 2002 and 2017: Results from the United States National Survey for Family Growth data. Andrology 10(1):137-142
47. Drake MJ, Mills IW, Cranston D 1999 On the chequered history of vasectomy. BJU Int 84(4):475-481
48. EngenderHealth 2002 Contraceptive Sterilization: Global Trends and Issues. New York
49. Schwingl PJ, Guess HA 2000 Safety and effectiveness of vasectomy. Fertility and Sterility 73(5):923-936
50. Awsare NS, Krishnan J, Boustead GB, Hanbury DC, McNicholas TA 2005 Complications of vasectomy. Ann R Coll Surg Engl 87(6):406-410
51. Sokal D, Irsula B, Hays M, Chen-Mok M, Barone MA 2004 Vasectomy by ligation and excision, with or without fascial interposition: a randomized controlled trial. BMC Med 2(1):6
52. Cook LA, Van Vliet HA, Lopez LM, Pun A, Gallo MF 2014 Vasectomy occlusion techniques for male sterilization. Cochrane Database Syst Rev 3(Cd003991
53. Li S, Goldstein M, Zhu J, Huber D 1991 The no-scalpel vasectomy. Journal of Urology 145(341-344
54. Sokal D, McMullen S, Gates D, Dominik R 1999 A comparative study of the no scalpel and standard incision approaches to vasectomy in 5 countries. The Male Sterilization Investigator Team. Journal of Urology 162(5):1621-1625
55. Cook LA, Pun A, Gallo MF, Lopez LM, Van Vliet HA 2014 Scalpel versus no-scalpel incision for vasectomy. Cochrane Database Syst Rev 3(Cd004112
56. Christiansen CG, Sandlow JI 2003 Testicular pain following vasectomy: a review of postvasectomy pain syndrome. J Androl 24(3):293-298
57. Leslie TA, Illing RO, Cranston DW, Guillebaud J 2007 The incidence of chronic scrotal pain after vasectomy: a prospective audit. BJU Int 100(6):1330-1333
58. Aradhya KW, Best K, Sokal DC 2005 Recent developments in vasectomy. Bmj 330(7486):296-299
59. Cook LA, Pun A, van Vliet H, Gallo MF, Lopez LM 2007 Scalpel versus no-scalpel incision for vasectomy. Cochrane Database Syst Rev2):CD004112
60. Barone MA, Irsula B, Chen-Mok M, Sokal DC 2004 Effectiveness of vasectomy using cautery. BMC Urol 4(1):10
61. Sokal D, Irsula B, Chen-Mok M, Labrecque M, Barone MA 2004 A comparison of vas occlusion techniques: cautery more effective than ligation and excision with fascial interposition. BMC Urol 4(1):12
62. Shapiro EI, Silber SJ 1979 Open-ended vasectomy, sperm granuloma, and postvasectomy orchialgia. Fertil Steril 32(5):546-550
63. Errey BB, Edwards IS 1986 Open-ended vasectomy: an assessment. Fertil Steril 45(6):843-846
64. Moss WM 1992 A comparison of open-end versus closed-end vasectomies: a report on 6220 cases. Contraception 46(6):521-525
65. Berthelsen JG 1976 Peroperative irrigation of the vas deferens during vasectomy. Scand J Urol Nephrol 10(2):100-102
66. Leungwattanakij S, Lertsuwannaroj A, Ratana-Olarn K 2001 Irrigation of the distal vas deferens during vasectomy: does it accelerate the post-vasectomy sperm-free rate? Int J Androl 24(4):241-245
67. Mason RG, Dodds L, Swami SK 2002 Sterile water irrigation of the distal vas deferens at vasectomy: does it accelerate clearance of sperm? a prospective randomized trial. Urology 59(3):424-427
68. Pearce I, Adeyoju A, Bhatt RI, Mokete M, Brown SC 2002 The effect of perioperative distal vasal lavage on subsequent semen analysis after vasectomy: a prospective randomized controlled trial. BJU Int 90(3):282-285
69. Eisner B, Schuster T, Rodgers P, Ahmed M, Faerber G, Smith G, Ohl D 2004 A randomized clinical trial of the effect of intraoperative saline perfusion on postvasectomy azoospermia. Ann Fam Med 2(3):221-223
70. Albert PS, Seebode J 1977 Nitrofurazone: vas irrigation as adjunct in vasectomy. Urology 10(5):450-451
71. Edwards IS 1977 Vasectomy: irrigation with euflavine. Med J Aust 1(23):847-849
72. Gandrup P, Berthelsen JG, Nielsen OS 1982 Irrigation during vasectomy: a comparison between sterile water and the spermicide euflavine. J Urol 127(1):60-61
73. Henry Yu HY, Halim A, Evans PR 1976 Chlorhexidine for irrigation of vas: a clinical trial and the study of viability of non-motile sperms in post-vasectomy patients with trypan blue uptake. Br J Urol 48(5):371-375
74. Cook LA, Van Vliet H, Lopez LM, Pun A, Gallo MF 2007 Vasectomy occlusion techniques for male sterilization. Cochrane Database Syst Rev2):CD003991
75. Wood BL, Doncel GF, Reddy PR, Sokal DC 2003 Effect of diltiazem and methylene blue on human sperm motility, viability and cervical mucus penetration: potential use as vas irrigants at the time of vasectomy. Contraception 67(3):241-245
76. Griffin T, Tooher R, Nowakowski K, Lloyd M, Maddern G 2005 How little is enough? The evidence for post-vasectomy testing. J Urol 174(1):29-36
77. Sharlip ID, Belker AM, Honig S, Labrecque M, Marmar JL, Ross LS, Sandlow JI, Sokal DC 2012 Vasectomy: AUA guideline. J Urol 188(6 Suppl):2482-2491
78. Coward RM, Badhiwala NG, Kovac JR, Smith RP, Lamb DJ, Lipshultz LI 2014 Impact of the 2012 American Urological Association vasectomy guidelines on post-vasectomy outcomes. J Urol 191(1):169-174
79. Hancock P, Woodward BJ, Muneer A, Kirkman-Brown JC 2016 2016 Laboratory guidelines for postvasectomy semen analysis: Association of Biomedical Andrologists, the British Andrology Society and the British Association of Urological Surgeons. J Clin Pathol 69(7):655-660
80. Barone MA, Nazerali H, Cortes M, Chen-Mok M, Pollack AE, Sokal D 2003 A prospective study of time and number of ejaculations to azoospermia after vasectomy by ligation and excision. J Urol 170(3):892-896
81. Labrecque M, Hays M, Chen-Mok M, Barone MA, Sokal D 2006 Frequency and patterns of early recanalization after vasectomy. BMC Urol 6(25
82. Deneux-Tharaux C, Kahn E, Nazerali H, Sokal DC 2004 Pregnancy rates after vasectomy: a survey of US urologists. Contraception 69(5):401-406
83. Farber NJ, Flannigan R, Li P, Li PS, Goldstein M 2019 The Kinetics of Sperm Return and Late Failure Following Vasovasostomy or Vasoepididymostomy: A Systematic Review. J Urol 201(2):241-250
84. Philp T, Guillebaud J, Budd D 1984 Complications of vasectomy: review of 16,000 patients. Br J Urol 56(6):745-748
85. Rubin GL, Ory HW, Layde PM 1982 The mortality risk of voluntary surgical contraception. Biomed Bull 3(2):1-5
86. Peng XS, Li FD, Miao ZR, Ye XM, Wong Y, Hu XZ, Zhong ZZ, Zeng FX, Wu XQ, Lan J, et al. 1987 Plasma reproductive hormones in normal and vasectomized Chinese males. Int J Androl 10(2):471-479
87. Petitti DB 1986 Epidemiologic studies of vasectomy. In: Zatuchni GI, Goldsmith A, Spieler JM, Sciarra JJ eds. Male Contraception: Advances and future prospects. Philadelphia: Harper & Row; 24-33
88. Giovannucci E, Tosteson TD, Speizer FE, Vessey MP, Colditz GA 1992 A long-term study of mortality in men who have undergone vasectomy. N Engl J Med 326(21):1392-1398
89. Nienhuis H, Goldacre M, Seagroatt V, Gill L, Vessey M 1992 Incidence of disease after vasectomy: a record linkage retrospective cohort study. Bmj 304(6829):743-746
90. Moller H, Knudsen LB, Lynge E 1994 Risk of testicular cancer after vasectomy: cohort study of over 73,000 men. Bmj 309(6950):295-299
91. Strader CH, Weiss NS, Daling JR 1988 Vasectomy and the incidence of testicular cancer. Am J Epidemiol 128(1):56-63
92. Giovannucci E, Tosteson TD, Speizer FE, Ascherio A, Vessey MP, Colditz GA 1993 A retrospective cohort study of vasectomy and prostate cancer in US men. JAMA 269(7):878-882
93. Giovannucci E, Ascherio A, Rimm EB, Colditz GA, Stampfer MJ, Willett WC 1993 A prospective cohort study of vasectomy and prostate cancer in US men. JAMA 269(7):873-877
94. Siddiqui MM, Wilson KM, Epstein MM, Rider JR, Martin NE, Stampfer MJ, Giovannucci EL, Mucci LA 2014 Vasectomy and risk of aggressive prostate cancer: a 24-year follow-up study. J Clin Oncol 32(27):3033-3038
95. Borgmeier I, Holman CD 2004 Does vasectomy reversal protect against prostate cancer? Ann Epidemiol 14(10):748-749
96. Randall S, Boyd J, Fuller E, Brooks C, Morris C, Earle CC, Ferrante A, Moorin R, Semmens J, Holman CDJ 2018 The Effect of Vasectomy Reversal on Prostate Cancer Risk: International Meta-Analysis of 684,660 Vasectomized Men. J Urol 200(1):121-125
97. Bernal-Delgado E, Latour-Perez J, Pradas-Arnal F, Gomez-Lopez LI 1998 The association between vasectomy and prostate cancer: a systematic review of the literature. Fertil Steril 70(2):191-200
98. Nayan M, Hamilton RJ, Macdonald EM, Li Q, Mamdani MM, Earle CC, Kulkarni GS, Jarvi KA, Juurlink DN 2016 Vasectomy and risk of prostate cancer: population based matched cohort study. Bmj 355(i5546
99. Jacobs EJ, Anderson RL, Stevens VL, Newton CC, Gansler T, Gapstur SM 2016 Vasectomy and Prostate Cancer Incidence and Mortality in a Large US Cohort. J Clin Oncol 34(32):3880-3885
100. Smith K, Byrne, Castano JM, Chirlaque MD, Lilja H, Agudo A, Ardanaz E, Rodriguez-Barranco M, Boeing H, Kaaks R, Khaw KT, Larranaga N, Navarro C, Olsen A, Overvad K, Perez-Cornago A, Rohrmann S, Sanchez MJ, Tjonneland A, Tsilidis KK, Johansson M, Riboli E, Key TJ, Travis RC 2017 Vasectomy and Prostate Cancer Risk in the European Prospective Investigation Into Cancer and Nutrition (EPIC). J Clin Oncol 35(12):1297-1303
101. Bhindi B, Wallis CJD, Nayan M, Farrell AM, Trost LW, Hamilton RJ, Kulkarni GS, Finelli A, Fleshner NE, Boorjian SA, Karnes RJ 2017 The Association Between Vasectomy and Prostate Cancer: A Systematic Review and Meta-analysis. JAMA Intern Med 177(9):1273-1286
102. Shoag J, Savenkov O, Christos PJ, Mittal S, Halpern JA, Askin G, Shoag D, Golan R, Lee DJ, O'Malley P, Najari B, Eisner B, Hu JC, Scherr D, Schlegel P, Barbieri CE 2017 Vasectomy and Risk of Prostate Cancer in a Screening Trial. Cancer Epidemiol Biomarkers Prev 26(11):1653-1659
103. Carbone DJ, Jr., Shah A, Thomas AJ, Jr., Agarwal A 1998 Partial obstruction, not antisperm antibodies, causing infertility after vasovasostomy. J Urol 159(3):827-830
104. Royle MG, Parslow JM, Kingscott MM, Wallace DM, Hendry WF 1981 Reversal of vasectomy: the effects of sperm antibodies on subsequent fertility. Br J Urol 53(6):654-659
105. Holman CD, Wisniewski ZS, Semmens JB, Rouse IL, Bass AJ 2000 Population-based outcomes after 28,246 in-hospital vasectomies and 1,902 vasovasostomies in Western Australia. BJU Int 86(9):1043-1049
106. Silber SJ, Grotjan HE 2004 Microscopic vasectomy reversal 30 years later: a summary of 4010 cases by the same surgeon. J Androl 25(6):845-859
107. Hsieh MH, Meng MV, Turek PJ 2007 Markov modeling of vasectomy reversal and ART for infertility: how do obstructive interval and female partner age influence cost effectiveness? Fertil Steril 88(4):840-846
108. Namekawa T, Imamoto T, Kato M, Komiya A, Ichikawa T 2018 Vasovasostomy and vasoepididymostomy: Review of the procedures, outcomes, and predictors of patency and pregnancy over the last decade. Reprod Med Biol 17(4):343-355
109. Kuang W, Shin PR, Oder M, Thomas AJ, Jr. 2005 Robotic-assisted vasovasostomy: a two-layer technique in an animal model. Urology 65(4):811-814
110. Schiff J, Li PS, Goldstein M 2005 Robotic microsurgical vasovasostomy and vasoepididymostomy in rats. Int J Med Robot 1(2):122-126
111. Chan P, Parekattil SJ, Goldstein M, Lipshultz LI, Kavoussi P, McCullough A, Sigman M 2018 Pros and cons of robotic microsurgery as an appropriate approach to male reproductive surgery for vasectomy reversal and varicocele repair. Fertil Steril 110(5):816-823
112. Scovell JM, Mata DA, Ramasamy R, Herrel LA, Hsiao W, Lipshultz LI 2015 Association between the presence of sperm in the vasal fluid during vasectomy reversal and postoperative patency: a systematic review and meta-analysis. Urology 85(4):809-813
113. Witt MA, Heron S, Lipshultz LI 1994 The post-vasectomy length of the testicular vasal remnant: a predictor of surgical outcome in microscopic vasectomy reversal. J Urol 151(4):892-894
114. Labrecque M, Hoang DQ, Turcot L 2003 Association between the length of the vas deferens excised during vasectomy and the risk of postvasectomy recanalization. Fertil Steril 79(4):1003-1007
115. Gerrard ER, Jr., Sandlow JI, Oster RA, Burns JR, Box LC, Kolettis PN 2007 Effect of female partner age on pregnancy rates after vasectomy reversal. Fertil Steril 87(6):1340-1344
116. Shiraishi K, Takihara H, Naito K 2003 Quantitative analysis of testicular interstitial fibrosis after vasectomy in humans. Aktuelle Urol 34(4):262-264
117. Raleigh D, O'Donnell L, Southwick GJ, De Kretser DM, McLachlan RI 2004 Stereological analysis of the human testis after vasectomy: impairment of spermatogenic efficiency with increasing obstructive interval. Fertility and Sterility 81(6):1595-1603
118. Herrel LA, Goodman M, Goldstein M, Hsiao W 2015 Outcomes of microsurgical vasovasostomy for vasectomy reversal: a meta-analysis and systematic review. Urology 85(4):819-825
119. Silber SJ 1979 Epididymal extravasation following vasectomy as a cause for failure of vasectomy reversal. Fertility and Sterility 31(3):309-315
120. Andonian S, Jarvi K, Zini A, Hermo L 2002 Ultrastructural features of the vas deferens from patients undergoing vasectomy and vasectomy reversal. J Androl 23(5):691-701
121. Jarow JP, Budin RE, Dym M, Zirkin BR, Noren S, Marshall FF 1985 Quantitative pathologic changes in the human testis after vasectomy. A controlled study. N Engl J Med 313(20):1252-1256
122. Shiraishi K, Takihara H, Naito K 2002 Influence of interstitial fibrosis on spermatogenesis after vasectomy and vasovasostomy. Contraception 65(3):245-249
123. Zhang Y, Wang X, Chen Z, Huang X 2012 Long-term reproductive consequences of no-scalpel vasectomy in beagles. J Huazhong Univ Sci Technolog Med Sci 32(6):899-905
124. Silber SJ, Barbey N, Lenahan K, Silber DZ 2013 Applying clinically proven human techniques for contraception and fertility to endangered species and zoo animals: a review. J Zoo Wildl Med 44(4 Suppl):S111-122
125. Pavlovich CP, Schlegel PN 1997 Fertility options after vasectomy: a cost-effectiveness analysis. Fertility and Sterility 67(1):133-141
126. Heidenreich A, Altmann P, Engelmann UH 2000 Microsurgical vasovasostomy versus microsurgical epididymal sperm aspiration/testicular extraction of sperm combined with intracytoplasmic sperm injection. A cost-benefit analysis. European Urology 37(5):609-614
127. Samplaski MK, Daniel A, Jarvi K 2014 Vasectomy as a reversible form of contraception for select patients. Can J Urol 21(2):7234-7240
128. Sharma V, Zargaroff S, Sheth KR, Le BV, Dupree JM, Sandlow JI, Polackwich AS, Hedges JC, Fuchs EF, Goldstein M, Brannigan RE 2014 Relating economic conditions to vasectomy and vasectomy reversal frequencies: a multi-institutional study. J Urol 191(6):1835-1840
129. Zhang GY, Wang XH, Chen ZW, Cui YG 1997 Research on male contraception in China. In: Waites GMH, Frick J, Baker GWH eds. Current Advances in Andrology. Bologna: Monduzzi Editore; 233-239
130. Zhao SC 1990 Vas deferens occlusion by percutaneous injection of polyurethane elastomer plugs: clinical experience and reversibility. Contraception 41(5):453-459
131. Soebadi DM, Gardjito W, Mensink HJ 1995 Intravasal injection of formed-in-place medical grade silicone rubber for vas occlusion. Int J Androl 18 Suppl 1(45-52
132. Zambon JV, Barone MA, Pollack AE, Mehta M 2000 Efficacy of percutaneous vas occlusion compared with conventional vasectomy. BJU Int 86(6):699-705; discussion 705-696
133. Song L, Gu Y, Lu W, Liang X, Chen Z 2006 A phase II randomized controlled trial of a novel male contraception, an intra-vas device. Int J Androl 29(4):489-495
134. Ansari AS, Hussain M, Khan SR, Lohiya NK 2016 Relative suitability of DMSO and NaHCO3 for reversal of RISUG(R) induced long-term contraception. Andrology 4(2):306-313
135. Guha SK, Singh G, Ansari S, Kumar S, Srivastava A, Koul V, Das HC, Malhotra RL, Das SK 1997 Phase II clinical trial of a vas deferens injectable contraceptive for the male. Contraception 56(4):245-250
136. Guha SK 2007 Biophysical mechanism-mediated time-dependent effect on sperm of human and monkey vas implanted polyelectrolyte contraceptive. Asian J Androl 9(2):221-227
137. Roy S, Ghosh D, Guha SK 2009 Polyelectrolyte polymer properties in relation to male contraceptive RISUG action. Colloids Surf B Biointerfaces 69(1):77-84
138. Sharma RS, Mathur AK, Singh R, Das HC, Singh GJ, Toor DPS, Guha SK 2019 Safety & efficacy of an intravasal, one-time injectable & non-hormonal male contraceptive (RISUG): A clinical experience. Indian J Med Res 150(1):81-86
139. Lu WH, Liang XW, Gu YQ, Wu WX, Bo LW, Zheng TG, Chen ZW 2014 A randomized, controlled, multicenter contraceptive efficacy clinical trial of the intravas device, a nonocclusive surgical male sterilization. Asian J Androl 16(3):432-436
140. Wang ZQ, Liu ZQ, Zhao CH, Zhang K, Kang ZJ, Qu TR, Zeng FS, Guo PY, Tong ZC, Wang CL, Wang KL, Wang HL, Xu YS, Wang WH, Chu ML, Wang L, Qiao ZY, Wang H, Xu W 2022 An Ultrasound-Induced Self-Clearance Hydrogel for Male Reversible Contraception. ACS Nano
141. Lohiya NK, Alam I, Hussain M, Khan SR, Ansari AS 2014 RISUG: an intravasal injectable male contraceptive. Indian J Med Res 140 Suppl(S63-72
142. Moore CR, Oslund R 1924 Experiments on the sheep testis - cryptorchidism, vasectomy and scrotal insulation. American Journal of Physiology 67(595-607
143. Kandeel FR, Swerdloff RS 1988 Role of temperature in regulation of spermatogenesis and the use of heating as a method for contraception. Fertility and Sterility 49(1):1-23
144. Thonneau P, Bujan L, Multigner L, Mieusset R 1998 Occupational heat exposure and male fertility: a review. Hum Reprod 13(8):2122-2125
145. Ding W, Chen Z, Gu Y, Chen Z, Zheng Y, Sun F 2021 Magnetic Testis Targeting and Magnetic Hyperthermia for Noninvasive, Controllable Male Contraception via Intravenous Administration. Nano Lett 21(14):6289-6297
146. Mieusset R, Bujan L 1994 The potential of mild testicular heating as a safe, effective and reversible contraceptive method for men. Int J Androl 17(4):186-191
147. Wang C, McDonald V, Leung A, Superlano L, Berman N, Hull L, Swerdloff RS 1997 Effect of increased scrotal temperature on sperm production in normal men. Fertility and Sterility 68(2):334-339
148. Joubert S, Tcherdukian J, Mieusset R, Perrin J 2022 Thermal male contraception: A study of users' motivation, experience, and satisfaction. Andrology 10(8):1500-1510
149. Amouroux M, Mieusset R, Desbriere R, Opinel P, Karsenty G, Paci M, Fernandes S, Courbiere B, Perrin J 2018 Are men ready to use thermal male contraception? Acceptability in two French populations: New fathers and new providers. PLoS One 13(5):e0195824
150. Setchell BP 1998 The Parkes Lecture. Heat and the testis. J Reprod Fertil 114(2):179-194
151. Baskin MJ 1932 Temporary sterilization by injection of human spermatozoa: a preliminary report. American Journal of Obstetrics and Gynecology 24(892-897
152. Naz RK 2011 Antisperm contraceptive vaccines: where we are and where we are going? Am J Reprod Immunol 66(1):5-12
153. Mortazavi B, Allahyari Fard N, Karkhane AA, Shokrpoor S, Heidari F 2021 Evaluation of multi-epitope recombinant protein as a candidate for a contraceptive vaccine. J Reprod Immunol 145(103325
154. Naz RK, Rajesh C 2004 Passive immunization for immunocontraception: lessons learned from infectious diseases. Front Biosci 9(2457-2465
155. Naz RK 2009 Development of genetically engineered human sperm immunocontraceptives. J Reprod Immunol 83(1-2):145-150
156. Barfield JP, Nieschlag E, Cooper TG 2006 Fertility control in wildlife: humans as a model. Contraception 73(1):6-22
157. McLaughlin EA, Aitken RJ 2011 Is there a role for immunocontraception? Mol Cell Endocrinol 335(1):78-88
158. Vickery BH, Grigg MB, Goodpasture JC, Bergstrom KK, Walker KAM 1986 Towards a same-day, orally administered male contraceptive. In: Zatuchni GI, Goldsmith A, Spieler JM, Sciarra JJ eds. Male Contraception. Advances and Future Prospects. Philadelphia: Harper & Row; 271-292
159. Giwercman A, Skakkebaek NE 1986 The effect of salicylazosulphapyridine (sulphasalazine) on male fertility. A review. International Journal of Andrology 9(38-52
160. Kjaergaard N, Kjaergaard B, Lauritsen JG 1988 Prazosin, an adrenergic blocking agent inadequate as male contraceptive pill. Contraception 37(6):621-629
161. van der Spoel AC, Jeyakumar M, Butters TD, Charlton HM, Moore HD, Dwek RA, Platt FM 2002 Reversible infertility in male mice after oral administration of alkylated imino sugars: a nonhormonal approach to male contraception. Proc Natl Acad Sci U S A 99(26):17173-17178
162. Wu D 1989 An overview of the clinical pharmacology and therapeutic potential of goosypol as a male contraceptive agent and in gynaecological disease. Drugs 38(3):333-341
163. He X, Wu C, Cui Y, Zhu H, Gao Z, Li B, Hua J, Zhao B 2017 The aldehyde group of gossypol induces mitochondrial apoptosis via ROS-SIRT1-p53-PUMA pathway in male germline stem cell. Oncotarget 8(59):100128-100140
164. Waites GMH, Wang C, Griffin PD 1998 Gossypol: reasons for its failure to be accepted as a safe, reversible male antifertility agent. International Journal of Andrology 21(8-12
165. Xiong J, Wang H, Guo G, Wang S, He L, Chen H, Wu J 2011 Male germ cell apoptosis and epigenetic histone modification induced by Tripterygium wilfordii Hook F. PLoS One 6(6):e20751
166. Ni B, Jiang Z, Huang X, Xu F, Zhang R, Zhang Z, Tian Y, Wang T, Zhu T, Liu J, Zhang L 2008 Male reproductive toxicity and toxicokinetics of triptolide in rats. Arzneimittelforschung 58(12):673-680
167. Huynh PN, Hikim AP, Wang C, Stefonovic K, Lue YH, Leung A, Atienza V, Baravarian S, Reutrakul V, Swerdloff RS 2000 Long-term effects of triptolide on spermatogenesis, epididymal sperm function, and fertility in male rats. J Androl 21(5):689-699
168. Chen SR, Batool A, Wang YQ, Hao XX, Chang CS, Cheng CY, Liu YX 2016 The control of male fertility by spermatid-specific factors: searching for contraceptive targets from spermatozoon's head to tail. Cell Death Dis 7(11):e2472
169. Kumar A, Majhi RK, Swain N, Giri SC, Kar S, Samanta L, Goswami C 2016 TRPV4 is endogenously expressed in vertebrate spermatozoa and regulates intracellular calcium in human sperm. Biochem Biophys Res Commun 473(4):781-788
170. Matzuk MM, Lamb DJ 2008 The biology of infertility: research advances and clinical challenges. Nat Med 14(11):1197-1213
171. Hogarth CA, Amory JK, Griswold MD 2011 Inhibiting vitamin A metabolism as an approach to male contraception. Trends Endocrinol Metab 22(4):136-144
172. Chung SS, Wang X, Roberts SS, Griffey SM, Reczek PR, Wolgemuth DJ 2011 Oral administration of a retinoic Acid receptor antagonist reversibly inhibits spermatogenesis in mice. Endocrinology 152(6):2492-2502
173. Wolgemuth DJ, Chung SS 2007 Retinoid signaling during spermatogenesis as revealed by genetic and metabolic manipulations of retinoic acid receptor alpha. Soc Reprod Fertil Suppl 63(11-23
174. Noman MAA, Kyzer JL, Chung SSW, Wolgemuth DJ, Georg GI 2020 Retinoic acid receptor antagonists for male contraception: current status†. Biol Reprod 103(2):390-399
175. Tash JS, Chakrasali R, Jakkaraj SR, Hughes J, Smith SK, Hornbaker K, Heckert LL, Ozturk SB, Hadden MK, Kinzy TG, Blagg BS, Georg GI 2008 Gamendazole, an orally active indazole carboxylic acid male contraceptive agent, targets HSP90AB1 (HSP90BETA) and EEF1A1 (eEF1A), and stimulates Il1a transcription in rat Sertoli cells. Biology of Reproduction 78(6):1139-1152
176. Tash JS, Attardi B, Hild SA, Chakrasali R, Jakkaraj SR, Georg GI 2008 A novel potent indazole carboxylic acid derivative blocks spermatogenesis and is contraceptive in rats after a single oral dose. Biology of Reproduction 78(6):1127-1138
177. Mok KW, Mruk DD, Lie PP, Lui WY, Cheng CY 2011 Adjudin, a potential male contraceptive, exerts its effects locally in the seminiferous epithelium of mammalian testes. Reproduction 141(5):571-580
178. Pozor MA, Macpherson ML, McDonnell SM, Nollin M, Roser JF, Love C, Runyon S, Thomas BF, Troedsson MH 2013 Indenopyride derivative RTI-4587-073(l): a candidate for male contraception in stallions. Theriogenology 80(9):1006-1016
179. Silva JV, Freitas MJ, Santiago J, Jones S, Guimarães S, Vijayaraghavan S, Publicover S, Colombo G, Howl J, Fardilha M 2021 Disruption of protein phosphatase 1 complexes with the use of bioportides as a novel approach to target sperm motility. Fertil Steril 115(2):348-362
180. Faber EB, Wang N, Georg GI 2020 Review of rationale and progress toward targeting cyclin-dependent kinase 2 (CDK2) for male contraception†. Biol Reprod 103(2):357-367
181. Wisniewski A, Georg GI 2020 BET proteins: Investigating BRDT as a potential target for male contraception. Bioorg Med Chem Lett 30(6):126958
182. Crapster JA, Rack PG, Hellmann ZJ, Le AD, Adams CM, Leib RD, Elias JE, Perrino J, Behr B, Li Y, Lin J, Zeng H, Chen JK 2020 HIPK4 is essential for murine spermiogenesis. Elife 9(
183. Arifuzzaman S, Rahman MS, Pang MG 2019 Research update and opportunity of non-hormonal male contraception: Histone demethylase KDM5B-based targeting. Pharmacol Res 141(1-20
184. Ford WCL, Waites GMH 1978 Chlorinated sugars: a biochemical approach to the control of male fertility. International Journal of Andrology Suppl2(541-564
185. Suganuma R, Walden CM, Butters TD, Platt FM, Dwek RA, Yanagimachi R, van der Spoel AC 2005 Alkylated imino sugars, reversible male infertility-inducing agents, do not affect the genetic integrity of male mouse germ cells during short-term treatment despite induction of sperm deformities. Biol Reprod 72(4):805-813
186. Bone W, Walden CM, Fritsch M, Voigtmann U, Leifke E, Gottwald U, Boomkamp S, Platt FM, van der Spoel AC 2007 The sensitivity of murine spermiogenesis to miglustat is a quantitative trait: a pharmacogenetic study. Reprod Biol Endocrinol 5(1
187. Amory JK, Muller CH, Page ST, Leifke E, Pagel ER, Bhandari A, Subramanyam B, Bone W, Radlmaier A, Bremner WJ 2007 Miglustat has no apparent effect on spermatogenesis in normal men. Hum Reprod 22(3):702-707
188. Sipila P, Jalkanen J, Huhtaniemi IT, Poutanen M 2009 Novel epididymal proteins as targets for the development of post-testicular male contraception. Reproduction 137(3):379-389
189. Hinton BT, Cooper TG 2010 The epididymis as a target for male contraceptive development. Handb Exp Pharmacol198):117-137
190. Silva EJ, Patrao MT, Tsuruta JK, O'Rand MG, Avellar MC 2012 Epididymal protease inhibitor (EPPIN) is differentially expressed in the male rat reproductive tract and immunolocalized in maturing spermatozoa. Mol Reprod Dev 79(12):832-842
191. O'Rand MG, Silva EJ, Hamil KG 2016 Non-hormonal male contraception: A review and development of an Eppin based contraceptive. Pharmacol Ther 157(105-111
192. Mariani NAP, Camara AC, Silva AAS, Raimundo TRF, Andrade JJ, Andrade AD, Rossini BC, Marino CL, Kushima H, Santos LD, Silva EJR 2020 Epididymal protease inhibitor (EPPIN) is a protein hub for seminal vesicle-secreted protein SVS2 binding in mouse spermatozoa. Mol Cell Endocrinol 506(110754
193. Dey S, Brothag C, Vijayaraghavan S 2019 Signaling Enzymes Required for Sperm Maturation and Fertilization in Mammals. Front Cell Dev Biol 7(341
194. Mulryan K, Gitterman DP, Lewis CJ, Vial C, Leckie BJ, Cobb AL, Brown JE, Conley EC, Buell G, Pritchard CA, Evans RJ 2000 Reduced vas deferens contraction and male infertility in mice lacking P2X1 receptors. Nature 403(6765):86-89
195. White CW, Choong YT, Short JL, Exintaris B, Malone DT, Allen AM, Evans RJ, Ventura S 2013 Male contraception via simultaneous knockout of alpha1A-adrenoceptors and P2X1-purinoceptors in mice. Proc Natl Acad Sci U S A 110(51):20825-20830
196. Mathiew M, Dennis BM, Bennetts F, Su NNE, Nguyen N, Botteon A, Baell JB, Ventura S 2020 Synthesis of 2-phenyl-5,6,7,8-tetrahydroquinoxaline derivatives and screening for P2X1-purinoceptor antagonist activity in isolated preparations of rat vas deferens, for translation into a male contraceptive†. Biol Reprod 103(2):323-332
197. Khera N, Ghayor C, Lindholm AK, Pavlova E, Atanassova N, Weber FE 2020 Reversible Contraceptive Potential of FDA Approved Excipient N, N-Dimethylacetamide in Male Rats. Front Physiol 11(601084
198. Suarez SS 2008 Control of hyperactivation in sperm. Hum Reprod Update 14(6):647-657
199. Oberheide K, Puchkov D, Jentsch TJ 2017 Loss of the Na(+)/H(+) exchanger NHE8 causes male infertility in mice by disrupting acrosome formation. J Biol Chem 292(26):10845-10854
200. Lishko P, Kirichok Y, Ren D, Navarro B, Chung JJ, Clapham DE 2011 The Control of Male Fertility by Spermatozoan Ion Channels. Annu Rev Physiol
201. Goyal S, Manivannan B, Kumraj GR, Ansari AS, Lohiya NK 2013 Evaluation of efficacy and safety of recombinant sperm-specific contraceptive vaccine in albino mice. Am J Reprod Immunol 69(5):495-508
202. Nayyab S, Gervasi MG, Tourzani DA, Caraballo DA, Jha KN, Teves ME, Cui W, Georg GI, Visconti PE, Salicioni AM 2021 TSSK3, a novel target for male contraception, is required for spermiogenesis. Mol Reprod Dev 88(11):718-730
203. Salicioni AM, Gervasi MG, Sosnik J, Tourzani DA, Nayyab S, Caraballo DA, Visconti PE 2020 Testis-specific serine kinase protein family in male fertility and as targets for non-hormonal male contraception†. Biol Reprod 103(2):264-274
204. Breton S, Smith PJ, Lui B, Brown D 1996 Acidification of the male reproductive tract by a proton pumping (H+)-ATPase. Nature Medicine 2(4):470-472
205. Ren D, Navarro B, Perez G, Jackson A, Hsu S, Shi Q, Tilly J, Clapham D 2001 A sperm ion channel required for sperm motility and male fertility. Nature 413(6856):603-609
206. Esposito G, Jaiswal BS, Xie F, Krajnc-Franken MA, Robben TJ, Strik AM, Kuil C, Philipsen RL, van Duin M, Conti M, Gossen JA 2004 Mice deficient for soluble adenylyl cyclase are infertile because of a severe sperm-motility defect. Proc Natl Acad Sci U S A 101(9):2993-2998
207. Xie F, Garcia MA, Carlson AE, Schuh SM, Babcock DF, Jaiswal BS, Gossen JA, Esposito G, van Duin M, Conti M 2006 Soluble adenylyl cyclase (sAC) is indispensable for sperm function and fertilization. Dev Biol 296(2):353-362
208. Sanchez G, Nguyen AN, Timmerberg B, Tash JS, Blanco G 2006 The Na,K-ATPase alpha4 isoform from humans has distinct enzymatic properties and is important for sperm motility. Mol Hum Reprod 12(9):565-576
209. Zheng LP, Wang HF, Li BM, Zeng XH 2013 Sperm-specific ion channels: targets holding the most potential for male contraceptives in development. Contraception 88(4):485-491
210. Syeda SS, Sánchez G, Hong KH, Hawkinson JE, Georg GI, Blanco G 2018 Design, Synthesis, and in Vitro and in Vivo Evaluation of Ouabain Analogues as Potent and Selective Na,K-ATPase α4 Isoform Inhibitors for Male Contraception. J Med Chem 61(5):1800-1820
211. Syeda SS, Sánchez G, McDermott JP, Hong KH, Blanco G, Georg GI 2020 The Na+ and K+ transport system of sperm (ATP1A4) is essential for male fertility and an attractive target for male contraception†. Biol Reprod 103(2):343-356
212. Strunker T, Goodwin N, Brenker C, Kashikar ND, Weyand I, Seifert R, Kaupp UB 2011 The CatSper channel mediates progesterone-induced Ca2+ influx in human sperm. Nature 471(7338):382-386
213. Lishko PV, Botchkina IL, Kirichok Y 2011 Progesterone activates the principal Ca2+ channel of human sperm. Nature 471(7338):387-391
214. Singh AP, Rajender S 2015 CatSper channel, sperm function and male fertility. Reprod Biomed Online 30(1):28-38
215. Correia J, Michelangeli F, Publicover S 2015 Regulation and roles of Ca2+ stores in human sperm. Reproduction 150(2):R65-76
216. Sun XH, Zhu YY, Wang L, Liu HL, Ling Y, Li ZL, Sun LB 2017 The Catsper channel and its roles in male fertility: a systematic review. Reprod Biol Endocrinol 15(1):65
217. Ren D, Xia J 2010 Calcium signaling through CatSper channels in mammalian fertilization. Physiology (Bethesda) 25(3):165-175
218. Li H, Ding X, Guo C, Guan H, Xiong C 2012 Immunization of male mice with B-cell epitopes in transmembrane domains of CatSper1 inhibits fertility. Fertil Steril 97(2):445-452
219. Yu Q, Mei XQ, Ding XF, Dong TT, Dong WW, Li HG 2015 Construction of a catsper1 DNA vaccine and its antifertility effect on male mice. PLoS One 10(5):e0127508
220. Gruber FS, Johnston ZC, Barratt CL, Andrews PD 2020 A phenotypic screening platform utilising human spermatozoa identifies compounds with contraceptive activity. Elife 9(
221. Carlson EJ, Francis R, Liu Y, Li P, Lyon M, Santi CM, Hook DJ, Hawkinson JE, Georg GI 2022 Discovery and Characterization of Multiple Classes of Human CatSper Blockers. ChemMedChem 17(15):e202000499
222. Ye Q, Belabed H, Wang Y, Yu Z, Palaniappan M, Li JY, Kalovidouris SA, MacKenzie KR, Teng M, Young DW, Fujihara Y, Matzuk MM 2022 Advancing ASMS with LC-MS/MS for the discovery of novel PDCL2 ligands from DNA-encoded chemical library selections. Andrology
223. Shunnarah A, Tumlinson R, Calderón AI 2021 Natural Products with Potential for Nonhormonal Male Contraception. J Nat Prod 84(10):2762-2774
224. Ghaffarilaleh V, Fisher D, Henkel R 2019 Carica papaya seed extract slows human sperm. J Ethnopharmacol 241(111972
225. Adewole KE, Attah AF 2019 Antimalarial plants with potential male-factor antifertility properties. J Complement Integr Med 17(2)
226. Chauhan A, Thaper D, Prabha V 2020 Sperm impairing microbial factor: potential candidate for male contraception. Reprod Biol Endocrinol 18(1):96
227. Xu Y, Liu R, Leu NA, Zhang L, Ibragmova I, Schultz DC, Wang PJ 2020 A cell-based high-content screen identifies isocotoin as a small molecule inhibitor of the meiosis-specific MEIOB-SPATA22 complex†. Biol Reprod 103(2):333-342
228. Kamischke A, Nieschlag E 2004 Progress towards hormonal male contraception. Trends Pharmacol Sci 25(1):49-57
229. Aaltonen P, Amory JK, Anderson RA, Behre HM, Bialy G, Blithe D, Bone W, Bremner WJ, Colvard D, Cooper TG, Elliesen J, Gabelnick HL, Gu YQ, Handelsman DJ, Johansson EA, Kersemaekers W, Liu P, MacKay T, Matlin S, Mbizvo M, McLachlan RI, Meriggiola MC, Mletzko S, Mommers E, Muermans H, Nieschlag E, Odlind V, Page ST, Radlmaier A, Sitruk-Ware R, Swerdloff R, Wang C, Wu F, Zitzmann M 2007 10th Summit Meeting consensus: recommendations for regulatory approval for hormonal male contraception. J Androl 28(3):362-363
230. Pangkahila W 1991 Reversible azoospermia induced by an androgen-progestagen combination regimen in Indonesian men. International Journal of Andrology 44(248-256
231. World Health Organisation Task Force on Methods for the Regulation of Male Fertility 1993 Comparison of two androgens plus depot-medroxyprogesterone acetate for suppression to azoospermia in Indonesian men. Fertility and Sterility 60(1062-1068
232. Steiner MJ, Hertz-Picciotto I, Schulz KF, Sangi-Haghpeykar H, Earle BB, Trussell J 1998 Measuring true contraceptive efficacy. A randomized approach--condom vs. spermicide vs. no method. Contraception 58(6):375-378
233. Grimes DA, Lopez LM, Gallo MF, Halpern V, Nanda K, Schulz KF 2007 Steroid hormones for contraception in men. Cochrane Database Syst Rev2):CD004316
234. Liu PY, Swerdloff RS, Christenson PD, Handelsman DJ, Wang C, Hormonal Male Contraception Summit Group 2006 Rate, extent and modifiers of spermatogenic recovery after hormonal male contraception: an integrated analysis. Lancet 367(9520)(1412-1420.
235. Heinemann K, Saad F, Wiesemes M, White S, Heinemann L 2005 Attitudes toward male fertility control: results of a multinational survey on four continents. Hum Reprod 20(2):549-556
236. Heinemann K, Saad F, Wiesemes M, Heinemann LA 2005 Expectations toward a novel male fertility control method and potential user types: results of a multinational survey. J Androl 26(2):155-162
237. Martin CW, Anderson RA, Cheng L, Ho PC, van der Spuy Z, Smith KB, Glasier AF, Everington D, Baird DT 2000 Potential impact of hormonal male contraception: cross-cultural implications for development of novel preparations. Hum Reprod 15(3):637-645
238. Weston GC, Schlipalius ML, Bhuinneanin MN, Vollenhoven BJ 2002 Will Australian men use male hormonal contraception? Medical Journal of Australia 176(5):208-210
239. Roth MY, Shih G, Ilani N, Wang C, Page ST, Bremner WJ, Swerdloff RS, Sitruk-Ware R, Blithe DL, Amory JK 2014 Acceptability of a transdermal gel-based male hormonal contraceptive in a randomized controlled trial. Contraception 90(4):407-412
240. Zhang L, Shah IH, Liu Y, Vogelsong KM 2006 The acceptability of an injectable, once-a-month male contraceptive in China. Contraception 73(5):548-553
241. Glasier AF, Anakwe R, Everington D, Martin CW, van der Spuy Z, Cheng L, Ho PC, Anderson RA 2000 Would women trust their partners to use a male pill? Hum Reprod 15(3):646-649
242. Meriggiola MC, Cerpolini S, Bremner WJ, Mbizvo MT, Vogelsong KM, Martorana G, Pelusi G 2006 Acceptability of an injectable male contraceptive regimen of norethisterone enanthate and testosterone undecanoate for men. Hum Reprod 21(8):2033-2040
243. Amory JK, Page ST, Anawalt BD, Matsumoto AM, Bremner WJ 2007 Acceptability of a combination testosterone gel and depomedroxyprogesterone acetate male contraceptive regimen. Contraception 75(3):218-223
244. Hannaford PC, Iversen L, Macfarlane TV, Elliott AM, Angus V, Lee AJ 2010 Mortality among contraceptive pill users: cohort evidence from Royal College of General Practitioners' Oral Contraception Study. Bmj 340(c927
245. Waites GM 2003 Development of methods of male contraception: impact of the World Health Organization Task Force. Fertil Steril 80(1):1-15
246. Harper MJ 2008 Public-private partnerships advance contraceptive research and development. Contraception 78(4 Suppl):S36-41
247. Nieschlag E, Kumar N, Sitruk-Ware R 2013 7alpha-methyl-19-nortestosterone (MENTR): the population council's contribution to research on male contraception and treatment of hypogonadism. Contraception 87(3):288-295
248. Liu PY, McLachlan RI 2008 Male hormonal contraception: so near and yet so far. J Clin Endocrinol Metab 93(7):2474-2476
249. Nieschlag E 2013 Hormonal male contraception: end of a dream or start of a new era? Endocrine 43(3):535-538
250. Heckel NJ 1939 Production of oligospermia in a man by the use of testosterone propionate. Proceedings of the Society for Experimental Biology and Medicine 40(658-659
251. McCullagh EP, McGurl FJ 1940 The effects of testosterone propionate on epiphyseal closure, sodium and chloride balance and on sperm counts. Endocrinology 26(377-384
252. Heller CG, Nelson WO, Hill IB, Henderson E, Maddock WO, Jungck EC, Paulsen CA, Mortimore GE 1950 Improvement in spermatogenesis following depression of the human testis with testosterone. Fertility and Sterility 1(415-422
253. Heckel NJ, Rosso WA, Kestel L 1951 Spermatogenic rebound phenomenon after administration of testosterone propionate. Journal of Clinical Endocrinology and Metabolism 11(3):235-245
254. Reddy PRK, Rao JM 1972 Reversible antifertility action of testosterone propionate in human males. Contraception 5(4):295-301
255. Mauss J, Borsch G, Richter E, Bormacher K 1974 Investigations on the use of testosterone oenanthate as a male contraceptive agent. A preliminary report. Contraception 10(3):281-289
256. Patanelli DJ ed. 1977 Hormonal control of fertility. Washington: US Department of Health Education and Welfare,
257. Matsumoto AM 1990 Effects of chronic testosterone administration in normal men: safety and efficacy of high dosage testosterone and parallel dose-dependent suppression of luteinizing hormone, follicle-stimulating hormone and sperm production. Journal of Clinical Endocrinology and Metabolism 70(282-287
258. Cunningham GR, Silverman VE, Thornby J, Kohler PO 1979 The potential for an androgen male contraceptive. Journal of Clinical Endocrinology and Metabolism 49(520-526
259. Steinberger E, Smith KD, Rodriguez-Rigau LJ 1978 Suppression and recovery of sperm production in men treated with testosterone enanthate for one year. A study of a possible reversible male contraceptive. International Journal of Andrology Suppl 2(748-760
260. Swerdloff RS, Palacios A, McClure RD, Campfield LA, Brosman SA 1978 Male contraception: clinical assessment of chronic administration of testosterone enanthate. International Journal of Andrology Suppl 2(731-747
261. Paulsen CA 1978 Male contraceptive development: re-examination of testosterone enanthate as an effective single entity agent. In: Patanelli DJ ed. Hormonal Control of Male Fertility. Washington: Department of Health Education and Welfare; 17-40
262. Handelsman DJ, Conway AJ, Howe CJ, Turner L, Mackey MA 1996 Establishing the minimum effective dose and additive effects of depot progestin in suppression of human spermatogenesis by a testosterone depot. J Clin Endocrinol Metab 81(11):4113-4121
263. McLachlan RI, O'Donnell L, Meachem SJ, Stanton PG, de K, Pratis K, Robertson DM 2002 Hormonal regulation of spermatogenesis in primates and man: insights for development of the male hormonal contraceptive. J Androl 23(2):149-162
264. Nieschlag E, Zitzmann M, Kamischke A 2003 Use of progestins in male contraception. Steroids 68(10-13):965-972
265. McLachlan RI, Robertson DM, Pruysers E, Ugoni A, Matsumoto AM, Anawalt BD, Bremner WJ, Meriggiola C 2004 Relationship between serum gonadotropins and spermatogenic suppression in men undergoing steroidal contraceptive treatment. J Clin Endocrinol Metab 89(1):142-149
266. Matthiesson KL, McLachlan RI, O'Donnell L, Frydenberg M, Robertson DM, Stanton PG, Meachem SJ 2006 The relative roles of follicle-stimulating hormone and luteinizing hormone in maintaining spermatogonial maturation and spermiation in normal men. J Clin Endocrinol Metab 91(10):3962-3969
267. Weinbauer GF, Schlatt S, Walter V, Nieschlag E 2001 Testosterone-induced inhibition of spermatogenesis is more closely related to suppression of FSH than to testicular androgen levels in the cynomolgus monkey model (Macaca fascicularis). J Endocrinol 168(1):25-38
268. Narula A, Gu YQ, O'Donnell L, Stanton PG, Robertson DM, McLachlan RI, Bremner WJ 2002 Variability in sperm suppression during testosterone administration to adult monkeys is related to follicle stimulating hormone suppression and not to intratesticular androgens. J Clin Endocrinol Metab 87(7):3399-3406
269. Handelsman DJ, Farley TMM, Peregoudov A, Waites GMH, WHO Task Force On Methods For The Regulation Of Male Fertility 1995 Factors in nonuniform induction of azoospermia by testosterone enanthate in normal men. Fertility and Sterility 63(125-133
270. Santner S, Albertson B, Zhang GY, Zhang GH, Santulli M, Wang C, Demers LM, Shackleton C, Santen RJ 1998 Comparative rates of androgen production and metabolism in Caucasian and Chinese subjects. Journal of Clinical Endocrinology and Metabolism 83(2104-2109
271. Jin B, Turner L, Zhou Z, Zhou EL, Handelsman DJ 1999 Ethnicity and migration as determinants of human prostate size. Journal of Clinical Endocrinology and Metabolism 84(3613-3619
272. Yu B, Handelsman DJ 2001 Pharmacogenetic polymorphisms of the AR and metabolism and susceptibility to hormone- induced azoospermia. Journal of Clinical Endocrinology and Metabolism 86(9):4406-4411.
273. Eckardstein SV, Schmidt A, Kamischke A, Simoni M, Gromoll J, Nieschlag E 2002 CAG repeat length in the androgen receptor gene and gonadotrophin suppression influence the effectiveness of hormonal male contraception. Clin Endocrinol (Oxf) 57(5):647-655
274. Ilani N, Liu PY, Swerdloff RS, Wang C 2011 Does ethnicity matter in male hormonal contraceptive efficacy? Asian J Androl 13(4):579-584
275. Johnson L, Barnard JJ, Rodriguez L, Smith EC, Swerdloff RS, Wang XH, Wang C 1998 Ethnic differences in testicular structure and spermatogenic potential may predispose testes of Asian men to a heightened sensitivity to steroidal contraceptives. Journal of Andrology 19(348-357
276. Veldhuis JD, Bae A, Swerdloff RS, Iranmanesh A, Wang C 2005 Experimentally induced androgen depletion accentuates ethnicity-related contrasts in luteinizing hormone secretion in asian and caucasian men. J Clin Endocrinol Metab 90(3):1632-1638
277. Coviello AD, Bremner WJ, Matsumoto AM, Herbst KL, Amory JK, Anawalt BD, Yan X, Brown TR, Wright WW, Zirkin BR, Jarow JP 2004 Intratesticular testosterone concentrations comparable with serum levels are not sufficient to maintain normal sperm production in men receiving a hormonal contraceptive regimen. J Androl 25(6):931-938
278. Matthiesson KL, Amory JK, Berger R, Ugoni A, McLachlan RI, Bremner WJ 2004 Novel male hormonal contraceptive combinations: the hormonal and spermatogenic effects of testosterone and levonorgestrel combined with a 5alpha-reductase inhibitor or gonadotropin-releasing hormone antagonist. J Clin Endocrinol Metab
279. Page ST, Kalhorn TF, Bremner WJ, Anawalt BD, Matsumoto AM, Amory JK 2007 Intratesticular androgens and spermatogenesis during severe gonadotropin suppression induced by male hormonal contraceptive treatment. J Androl 28(5):734-741
280. Amory JK, Coviello AD, Page ST, Anawalt BD, Matsumoto AM, Bremner WJ 2007 Serum 17-hydroxyprogesterone strongly correlates with intratesticular testosterone in gonadotropin-suppressed normal men receiving various dosages of human chorionic gonadotropin. Fertil Steril
281. Amory JK, Page ST, Anawalt BD, Coviello AD, Matsumoto AM, Bremner WJ 2007 Elevated end-of-treatment serum INSL3 is associated with failure to completely suppress spermatogenesis in men receiving male hormonal contraception. J Androl 28(4):548-554
282. Ly LP, Liu PY, Handelsman DJ 2005 Rates of suppression and recovery of human sperm output in testosterone-based hormonal contraceptive regimens. Hum Reprod 20(6):1733-1740
283. Wu FCW, Farley TMM, Peregoudov A, Waites GMH, WHO Task Force on Methods for the Regulation of Male Fertility 1996 Effects of testosterone enanthate in normal men: experience from a multicenter contraceptive efficacy study. Fertility and Sterility 65(626-636
284. Mackey MA, Conway AJ, Handelsman DJ 1995 Tolerability of intramuscular injections of testosterone ester in an oil vehicle. Human Reproduction 10(862-865
285. McLachlan RI, O'Donnell L, Stanton PG, Balourdos G, Frydenberg M, de Kretser DM, Robertson DM 2002 Effects of testosterone plus medroxyprogesterone acetate on semen quality, reproductive hormones, and germ cell populations in normal young men. Journal of Clinical Endocrinology and Metabolism 87(2):546-556.
286. Meriggiola MC, Costantino A, Bremner WJ, Morselli-Labate AM 2002 Higher testosterone dose impairs sperm suppression induced by a combined androgen-progestin regimen. J Androl 23(5):684-690
287. Behre HM, Nieschlag E 1998 Comparative pharmacokinetics of testosterone esters. In: Nieschlag E, Behre HM eds. Testosterone: Action Deficiency Substitution. 2nd ed. Berlin: Springer; 329-348
288. Handelsman DJ, Conway AJ, Boylan LM 1990 Pharmacokinetics and pharmacodynamics of testosterone pellets in man. Journal of Clinical Endocrinology and Metabolism 71(216-222
289. Amory JK, Anawalt BD, Blaskovich PD, Gilchriest J, Nuwayser ES, Matsumoto AM 2002 Testosterone release from a subcutaneous, biodegradable microcapsule formulation (Viatrel) in hypogonadal men. Journal of Andrology 23(1):84-91
290. Behre HM, Baus S, Kliesch S, Keck C, Simoni M, Nieschlag E 1995 Potential of testosterone buciclate for male contraception: endocrine differences between responders and nonresponders. Journal of Clinical Endocrinology and Metabolism 80(2394-2403
291. Handelsman DJ, Conway AJ, Boylan LM 1992 Suppression of human spermatogenesis by testosterone implants in man. Journal of Clinical Endocrinology and Metabolism 75(1326-1332
292. McCullagh EP, Rossmiller HR 1941 Methyl testosterone. I androgenic effects and production of gynecomastia and oligospermia. Journal of Clinical Endocrinology and Metabolism 1(496-502)
293. Jones TM, Fang VS, Landau RL, Rosenfield RL 1977 The effects of fluoxymesterone administration on testicular function. Journal of Clinical Endocrinology and Metabolism 44(121-129
294. Holma PK 1977 Effects of an anabolic steroid (metandienone) on spermatogenesis. Contraception 15(2):151-162
295. Skoglund RD, Paulsen CA 1973 Danazol-testosterone combination: a potentially effective means for reversible male contraception. a preliminary report. Contraception 7(5):357-365
296. Sherins RJ, Gandy HM, Thorslund TW, Paulsen CA 1971 Pituitary and testicular function studies. I. Experience with a new gonadal inhibitor, 17a-pregn-4-en-20-yno-(2,3-d) isoxazol-17-ol (Danazol). Journal of Clinical Endocrinology and Metabolism 32(522-531
297. Ishak KG, Zimmerman HJ 1987 Hepatotoxic effects of the anabolic-androgenic steroids. Seminars in Liver Disease 7(3):230-236
298. Velazquez I, Alter BP 2004 Androgens and liver tumors: Fanconi's anemia and non-Fanconi's conditions. Am J Hematol 77(3):257-267
299. Knuth UA, Maniera H, Nieschlag E 1989 Anabolic steroids and semen parameters in bodybuilders. Fertility and Sterility 52(1041-1047
300. Knuth UA, Behre H, Belkien L, Bents H, Nieschlag E 1985 Clinical trial of 19-nortestosterone hexoxyphenylpropionate (Anadur) for male fertility regulation. Fertility and Sterility 44(6):814-821
301. Schurmeyer T, Knuth UA, Belkein L, Nieschlag E 1984 Reversible azoospermia induced by the anabolic steroid 19-nortestosterone. Lancet 1(417-420
302. Schellen TNCM, Beek JMJHA 1972 The influence of high doses of mesterolone on the spermiogram. Fertility and Sterility 23(712-714
303. Behre HM, Kliesch S, Lemcke B, von Eckardstein S, Nieschlag E 2001 Suppression of spermatogenesis to azoospermia by combined administration of GnRH antagonist and 19-nortestosterone cannot be maintained by this non-aromatizable androgen alone. Hum Reprod 16(12):2570-2577
304. Swerdloff RS, Bagatell CJ, Wang C, Anawalt BD, Berman N, Steiner B, Bremner WJ 1998 Suppression of spermatogenesis in man induced by Nal-Glu gonadotropin releasing hormone antagonist and testosterone enanthate (TE) in maintained by TE alone. Journal of Clinical Endocrinology and Metabolism 83(3527-3533
305. Sundaram K, Kumar N 2000 7alpha-methyl-19-nortestosterone (MENT): the optimal androgen for male contraception and replacement therapy. Int J Androl 23 Suppl 2(13-15
306. Cummings DE, Kumar N, Bardin CW, Sundaram K, Bremner WJ 1998 Prostate-sparing effects in primates of the potent androgen 7alpha-methyl-19-nortestosterone: a potential alternative to testosterone for androgen replacement and male contraception. Journal of Clinical Endocrinology and Metabolism 83(12):4212-4219
307. Anderson RA, Wallace AM, Sattar N, Kumar N, Sundaram K 2003 Evidence for tissue selectivity of the synthetic androgen 7 alpha-methyl-19-nortestosterone in hypogonadal men. J Clin Endocrinol Metab 88(6):2784-2793
308. Idan A, Griffiths KA, Harwood DT, Seibel MJ, Turner L, Conway AJ, Handelsman DJ 2010 Long-term effects of dihydrotestosterone treatment on prostate growth in healthy, middle-aged men without prostate disease: a randomized, placebo-controlled trial. Ann Intern Med 153(10):621-632
309. Finkelstein JS, Lee H, Burnett-Bowie SA, Pallais JC, Yu EW, Borges LF, Jones BF, Barry CV, Wulczyn KE, Thomas BJ, Leder BZ 2013 Gonadal steroids and body composition, strength, and sexual function in men. N Engl J Med 369(11):1011-1022
310. Sartorius GA, Ly LP, Handelsman DJ 2014 Male sexual function can be maintained without aromatization: randomized placebo-controlled trial of dihydrotestosterone (DHT) in healthy, older men for 24 months. J Sex Med 11(10):2562-2570
311. Avery MA, Tanabe M, Crowe DF, Detre G, Peters RH, Chong WKM 1990 Synthesis and testing of 17ab-hydroxy-7a methyl-D-homoestra-4,16-dien-3-one: a highly potent orally active androgen. Steroids 55(59-64
312. Grootenhuis AJ, de Gooyer ME, Louw J, Bursi R, Leysen D 2004 Synthesis and pharmacological profiling of new orally active steroidal androgens. In: Nieschlag E, Behre HM eds. Testosterone: Action, Deficiency and Substitution. 3rd ed. Berlin: Springer-Verlag; 665-684
313. Wu FC, von Eckardstein A 2003 Androgens and coronary artery disease. Endocrine Reviews 24(2):183-217
314. Liu PY, Death AK, Handelsman DJ 2003 Androgens and cardiovascular disease. Endocrine Reviews 24(3):313-340
315. Handelsman DJ, Conway AJ, Howe CJ, Turner L, Mackey MA 1996 Establishing the minimum effective dose and additive effects of depot progestin in suppression of human spermatogenesis by a testosterone depot. Journal of Clinical Endocrinology and Metabolism 81(4113-4121
316. Atkinson LE, Chang YL, Snyder PJ 1998 Long-term experience with testosterone replacement through scrotal skin. In: Nieschlag E, Behre HM eds. Testosterone: Action Deficiency Substitution. 2nd ed. Berlin: Springer; 364-388
317. Handelsman DJ 1998 The safety of androgens: prostate and cardiovascular disease. In: Wang C ed. Male Reproductive Function. Boston: Kluwer Academic Publishers; 173-190
318. Wu JP, Gu FL 1987 The prostate 41-65 years post castration. Chinese Medical Journal 100(271-272
319. Imperato-McGinley J, Gautier T, Zirinsky K, Hom T, Palomo O, Stein E, Vaughan ED, Markisz JA, deArellano ER, Kazam E 1992 Prostate visualization studies in males homozygous and heterozygous for 5-a reductase deficiency. Journal of Clinical Endocrinology and Metabolism 75(1022-1026
320. Quigley CA, DeBellis A, Marschke KB, El-Awady MK, Wilson EM, French FF 1995 Androgen receptor defects: historical, clinical and molecular perspectives. Endocrine Reviews 16(271-321
321. Roddam AW, Allen NE, Appleby P, Key TJ 2008 Endogenous sex hormones and prostate cancer: a collaborative analysis of 18 prospective studies. J Natl Cancer Inst 100(3):170-183
322. Zitzmann M, Depenbusch M, Gromoll J, Nieschlag E 2003 Prostate volume and growth in testosterone-substituted hypogonadal men are dependent on the CAG repeat polymorphism of the androgen receptor gene: a longitudinal pharmacogenetic study. J Clin Endocrinol Metab 88(5):2049-2054
323. Nelson KA, Witte JS 2002 Androgen receptor CAG repeats and prostate cancer. Am J Epidemiol 155(10):883-890
324. Behre HM, Bohmeyer J, Nieschlag E 1994 Prostate volume in testosterone-treated and untreated hypogonadal men in comparison to age-matched normal controls. Clinical Endocrinology 40(341-349
325. Meikle AW, Arver S, Dobs AS, Adolfsson J, Sanders SW, Middleton RG, Stephenson RA, Hoover DR, Rajaram L, Mazer NA 1997 Prostate size in hypogonadal men treated with a nonscrotal permeation-enhanced testosterone transdermal system. Urology 49(2):191-196
326. Jin B, Conway AJ, Handelsman DJ 2001 Effects of androgen deficiency and replacement on prostate zonal volumes. Clin Endocrinol (Oxf) 54(4):437-445
327. Jin B, Turner L, Walters WAW, Handelsman DJ 1996 Androgen or estrogen effects on the human prostate. Journal of Clinical Endocrinology and Metabolism 81(4290-4295
328. Archer J 1991 The influence of testosterone on human aggression. British Journal of Psychiatry 82(1-28
329. Anderson RA, Bancroft J, Wu FCW 1992 The effects of exogenous testosterone on sexuality and mood of normal men. Journal of Clinical Endocrinology and Metabolism 75(1503-1507
330. Christiansen K 1998 Behavioural correlates of testosterone. In: Nieschlag E, Behre HM eds. Testosterone: Action Deficiency Substitution. 2nd ed. Berlin: Springer; 107-142
331. O'Connor DB, Archer J, Wu FC 2004 Effects of testosterone on mood, aggression, and sexual behavior in young men: a double-blind, placebo-controlled, cross-over study. J Clin Endocrinol Metab 89(6):2837-2845
332. Pope HG, Jr., Kouri EM, Hudson JI 2000 Effects of supraphysiologic doses of testosterone on mood and aggression in normal men: a randomized controlled trial. Archives of General Psychiatry 57(2):133-140; discussion 155-136
333. Bahrke MS, Yesalis CE, Wright JE 1990 Psychological and behavioural effects of endogenous testosterone levels and anabolic-androgenic steroids among male. A review. Sports Medicine 10(5):303-337
334. Prasad MRN, Singh SP, Rajalakshmi M 1970 Fertility control in male rats by continuous release of microquantities of cyproterone acetate from subcutaneous silastic capsules. Contraception 2(165-178
335. Wang C, Yeung KK 1980 Use of low-dosage oral cyproterone acetate as a male contraceptive. Contraception 21(3):245-272
336. Chandolia RK, Weinbauer GF, Simoni M, Behre HM, Nieschlag E 1991 Comparative effects of chronic administration of the non-steroidal antiandrogens flutamide and casodex on the reproductive system of the male rat. Acta Endocr 125(547-555
337. Dhar JD, Sety BS 1990 Effect of a nonsteroidal antiandrogen, anadron, on the reproductive system and fertility in male rats. Contraception 42(1):121-138
338. McLachlan RI, McDonald J, Rushford D, Robertson DM, Garrett C, Baker HW 2000 Efficacy and acceptability of testosterone implants, alone or in combination with a 5alpha-reductase inhibitor, for male hormonal contraception. Contraception 62(2):73-78
339. Kinniburgh D, Anderson RA, Baird DT 2001 Suppression of spermatogenesis with desogestrel and testosterone pellets is not enhanced by addition of finasteride. J Androl 22(1):88-95
340. Killian J, Pratis K, Clifton RJ, Stanton PG, Robertson DM, O'Donnell L 2003 5alpha-reductase isoenzymes 1 and 2 in the rat testis during postnatal development. Biol Reprod 68(5):1711-1718
341. Amory JK, Kalhorn TF, Page ST 2007 Pharmacokinetics and Pharmacodynamics of Oral Testosterone Enanthate Plus Dutasteride for Four Weeks in Normal Men: Implications for Male Hormonal Contraception. J Androl
342. Bebb RA, Anawalt BD, Christensen RB, Paulsen CA, Bremner WJ, Matsumoto AM 1996 Combined administration of levonorgestrel and testosterone induces more rapid and effective suppression of spermatogenesis than testosterone alone: a promising male contraceptive approach. Journal of Clinical Endocrinology and Metabolism 81(757-762
343. Meriggiola MC, Bremner WJ, Paulsen CA, Valdiserri A, Incorvaia L, Motta R, Pavani A, Capelli M, Flamigni C 1996 A combined regimen of cyproterone acetate and testosterone enanthate as a potentially highly effective male contraceptive. Journal of Clinical Endocrinology and Metabolism 81(3018-3023
344. Bouchard P, Garcia E 1987 Influence of testosterone substitution on sperm suppression by LHRH agonists. Hormone Res 28(175-180
345. Behre HM, Nashan D, Hubert W, Nieschlag E 1992 Depot gonadotropin-releasing hormone agonist blunts the androgen-induced suppression of spermatogenesis in a clinical trial of male contraception. Journal of Clinical Endocrinology and Metabolism 74(84-90
346. Garcia MA, Meizel S 1999 Progesterone-mediated calcium influx and acrosome reaction of human spermatozoa: pharmacological investigation of T-type calcium channels. Biol Reprod 60(1):102-109
347. Losel RM, Falkenstein E, Feuring M, Schultz A, Tillmann HC, Rossol-Haseroth K, Wehling M 2003 Nongenomic steroid action: controversies, questions, and answers. Physiol Rev 83(3):965-1016
348. Luetjens CM, Didolkar A, Kliesch S, Paulus W, Jeibmann A, Bocker W, Nieschlag E, Simoni M 2006 Tissue expression of the nuclear progesterone receptor in male non-human primates and men. J Endocrinol 189(3):529-539
349. Heller CG, Moore DJ, Paulsen CA, Nelson WO, Laidlaw WM 1959 Effects of progesterone and synthetic progestins on the reproductive physiology of normal men. Federation Proceedings 18(1057-1064
350. Frick J, Danner C, Joos H, Kunit G, Luukkainen T 1981 Spermatogenesis in men treated with subcutaneous application of levonorgestrel and estrone rods. Journal of Andrology 2(331-338
351. Junaidi A, Luetjens CM, Wistuba J, Kamischke A, Yeung CH, Simoni M, Nieschlag E 2005 Norethisterone enanthate has neither a direct effect on the testis nor on the epididymis: a study in adult male cynomolgus monkeys (Macaca fascicularis). Eur J Endocrinol 152(4):655-661
352. Schearer SB, Alvarez-Sanchez F, Anselmo J, Brenner P, Coutinho E, Latham-Faundes A, Frick J, Heinild B, Johansson EDB 1978 Hormonal contraception for men. International Journal of Andrology (suppl 2)(680-712
353. Behre HM, Zitzmann M, Anderson RA, Handelsman DJ, Lestari SW, McLachlan RI, Meriggiola MC, Misro MM, Noe G, Wu FC, Festin MP, Habib NA, Vogelsong KM, Callahan MM, Linton KA, Colvard DS 2016 Efficacy and Safety of an Injectable Combination Hormonal Contraceptive for Men. J Clin Endocrinol Metab 101(12):4779-4788
354. Wu FCW, Aitken RJ 1989 Suppression of sperm function by depot medroxyprogesterone acetate and testosterone enanthate in steroid male contraception. Fertility and Sterility 51(691-698
355. Foegh M 1983 Evaluation of steroids as contraceptives in men. Acta Endocr suppl 260(1-48
356. Anawalt BD, Bebb RA, Bremner WJ, Matsumoto AM 1999 A lower dosage levonorgestrel and testosterone combination effectively suppresses spermatogenesis and circulating gonadotropin levels with fewer metabolic effects than higher dosage combinations. Journal of Andrology 20(3):407-414
357. Lobel B, Olivo JF, Guille F, Le Lannou D 1989 Contraception in men: efficacy and immediate toxicity. A study of 18 cases. Acta Urol Belg 57(1):117-124
358. Guerin JF, Rollet J 1988 Inhibition of Spermatogenesis in Men Using Various Combinations of Oral Progestagens and Percutaneous or Oral Androgens. International Journal of Andrology 11(3):187-199
359. Meriggiola MC, Bremner WJ, Constantino A, Di Cintio G, Flamigni C 1998 Low dose of cyproterone acetate and testosterone enanthate for contraception. Human Reproduction 13(1225-1229
360. Meriggiola MC, Pavani A, Bremner WJ, Capelli M, Constantino A, Flamigni C 1997 An oral regimen of cyproterone acetate and testosterone undecanoate for spermatogenic suppression in men. Fertility and Sterility 68(844-850
361. Gonzalo IT, Swerdloff RS, Nelson AL, Clevenger B, Garcia R, Berman N, Wang C 2002 Levonorgestrel implants (Norplant II) for male contraception clinical trials: combination with transdermal and injectable testosterone. Journal of Clinical Endocrinology and Metabolism 87(8):3562-3572.
362. Gui YL, He CH, Amory JK, Bremner WJ, Zheng EX, Yang J, Yang PJ, Gao ES 2004 Male hormonal contraception: suppression of spermatogenesis by injectable testosterone undecanoate alone or with levonorgestrel implants in chinese men. J Androl 25(5):720-727
363. Wang C, Wang XH, Nelson AL, Lee KK, Cui YG, Tong JS, Berman N, Lumbreras L, Leung A, Hull L, Desai S, Swerdloff RS 2006 Levonorgestrel implants enhanced the suppression of spermatogenesis by testosterone implants: comparison between Chinese and non-Chinese men. J Clin Endocrinol Metab 91(2):460-470
364. Anderson RA, Kinniburgh D, Baird DT 2002 Suppression of spermatogenesis by etonogestrel implants with depot testosterone: potential for long-acting male contraception. Journal of Clinical Endocrinology and Metabolism 87(8):3640-3649.
365. Brady BM, Amory JK, Perheentupa A, Zitzmann M, Hay CJ, Apter D, Anderson RA, Bremner WJ, Pollanen P, Nieschlag E, Wu FC, Kersemaekers WM 2006 A multicentre study investigating subcutaneous etonogestrel implants with injectable testosterone decanoate as a potential long-acting male contraceptive. Hum Reprod 21(1):285-294
366. Gu YQ, Tong JS, Ma DZ, Wang XH, Yuan D, Tang WH, Bremner WJ 2004 Male hormonal contraception: effects of injections of testosterone undecanoate and depot medroxyprogesterone acetate at eight-week intervals in chinese men. J Clin Endocrinol Metab 89(5):2254-2262
367. Page ST, Amory JK, Anawalt BD, Irwig MS, Brockenbrough AT, Matsumoto AM, Bremner WJ 2006 Testosterone gel combined with depomedroxyprogesterone acetate is an effective male hormonal contraceptive regimen and is not enhanced by the addition of a GnRH antagonist. J Clin Endocrinol Metab 91(11):4374-4380
368. Kamischke A, Heuermann T, Kruger K, von Eckardstein S, Schellschmidt I, Rubig A, Nieschlag E 2002 An effective hormonal male contraceptive using testosterone undecanoate with oral or injectable norethisterone preparations. Journal of Clinical Endocrinology and Metabolism 87(2):530-539
369. Meriggiola MC, Costantino A, Saad F, D'Emidio L, Morselli Labate AM, Bertaccini A, Bremner WJ, Rudolph I, Ernst M, Kirsch B, Martorana G, Pelusi G 2005 Norethisterone enanthate plus testosterone undecanoate for male contraception: effects of various injection intervals on spermatogenesis, reproductive hormones, testis, and prostate. J Clin Endocrinol Metab 90(4):2005-2014
370. Chao JH, Page ST 2016 The current state of male hormonal contraception. Pharmacol Ther 163(109-117
371. Zitzmann M, Rohayem J, Raidt J, Kliesch S, Kumar N, Sitruk-Ware R, Nieschlag E 2017 Impact of various progestins with or without transdermal testosterone on gonadotropin levels for non-invasive hormonal male contraception: a randomized clinical trial. Andrology 5(3):516-526
372. Attardi BJ, Engbring JA, Gropp D, Hild SA 2011 Development of dimethandrolone 17beta-undecanoate (DMAU) as an oral male hormonal contraceptive: induction of infertility and recovery of fertility in adult male rabbits. J Androl 32(5):530-540
373. Anawalt BD, Roth MY, Ceponis J, Surampudi V, Amory JK, Swerdloff RS, Liu PY, Dart C, Bremner WJ, Sitruk-Ware R, Kumar N, Blithe DL, Page ST, Wang C 2019 Combined nestorone-testosterone gel suppresses serum gonadotropins to concentrations associated with effective hormonal contraception in men. Andrology 7(6):878-887
374. Surampudi P, Page ST, Swerdloff RS, Nya-Ngatchou JJ, Liu PY, Amory JK, Leung A, Hull L, Blithe DL, Woo J, Bremner WJ, Wang C 2014 Single, escalating dose pharmacokinetics, safety and food effects of a new oral androgen dimethandrolone undecanoate in man: a prototype oral male hormonal contraceptive. Andrology 2(4):579-587
375. Thirumalai A, Ceponis J, Amory JK, Swerdloff R, Surampudi V, Liu PY, Bremner WJ, Harvey E, Blithe DL, Lee MS, Hull L, Wang C, Page ST 2018 Effects of 28 Days of Oral Dimethandrolone Undecanoate in Healthy Men: A Prototype Male Pill. J Clin Endocrinol Metab
376. Ayoub R, Page ST, Swerdloff RS, Liu PY, Amory JK, Leung A, Hull L, Blithe D, Christy A, Chao JH, Bremner WJ, Wang C 2017 Comparison of the single dose pharmacokinetics, pharmacodynamics, and safety of two novel oral formulations of dimethandrolone undecanoate (DMAU): a potential oral, male contraceptive. Andrology 5(2):278-285
377. Wu S, Yuen F, Swerdloff RS, Pak Y, Thirumalai A, Liu PY, Amory JK, Bai F, Hull L, Blithe DL, Anawalt BD, Parman T, Kim K, Lee MS, Bremner WJ, Page ST, Wang C 2018 Safety and Pharmacokinetics of Single Dose Novel Oral Androgen 11beta-Methyl-19-Nortestosterone-17beta-dodecylcarbonate in Men(a). J Clin Endocrinol Metab
378. Nguyen BT, Farrant MT, Anawalt BD, Yuen F, Thirumalai A, Amory JK, Swerdloff RS, Bremner WJ, Liu PY, Blithe DL, Page ST, Wang C 2020 Acceptability of oral dimethandrolone undecanoate in a 28-day placebo-controlled trial of a hormonal male contraceptive prototype. Contraception 102(1):52-57
379. Nguyen BT, Yuen F, Farrant M, Thirumalai A, Fernando F, Amory JK, Swerdloff RS, Anawalt BD, Blithe DL, Long JE, Liu PY, Page ST, Wang C 2021 Acceptability of the oral hormonal male contraceptive prototype, 11β-methyl-19-nortestosterone dodecylcarbonate (11β-MNTDC), in a 28-day placebo-controlled trial. Contraception 104(5):531-537
380. Ilani N, Roth MY, Amory JK, Swerdloff RS, Dart C, Page ST, Bremner WJ, Sitruk-Ware R, Kumar N, Blithe DL, Wang C 2012 A new combination of testosterone and nestorone transdermal gels for male hormonal contraception. J Clin Endocrinol Metab 97(10):3476-3486
381. Lobl TJ, Kirton KT, Forbes AD, Ewing LL, Kemp PL, Desjardins C 1983 Contraceptive efficacy of testosterone-estradiol implants in male rhesus monkeys. Contraception 27(4):383-389
382. Lobl TJ, Kirton KT, Forbes AD, Ewing LL, Kemp PL, Desjardins C 1983 Contraceptive efficacy of testosterone-estradiol implants in male rhesus monkeys. Contraception 27(4):383-389
383. Handelsman DJ, Wishart S, Mackey MA, Conway AJ 1998 Efficacy and safety of low dose estradiol supplementation to augment depot testosterone-induced suppression of human spermatogenesis. Annual Scientific Meeting of the Endocrine Society of Australia, Perth, 1998
384. Lunn SF, Dixson AF, Sandow J, Fraser HM 1990 Pituitary-testicular function is suppressed by an LHRH antagonist but not by an LHRH agonist in the marmoset monkey. Journal of Endocrinology 125(233-239
385. Marshall GF, Akhtar FB, Weinbauer GF, Nieschlag E 1986 Gonadotrophin-releasing hormone (GnRH) overcomes GnRH antagonist-induced suppression of LH secretion in primates. Journal of Endocrinology 110(145-150
386. Herbst KL, Coviello AD, Page S, Amory JK, Anawalt BD, Bremner WJ 2004 A single dose of the potent gonadotropin-releasing hormone antagonist acyline suppresses gonadotropins and testosterone for 2 weeks in healthy young men. J Clin Endocrinol Metab 89(12):5959-5965
387. Weinbauer GF, Surmann FJ, Nieschlag E 1987 Suppression of spermatogenesis in a non-human primate (Macaca fascicularis) by concomitant gonadotrophin-releasing hormone antagonist and testosterone treatment. Acta Endocr 114(138-146
388. Weinbauer GF, Khurshid S, Findscheidt U, Nieschlag E 1989 Sustained inhibition of sperm production and inhibin secretion by a gonadotrophin-releasing hormone antagonist and delayed testosterone substitution in non-human primates (Macaca fascicularis). Acta Endocr 123(303-310
389. Bremner WJ, Bagatell CJ, Steiner RA 1991 Gonadotropin-releasing hormone antagonist plus testosterone: a potential male contraceptive. Journal of Clinical Endocrinology and Metabolism 73(465-469
390. Tom L, Bhasin S, Salameh W, Steiner B, Peterson M, Sokol R, Rivier J, Vale WW, Swerdloff RS 1992 Induction of azoospermia in normal men with combined Nal-Glu GnRH antagonist and testosterone enanthate. Journal of Clinical Endocrinology and Metabolism 75(476-483
391. Pavlou SN, Brewer K, Farley MG, Lindner J, Bastias MC, Rogers BJ, Swift LL, Rivier JE, Vale WW, Conn PM, Herbert CM 1991 Combined administration of a gonadotropin-releasing hormone antagonist and testosterone in men induces reversible azoospermia without loss of libido. Journal of Clinical Endocrinology and Metabolism 73(1360-1369
392. Bagatell CJ, Matsumoto AM, Christensen RB, Rivier JE, Bremner WJ 1993 Comparison of a gonadotropin-releasing hormone antagonist plus testosterone (T) versus T alone as a potential male contraceptive. Journal of Clinical Endocrinology and Metabolism 77(427-432
393. Simms MS, Scholfield DP, Jacobs E, Michaeli D, Broome P, Humphreys JE, Bishop MC 2000 Anti-GnRH antibodies can induce castrate levels of testosterone in patients with advanced prostate cancer. Br J Cancer 83(4):443-446
394. Talwar GP 1997 Fertility regulating and immunotherapeutic vaccines reaching human trials stage. Human Reproduction Update 3(301-310
395. Naz RK 2005 Contraceptive vaccines. Drugs 65(5):593-603
396. Kirkpatrick JF, Lyda RO, Frank KM 2011 Contraceptive vaccines for wildlife: a review. Am J Reprod Immunol 66(1):40-50
397. Burger HG 1992 Inhibin. Reproductive Medicine Reviews 1(1-20
398. Wiebe JP, Wood PH 1987 Selective suppression of follicle-stimulating hormone by 3-alpha-hydroxy-4-pregnen-20-one, a steroid found in Sertoli cells. Endocrinology 120(2259-2264
399. Moudgal NR, Jeyakumar M, Krishnamurthy HN, Sridhar S, Krishnamurthy H 1997 Development of male contraceptive vaccine - a perspective. Human Reproduction Update 3(335-346
400. Xu C, Li YC, Yang H, Long Y, Chen MJ, Qin YF, Xia YK, Song L, Gu AH, Wang XR 2014 The preparation and application of N-terminal 57 amino acid protein of the follicle-stimulating hormone receptor as a candidate male contraceptive vaccine. Asian J Androl 16(4):623-630
401. Leng N, Grasso P, Reichert LE 1996 D-amino acid substitution of residues 32 to 46 of the glycoprotein hormone common alpha-subunit: development of a synthetic glycoprotein hormone antagonist. Peptide Research 9(188-194
402. Singh J, O'Neill C, Handelsman DJ 1995 Induction of spermatogenesis by androgens in gonadotropin-deficient (hpg) mice. Endocrinology 136(5311-5321
403. Kumar TR, Wang Y, Lu N, Matzuk MM 1997 FSH is required for ovarian follicle maturation but not for male fertility. Nature Genetics 15(201-204
404. Dierich A, Sairam MR, Monaco L, Fimia GM, Gansmuller A, LeMeur M, Sassone-Corsi P 1998 Impairing follicle-stimulating hormone (FSH) signalling in-vivo: targeted disruption of the FSH receptor leads to aberrant gametogenesis and hormonal imbalance. Proceedings of the National Academy of Sciences USA 95(13612-13617
405. Tapanainen JS, Aittomaki K, Min J, Vasivou T, Huhtaniemi IT 1997 Men homozygous for an inactivating mutation of the follicle-stimulating hormone (FSH) receptor present variable suppression of spermatogenesis and fertility. Nature Genetics 15(205-206
406. Nieschlag E 1986 Reasons for abandoning immunization against FSH as an approach to male fertility regulation. In: Zatuchni GI, Goldsmith A, Spieler JM, Sciarra JJ eds. Male Contraception: Advances and Future Prospects. Philadelphia: Harper & Row; 395-400
407. Trussell J 2004 Contraceptive failure in the United States. Contraception 70(2):89-96