ABSTRACT
The process of growth is complex and is influenced by various factors that act centrally and peripherally. In this chapter, we describe conditions associated with multiple pituitary hormone deficiency, isolated growth hormone deficiency, and abnormal growth without growth hormone deficiency, discuss the genes that are associated with these conditions, and prepare guidelines for the clinicians to evaluate a child with poor growth. In addition, we review treatment modalities, daily vs weekly, for growth hormone deficiency and their side effects.
INTRODUCTION
Human growth starts at conception and proceeds through various identifiable developmental stages. The process of growth depends on both genetic and environmental factors that combine to determine an individual’s eventual height. Genetic control of statural growth is becoming increasingly clear. Many genes have been identified that are required for normal development and function of the pituitary in general, and that control the growth hormone/insulin-like growth factor axis in particular and many more that are involved in numerous cascades of intracellular processes “downstream” of GH/IGF1 action. Mutations of these genes have been shown to be responsible for abnormal growth in humans and animals.
Growth hormone (GH) has been used to treat short children since the late 1950’s (1,2). Initially only those children with the most pronounced growth failure due to severe growth hormone deficiency (GHD) were considered appropriate candidates, but with time children with growth failure from a range of conditions have been shown to benefit from GH treatment. GH has also been used to treat several catabolic diseases including cystic fibrosis, inflammatory bowel disease and AIDS wasting (3–7). Here we review the physiology of growth, the diagnosis of GH deficiency, treatment options and genetic growth hormone disorders.
GROWTH DISORDERS
Growth failure may be due to genetic mutations, acquired disease and/or environmental deficiencies. Growth failure may result from a failure of hypothalamic growth hormone-releasing hormone (GHRH) production or release, from (genetic or sporadic) mal-development of the pituitary somatotropes, secondary to ongoing chronic illness, malnutrition, intrinsic abnormalities of cartilage and/or bone such as osteo-chondrodysplasias, and from genetic disorders affecting growth hormone production and responsiveness. Children without any identifiable cause of their growth failure are commonly labeled as having idiopathic short stature (ISS).
Genetic factors affecting growth include pituitary transcription factors (PROP1, POU1F1, HESX1, LHX3, and LHX4), GHRH, the GH secretagogue (GHS), GH, insulin like growth factor-1 (IGF1), insulin like growth factor-2 (IGF2), insulin (INS) and their receptors (GHRHR, GHSR, GHR, IGF1R, IGF2R and INSR) as well as transcription factors controlling GH signaling, including STAT1, STAT3, STAT5a, and STAT5b. Growth is also influenced by other factors such as the Short Stature Homeobox, sex steroids (estrogens and androgens), glucocorticoids and thyroid hormone.
Since the replacement of human pituitary-derived GH with recombinant human GH, much experience has been gained with the use of GH therapy. The Food and Drug Administration (FDA) had expanded GH use for the following conditions for children (8).
- GH deficiency/insufficiency
- Chronic renal insufficiency (pre-transplantation)
- Turner syndrome
- SHOX haploinsufficiency
- Short stature from Prader-Willi Syndrome (PWS)
- Children with a history of fetal growth restriction (SGA, IUGR) who have not caught up to a normal height range by age 2 years
- Children with idiopathic short stature (ISS): height > 2.25 SD below the mean in height and unlikely to catch up in height.
- Noonan Syndrome
- Short Bowel Syndrome
FDA approved conditions for GH treatment for adults:
- Adults with GH deficiency
- Adults with AIDS wasting
The efficacy of GH treatment has been investigated in children whose height has been compromised due to chronic illnesses such as Crohn’s disease, cystic fibrosis, glucocorticoid-induced suppression of growth in other disorders (asthma and juvenile idiopathic arthritis (JIA), also known as juvenile rheumatoid arthritis (JRA)), and adrenal steroid disorders such as congenital adrenal hyperplasia (CAH). Studies have shown both anabolic effects and improvement of growth velocity after GH treatment in children with glucocorticoid dependent Crohn’s disease (3,9,10). Improvement in linear growth has also been observed after GH treatment in children with cystic fibrosis and JIA (4–6). The same studies have shown significant improvement in weight gain and body composition, changes that have been variably correlated with improvement in life expectancy and quality of life.
The growth-suppressing effects of glucocorticoids are also seen in children affected with CAH where high androgens both increase short-term growth velocity and limit the height potential. Most patients with CAH complete their growth prematurely and are ultimately short adults. Lin-Su et al, showed that GH in combination with LHRHa significantly improved their final adult height in children with CAH (11).
Larger, long-term prospective studies are needed to determine the safety and efficacy of GH treatment in these populations of children.
The key mediator of GH action in the periphery for both prenatal and postnatal mammalian growth is IGF system. GH exerts its direct effects at the growth plate and indirect effects via IGF1. Better understanding the role of IGF1 on growth had led to the concept of IGF1 deficiency in addition to GH deficiency. With the introduction of recombinant human (rh) IGF1, today, it is possible to treat conditions due to genetic GH resistance or insensitivity caused by GH receptor defects, and the presence of neutralizing GH antibodies (12).
MULTIPLE PITUITARY HORMONE DEFICIENCY (MPHD)
GH deficiency may occur in combination with other pituitary hormone deficiencies and is often referred to as hypopituitarism, panhypopituitarism or multiple pituitary hormone deficiency (MPHD).
The anterior portion of pituitary gland forms from Rathke's pouch around the third week of gestation (13). It is influenced by the expression of numerous transcription factors and signaling molecules; some of them required for continued normal function of pituitary gland. Mutations have been identified in the genes encoding several pituitary transcription factors and signaling molecules, including GLI2, LHX3, LHX4, HESX1, PROP1, POU1F1, SOX2, PITX2, OTX2 and SOX3 (Table 1). The most frequently mutated gene is PROP1 (6.7% in sporadic and 48.5% in familial cases) (14).
Most cases of hypopituitarism are idiopathic in origin; however, familial inheritance, which may be either dominant or recessive, accounts for between 5 and 30% of all cases (15). It may present early in the neonatal period with symptoms of hypoglycemia, micropenis in males, prolonged jaundice, with/without midline defects or later in childhood with poor feeding, growth failure, or delayed puberty. It can be associated with single or multiple pituitary hormone deficiencies, and endocrinopathy. It may be associated with several other congenital anomalies such as optic nerve hypoplasia, anophthalmia, microphthalmia, agenesis of the corpus callosum, and absence of the septum pellucidum.
Table 1. Transcription Factors Required for Normal Pituitary Development
|
Transcription Factors
|
Function
|
GLI2
|
Essential for the forebrain and early stages of the anterior pituitary development
|
LHX3
|
Essential for the early development of the anterior pituitary, including the somatotrope, thyrotrope, lactotrope and the gonadotrope (but not the corticotrope)
|
LHX4
|
Essential for the proliferation of the anterior pituitary cell types, including the somatotrope, thyrotrope and the corticotrope
|
HESX1
|
Essential for the development of the anterior pituitary, including the somatotrope, thyrotrope, lactotrope and the gonadotrope
|
PROP1
|
Essential for the development of most cell types of the anterior pituitary, including the somatotrope, the thyrotrope, the lactotrope and the gonadotrope (but not the corticotrope). Also essential for the expression of the PIT1 protein and the extinction of HESX1 in the anterior pituitary
|
POU1F1 (PIT1)
|
Necessary for somatotrope, lactotrope and thyrotrope development and for their continued function
|
SOX2
|
Essential for the expression of POU1F1 and the development of the gonadotrope
|
PITX2
|
Necessary for the development of gonadotrope, somatotrope, lactotrope and thyrotrope
|
OTX2
|
Transactivates HESX1 and POU1F1
|
SOX3
|
Essential for the early formation of hypothalamic-pituitary axis
|
GLI2
GLI2 is a transcription factor, mediating Sonic Hedgehog (SHH) signaling and is necessary for forebrain development as well as for the early stages of pituitary development (16). It is located on the long arm of chromosome 2 at position q14, (Figure 1). The clinical phenotype of persons with mutations in GLI2 may vary from asymptomatic individuals to isolated GH deficiency to hypopituitarism in combination with a small anterior pituitary, ectopic posterior pituitary, midfacial hypoplasia, anophthalmia, holoprosencephaly, and polydactyly (17–19).
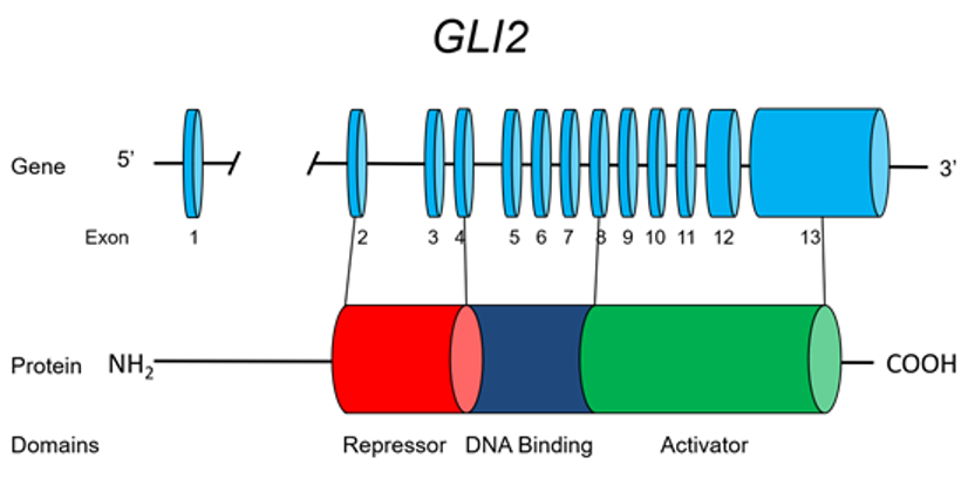
Figure 1. The GLI2 gene.
LHX3
LHX3 is a member of the LIM family of HomeoboX transcription factors. The LHX3 gene is located on 9q34.3, comprises 7 exons (including two alternative exon 1's, 1a and 1b) and encodes a protein of 402 amino acids (Figure 2. LHX3 is expressed in the developing Rathke's pouch and is required for the development of most anterior pituitary cell types, including the somatotrope, the thyrotrope, the lactotrope and the gonadotrope (but notably not the corticotrope). LHX3binds as a dimer, synergizing with POU1F1 (PIT1). Two unrelated families with MPHD were identified in 2000 (20) as harboring mutations in LHX3. The affected members of the family manifested severe growth retardation in association with restricted rotation of the cervical spine and a variable degree of sensory neural hearing loss. Inheritance is consistent with an autosomal recessive pattern of inheritance and of note one individual was found to have an enlarged pituitary. Recently, a new mutation in LHX3 was described in a child with hypointense pituitary lesion, focal amyotrophy and mental retardation in addition to neck rigidity and growth retardation (21). These clinical findings expand the phenotype associated with mutations in LHX3.
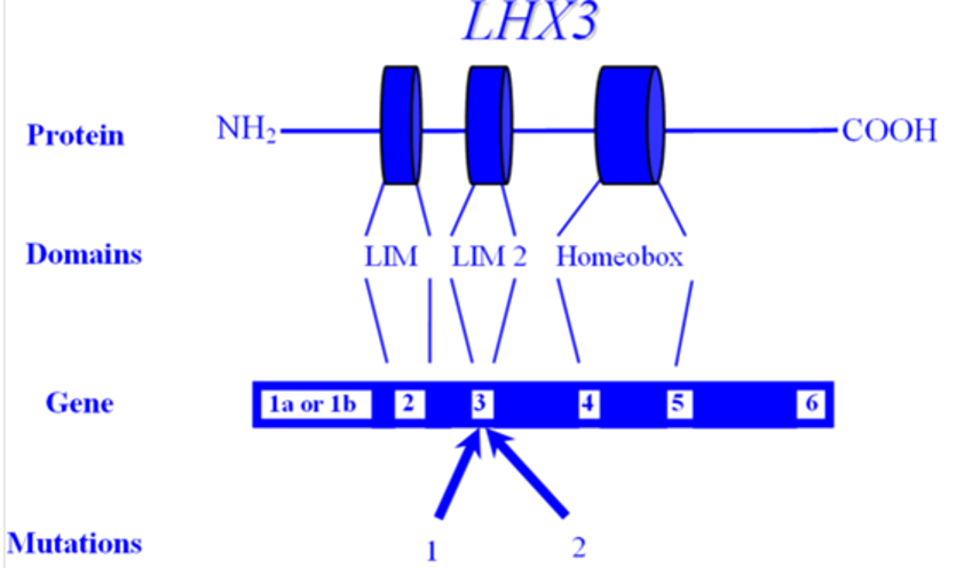
Figure 2. The LHX3 Gene.
LHX4
LHX4 also has a critical role in the development of anterior pituitary cells and is co-expressed with LHX3 in Rathke's pouch in an overlapping but not wholly redundant pattern. Raetzman et al showed overlapping functions with PROP1 in early pituitary development but also observed that their mechanisms of action were not identical (22). While LHX4 is necessary for cell survival, LHX3 expression is required for cell differentiation (23). The pituitary hypoplasia seen in LHX4 mutants results from increased cell death and reduced differentiation due to loss of LHX3 (22). However, PROP1mutants exhibit normal cell proliferation and cell survival but show evidence of defective dorsal-ventral patterning (22). In the absence of both LHX4 and LHX3 genes, no specification of corticotropes, gonadotropes or thyrotropes occurs in the anterior lobe. Although both LHX3 and LHX4 are crucial for the development of pituitary gland, LHX3 is expressed at all stages studied, whereas LHX4 expression is transient at 6 weeks of development (24). LHX4 is located on 1q25 and comprises 6 exons spread over a 45 kb genomic region (Figure 3). An intronic splice site mutation has been described in one family, manifesting GH, TSH and ACTH deficiency, along with cerebellar and skull defects. The mutation is transmitted as an autosomal dominant condition, with complete penetrance. Interestingly, a heterozygous mutant mouse model had no discernable phenotype, while homozygous loss of function in the mouse was fatal (25).
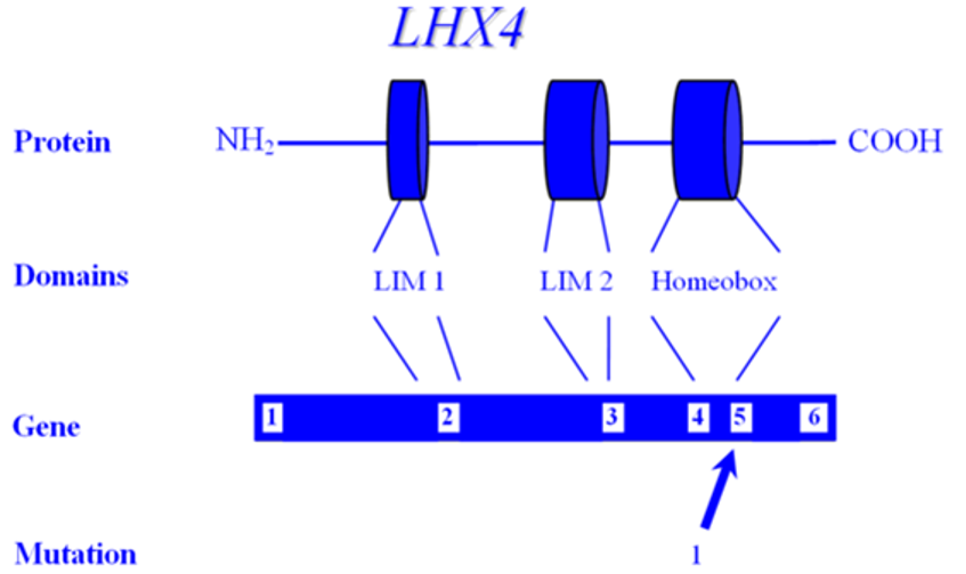
Figure 3. The LHX4 Gene.
HESX1
HESX1 (HomeoboX gene expressed in Embryonic Stem cells), also referred to as RPX1 (Rathke's Pouch HomeoboX) is necessary for the development of the anterior pituitary. RPX1 comprises 4 exons and encodes a protein of 185 amino acids that features both a homeodomain as well as a repressor domain and is located on chromosome 3p21.2 (Figure 4). The extinguishing of HESX1 requires the appearance of another pituitary transcription factor, PROP1. A mutation has been described in two children of a consanguineous union who had optic nerve hypoplasia, agenesis of the corpus callosum and panhypopituitarism, with an apparent autosomal recessive mode of inheritance (26). This Arg → Cys mutation lies between the repressor and homeodomains, but the mutant protein was shown in vitro to be unable to bind to its cognate sequences. A novel homozygous missense mutation (126T) of the critical engrailed homology repressor domain (eh1) of HESX1 was described in a girl born to consanguineous parents (27). Neuroimaging revealed a thin pituitary stalk with anterior pituitary hypoplasia and an ectopic posterior pituitary. Unlike previous cases, she did not have midline or optic nerve abnormalities. Although 126T mutation did not affect the DNA-binding ability of HESX1, it impaired ability of HESX1 to recruit Groucho-related corepressor, thereby leading to partial loss of repression. It appears that HESX1 mutations exhibit a variety of clinical phenotypes with no clear genotype-phenotype correlation
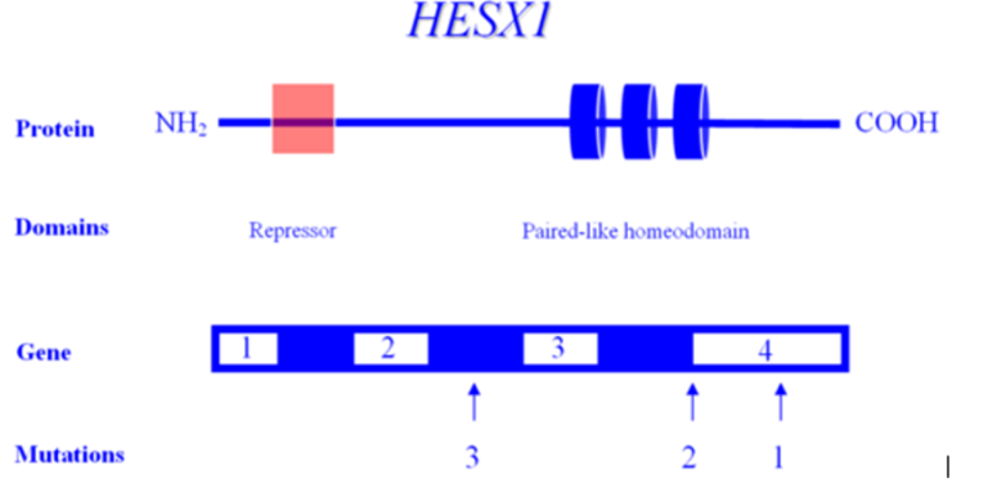
Figure 4. The HESX1 Gene.
PROP1
PROP1 (the Prophet of PIT-1) encodes a transcription factor required for the development of most pituitary cell lines, including the somatotrope (GH secretion), lactotrope (prolactin (PRL) secretion), thyrotrope (TSH secretion), and the gonadotrope (FSH and LH secretion). Mutation of PROP1, therefore, results in the deficiency of GH, TSH, PRL, FSH and LH although some individuals with PROP1 mutations have been described with ACTH deficiency (30). Since PROP1 does not appear to be required for the development of the corticotrope cell line, the etiology of ACTH deficiency is unclear. It appears that the ACTH deficiency here is a consequence of the compensatory pituitary hyperplasia that develops over time. Significantly, the degree of TSH deficiency appears to be quite variable, even within mutation-identical individuals, suggesting that the general phenotype associated with PROP1 mutations is also quite variable. PROP1 is encoded by three exons and is located on 5q. Many mutations have been described in PROP1-all inherited in an autosomal recessive manner. Although several studies suggest that mutation of PROP1 is the most common cause of familial MPHD, it is less common in sporadic cases of MPHD (14,31). Two recurrent mutations have been described, both involving exonic runs of GA tandem repeats (Figure 5). In both cases, the loss of a tandem unit at either locus results in a frameshift and premature termination, and a protein incapable of transactivation.
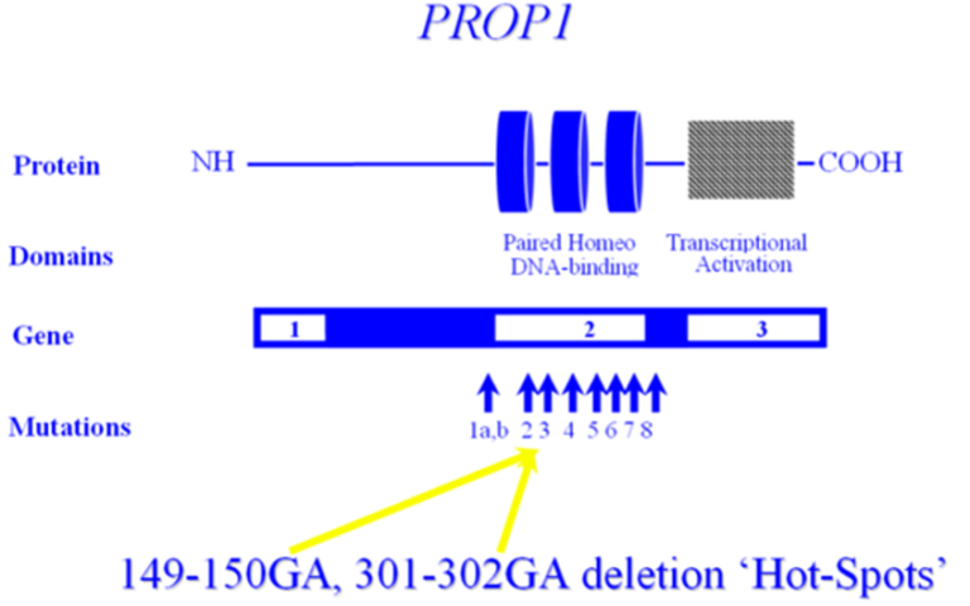
Figure 5. The PROP1 Gene.
POU1F1
POU1F1 encodes the POU1F1 transcription factor, also known as PIT1, which is required for the development and function of three major cell lines of anterior pituitary: somatotropes, lactotropes and thyrotropes. Various mutations in the gene encoding POU1F1 have been described, resulting in a syndrome of multiple pituitary hormone deficiency involving GH, PRL and TSH hormones. POU1F1 is located on 3p11 and consists of six exons encoding 291 amino acids (Figure 6). Many mutations of POU1F1 have been described; some are inherited as autosomal recessive and some as autosomal dominant. There is a wide variety of clinical presentations in patients with POUF1 mutations. Generally, GH and prolactin deficiencies are seen early in life. However, TSH deficiency can be highly variable with presentation later in childhood or normal T4 secretion can be preserved into the 3rd decade (32,33). POU1F1 mutations have been described in a total of 46 patients from 34 families originating in 17 different countries (34). Recessive mutations are generally associated with decreased activation, while dominant mutations have been shown to bind but not transactivate - i.e. act as dominant-negative mutations, rather than through haploinsufficiency. One such mutation is the recurrent Arg271Trp (R271W), located in exon 6, which results from a C T transition at a CpG dinucleotide, i.e. a region predisposed to spontaneous mutagenesis. Another interesting mutation is the Lys216Glu mutation of exon 5. This mutation is unique in that the mutant transcription factor activates both the GH and PRL promoters at levels greater than wild-type (i.e. acts as a super-agonist), but down-regulates its own (i.e. the POU1F1) promoter-leading to decreased expression of PIT1. R271W is the most frequent mutation of POU1F1. Another report described a novel mutational hot spot (E230K) in Maltese patients suggests a founder effect (33). The same group reported two additional novel mutations within POU1F1 gene; an insertion of a single base pair (ins778A) and a missense mutation (R172Q) (31).
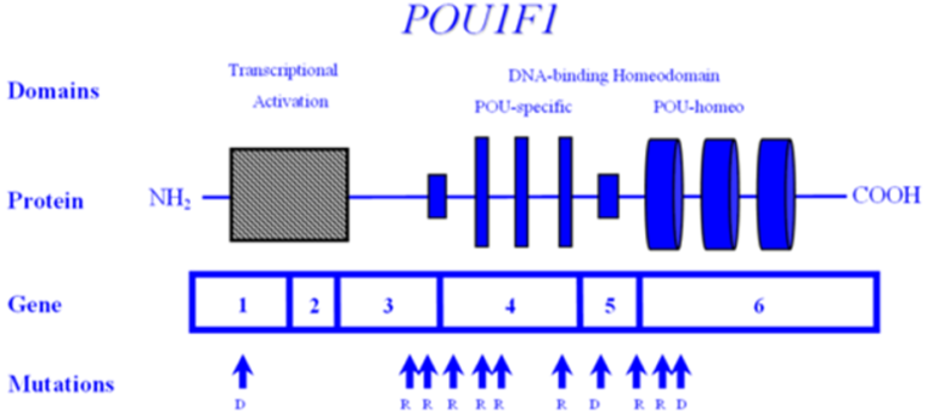
Figure 6. The POU1F1 gene.
SOX3
SOX3 encodes a single-exon gene SOX3, an HMG box protein, located on the X chromosome (Xq26.3) in all mammals(35). It is believed to be the gene from which SRY, testis–determining gene evolved (36). Based on sequence homology, SOX, however, is more closely related to SOX1 and SOX2, together comprising the SOXB1 subfamily and are expressed throughout the developing CNS (37,38). In humans, mutations in the SOX3 gene have been implicated in X-linked hypopituitarism and mental retardation. In a single family, a SOX3 gene mutation was shown in affected males who had mental retardation and short stature due to GH deficiency (39). The mutation was an in-frame duplication of 33 bp encoding for an additional 11-alanine, causing an expansion of a polyalanine tract within SOX3. Other mutations including a submicroscopic duplication of Xq27.1 containing SOX3, a novel 7-alanine expansion within the polyalanine tract, and a novel polymorphism (A43T) in the SOX3 gene were described in males with hypopituitarism (40). Phenotypes of these patients include severe short stature, anterior pituitary hypoplasia, ectopic posterior pituitary, hypoplastic corpus callosum, and infundibular hypoplasia. Although duplications of SOX3 have been implicated in the etiology of X-linked hypopituitarism with mental retardation, in at least one study, none of the affected individuals had mental retardation or learning difficulties (40). Taken together, the data suggests that SOX3 has a critical role in the development of the hypothalamic-pituitary axis in humans, and mutations in SOX3 gene are associated with X-linked hypopituitarism but not necessarily mental retardation (40).
ISOLATED GH DEFICIENCY (IGHD)
Abnormalities either in the synthesis or the activity of GH can cause a wide variation in the clinical phenotype of the patient. Most frequently, it occurs as a sporadic condition of unknown etiology but severe forms of IGHD may result from mutations or deletions in GH1 or GHRHR gene. General clinical features of IGHD deficiency include proportionate growth retardation accompanied by a decreased growth velocity, puppet-like facies, mid-facial hypoplasia, frontal bossing, thin hair, a high-pitched voice, microphallus, moderate trunk obesity, acromicria, delayed bone maturation and dentition. Most children with IGHD have normal birth weight and length, however, some newborns may present with hypoglycemia, microphallus, and prolonged jaundice. Patients with IGHD appear younger than their chronological age. Puberty may be delayed until late teens, but usually fertility is preserved.
To date, four Mendelian patterns of inheritance for IGHD have been identified based on the type of defect, mode of inheritance, and degree of deficiency.
- Type 1 GH deficiency is an autosomal recessively inherited condition, which exists as either complete, or partial loss of GH expression.
- a) Type 1a deficiency is characterized by the complete absence of measurable GH. Infants born with a type 1a defect are generally of normal length and weight, suggesting that, in utero, GH is not an essential growth factor (41,42). Growth immediately after birth and during infancy may also be less dependent on circulating GH levels than during other phases of life. Patients with Type 1a deficiency initially respond to rhGH treatment well. However, about 1/3 of patients develop antibodies to GH which leads to markedly decreased final height as adults (34). The exact prevalence of Type 1a deficiency is not known, and most reported families are consanguineous (34). Mutations in Type 1a have been described in GH1 and GHRHR-including nonsense mutations, microdeletions/frameshifts, and missense mutations.
- b) Type 1b deficiency represents a state of partial - rather than an absolute - deficiency of GH, with measurable (but insufficient) serum GH. Therefore, Type 1b is milder than Type 1a deficiency. Patients with Type 1b deficiency do not typically present with mid-facial hypoplasia or microphallus. They also have a good response to GH treatment without developing GH antibodies. Most cases of Type 1b GH deficiency are caused by missense and/or splice site mutations in the GH1 and GHRHR genes (43).
- Type 2 GH deficiency is an autosomal dominantly inherited disorder with reduced secretion of GH. Patients with Type 2 GHD usually do not have any pituitary abnormality (44). However, recently, it has been shown that their pituitary may become small over time (45). They have a good response to GH treatment. This type of GH deficiency is intuitively less clear, since autosomal dominant conditions generally occur because of either haploinsufficiency or secondary to dominant-negative activity. Haploinsufficiency, however, has not been demonstrated in the obligate heterozygote carriers of individuals harboring GH1 deletions, and is therefore an unlikely explanation. Dominant-negative activity is usually associated with multimeric proteins, also making this explanation less intuitive. Type 2 GHD appears to be the most common form of IGHD, and many mutations have been identified in GH1 including splicing and missense mutations (46–53). Recent studies suggest that GH1 may not be the only gene involved in Type 2 GHD. Screening 30 families with autosomal dominant IGHD did not show any GH1 mutations, raising the possibility of other gene(s) being involved (54).
- Type 3 growth hormone deficiency is inherited in an X-linked recessive manner. There are no candidate genes and no compelling explanations for this condition. There are no reported mutations of the GH-1 gene in Type 3 GHD. In addition to short stature, patients may also have agammaglobulinemia (34).
Table 2 summarizes the phenotypes of mutations involved in human pituitary transcription factors causing IGHD and MPHD and their mode of inheritance.
Table 2. Genotype and Phenotype Correlations in Human Pituitary Transcription Factors
|
Gene
|
Phenotype
|
Mode of Inheritance
|
IGHD
|
GH-1
|
IGHD type 1a/1b
IGHD type 2
IGHD type 3
|
AR
AD
X-linked
|
GHRHR
|
IGHD type 1b
|
AR
|
MPHD
|
LHX3
|
Deficiencies of GH, TSH, LH, FSH, PRL, rigid neck, small/normal/or enlarged anterior pituitary
|
AR
|
LHX4
|
Deficiencies of GH, TSH and ACTH, small anterior pituitary, cerebellar and skull defects
|
AD
|
HESX1
|
Hypopituitarism, optic nerve hypoplasia, agenesis of the corpus callosum, ectopic posterior pituitary
|
AR/AD
|
PROP1
|
Hypopituitarism except ACTH deficiency, small/normal/or enlarged anterior pituitary
|
AR
|
POU1F1 (PIT1)
|
Deficiencies of GH, TSH, PRL, small or normal anterior pituitary
|
AR/AD
|
SOX3
|
Hypopituitarism, mental retardation, learning difficulties, small anterior pituitary, ectopic posterior pituitary,
|
X-linked recessive
|
OTX2
|
Hypopituitarism, microphthalmia, microcephaly, cleft palate
|
AD
|
GLI2
|
Hypopituitarism, small anterior pituitary, ectopic posterior pituitary, holoprosencephaly, polydactyly
|
AD
|
AR: Autosomal Recessive; AD: Autosomal Dominant.
HYPOTHALAMIC GH DEFICIENCY
Synthesis and Secretion of GH
GH is synthesized within the somatotropes of the anterior pituitary gland and is secreted into circulation in a pulsatile fashion under tripartite control, stimulated by growth hormone releasing hormone (GHRH), Growth Hormone Secretagogues (GHS) such as Ghrelin and inhibited by somatostatin (SST) (Figure 7). GHRH, GHS and SST secretion are themselves regulated by numerous central nervous system neurotransmitters (Table 3). GH, via a complex signal transduction, exerts direct metabolic effects on target tissues and exerts many of its growth effects through releasing of IGF1 which is mainly produced by the liver and the target tissues (e.g. growth plates). Additional regulation of GH secretion is achieved through feedback control by IGF1 and GH at the pituitary and at the hypothalamus.
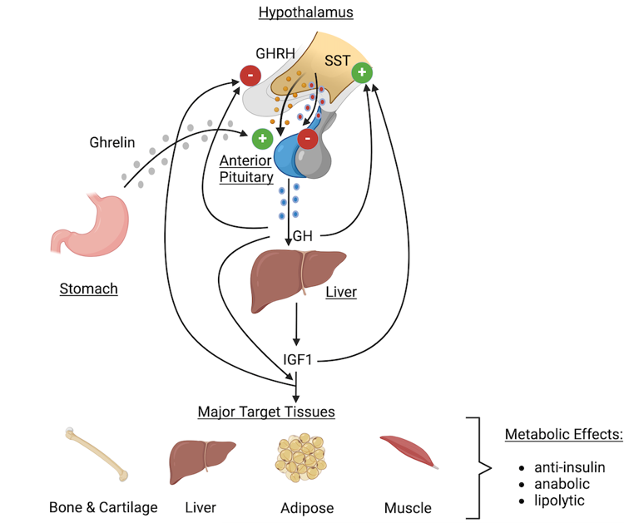
Figure 7. Hypothalamic-pituitary-peripheral regulation of GH Secretion. SST, somatostatin; GHRH, growth hormone releasing hormone; IGF1, insulin-like growth factor type 1.
Table 3. Neurotransmitters and Neuropeptides Regulating GHRH Secretion from Hypothalamus
|
Dopamine
|
Gastrin
|
GABA
|
Neurotensin
|
Substance-P
|
Calcitonin
|
TRH
|
Neuropeptide-Y
|
Acetylcholine
|
Vasopressin
|
VIP
|
CRHs
|
Timing
In addition to the absolute GH levels reached, the timing of the GH pulse is also physiologically important. GH is secreted in episodic pulses throughout the day, and the basal levels of GH are often immeasurably low between these peaks (Figure 8). Figure 8 illustrates normal spontaneous daily GH secretion, while figure 9 represents that of a child with GH deficiency.
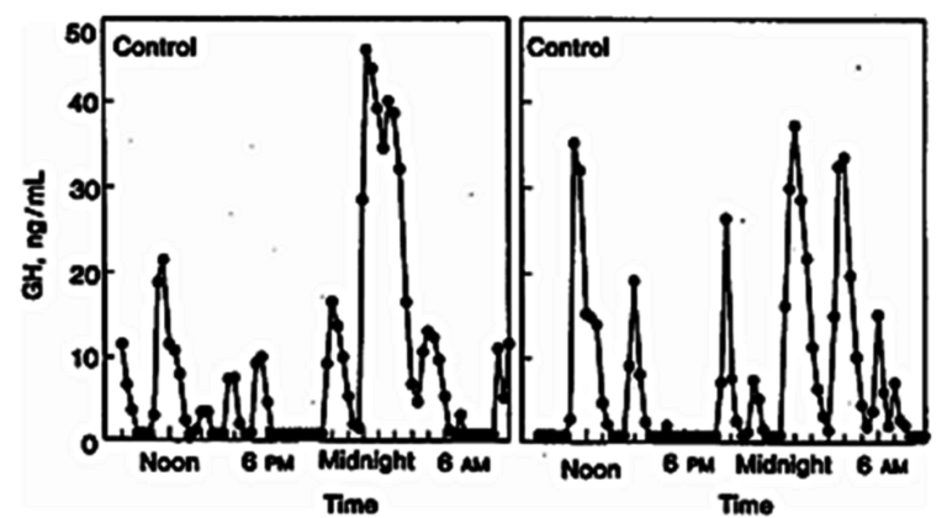
Figure 8. The characteristic pulsatile pattern of GH secretion in normal children. Note the maximal GH secretion during the night.
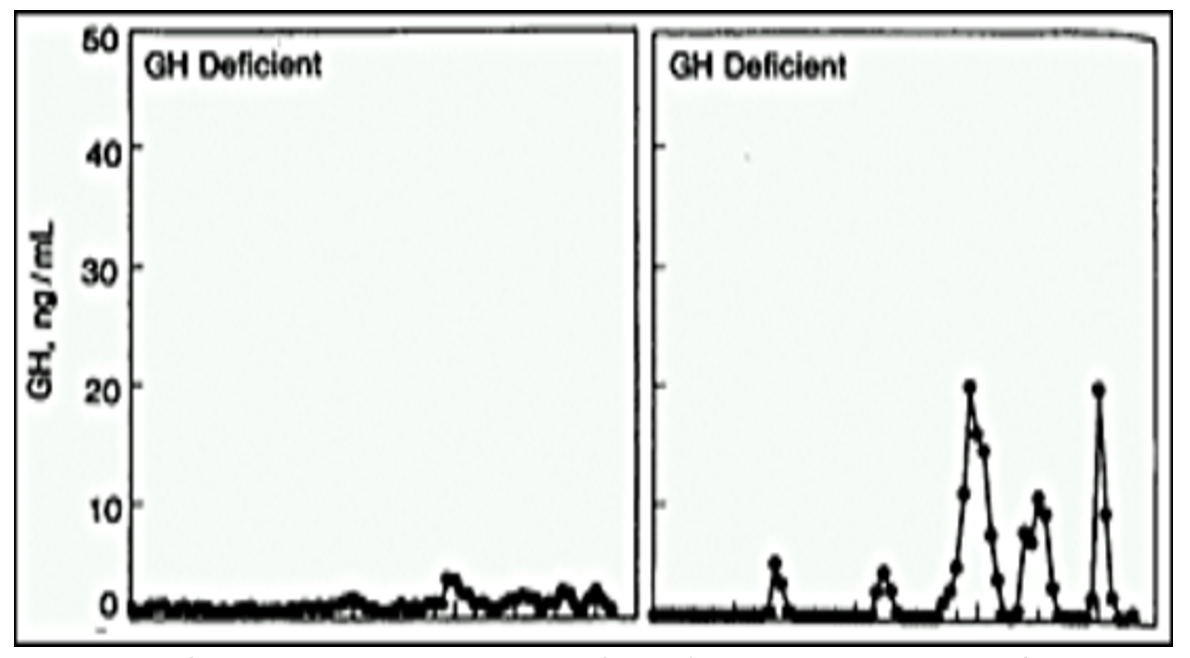
Figure 9. GH secretion in a child with GH deficiency. Note the loss (both qualitative and quantitative) of episodic pulses seen in normal children.
Approximately 67% or more of the daily production of GH in children and young adults occurs overnight, and most of that during the early nighttime hours that follow the onset of deep sleep. During puberty, there is an increase in GH pulse amplitude and duration, most likely due to estrogens (55). GH secretion is sexually dimorphic, with females having higher secretory burst mass per peak but no difference in the frequency of peaks, or basal GH release (56). In addition, GH secretion is stimulated by multiple physiological factors (Table 4). Overweight children, independent of pubertal status, have reduced GH levels mainly due to reduced GH burst mass with no change in frequency (57).
Table 4. Physiological Factors that Affect GH Secretion
|
Factors that stimulate GH secretion
|
Factors that suppress GH secretion
|
Exercise
|
Hypothyroidism
|
Stress
|
Obesity
|
Hypoglycemia
|
Hyperglycemia
|
Fasting
|
High carbohydrate meals
|
High protein meals
|
Excess glucocorticoids
|
Sleep
|
|
Growth Hormone Releasing Hormone
GHRH (also known as Somatocrinin) is the hypothalamic-releasing hormone isolated in 1982 (58) believed to be the chief mediator of GH secretion from the somatotrope. GHRH deficiency is thought to be the most common cause of 'acquired' GHRH deficiency, secondary to (even mild) birth trauma. GHRH includes 5 exons, with transcription of (the non-coding) exon 1 differing on a tissue-specific basis (59). The mature GHRH protein contains 44 amino acids, with an amidated carboxy terminus (Figure 10). Despite this post-translational modification, much of the GH-secreting ability resides in the (original) amino half, allowing the synthesis of shorter peptides retaining efficacy (e.g. 1-29 GHRH). Despite being cloned in 1985 (60), there are no reports of (spontaneous) mutations in humans or in any animal model. Individuals with mutations in GHRH are predicted to have isolated GH deficiency.
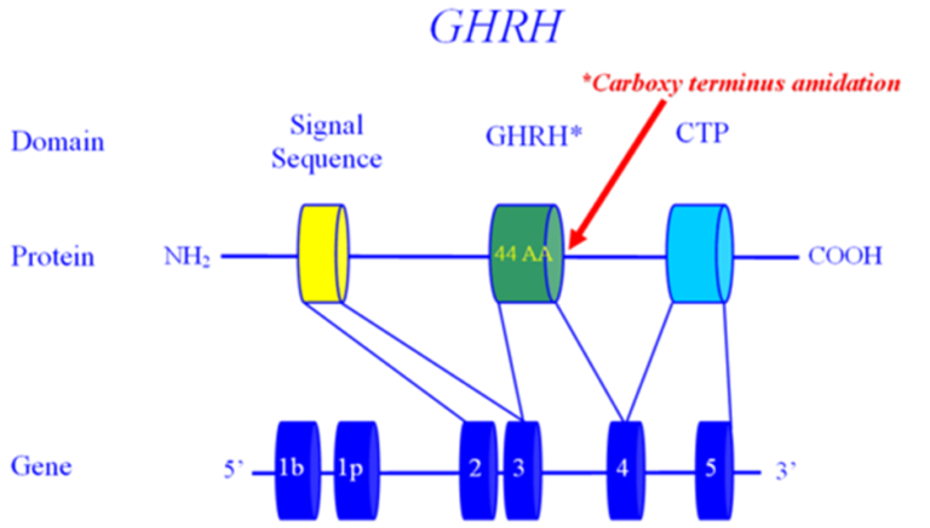
Figure 10. The growth hormone releasing hormone (GHRH) gene.
Growth Hormone Releasing Hormone Receptor
GHRHR was cloned in 1992 (61), described as the cause of isolated GH deficiency (IGHD) in the Little strain of dwarf mouse by 1993 (62,63), mapped in the human by 1994 (64), and demonstrated to be a cause of human GH deficiency in 1996 (43). GHRHR is located on 7p15 (64), comprises 13 exons and encodes a protein of 423 amino acids, belonging to the G-protein coupled, heptahelical transmembrane domain receptors (Figure 10). The initial reports of GHRHRmutations were in geographically isolated (and therefore endogamous) populations in South Asia (43,65,66) and later in Brazil (67). In fact, haplotype analysis of the GHRHR locus in three unrelated families from the Indian subcontinent, carrying the identical E72X nonsense mutation in GHRHR indicated that this represents a common ancient founder mutation (68). An independent analysis of patients with familial isolated GH deficiency from non-consanguineous families revealed that most patients carried the identical E72X mutation, suggesting that E72X mutation can be a reasonable candidate for isolated GH deficiency (69). There are now numerous other reports, making GHRHR one of the most mutated genes in IGHD. Roelfsema et al studied two members of a single family with an inactivating mutation of the GHRHR and noted that the 'normal' pattern of spontaneous GH production was preserved, although the absolute quantity of GH secreted was quite low and the approximate entropy significantly elevated (70); supporting the view that the amplitude of a GH pulse is the result of a GHRH burst, while the timing of GH pulses is the result of a somatostatin trough.
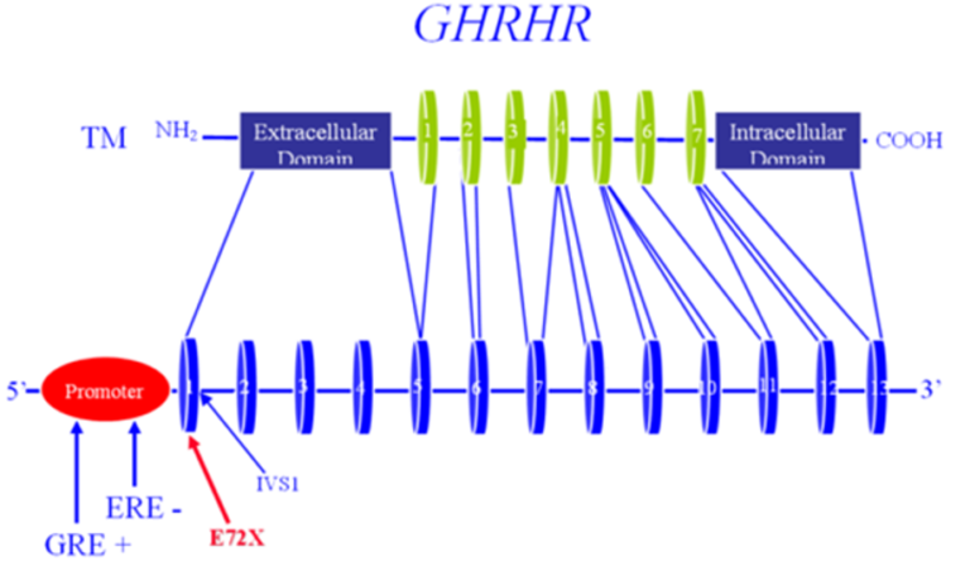
Figure 11. The growth hormone releasing hormone receptor (GHRHR) gene.
Ghrelin
In 1977 Bowers et al (71) reported on the ability of enkephalins to secrete GH and it was later demonstrated that this secretion was independent of GHRH. This sentinel finding gave rise to a new field of study, that of the growth hormone releasing peptides (GHRPs) or growth hormone secretagogues (GHSs). Twenty-two years later Kojima et al (72)reported the isolation of the endogenous ligand whose actions were mimicked by the enkephalins. They named the hormone Ghrelin, based on the Proto-Indian word for 'grow'. Ghrelin is located on 3p25-26 (73) (Figure 12), is processed from a ‘preproGhrelin’ precursor, and is primarily produced by the oxyntic cells of the stomach and to a lesser extent in the arcuate ventro-medial and infundibular nuclei of the hypothalamus (74). Ghrelin also plays a role in regulating food intake. In addition to its GH secreting actions, direct intracerebroventricular injection of Ghrelin in mice has potent orexigenic “appetite stimulating” action, and this action is mediated by NPY, which antagonizes the actions of Leptin.
Several studies have shown that, on a molar basis, Ghrelin is significantly more potent at inducing GH secretion than GHRH (75). Additionally, many of these studies have shown that Ghrelin and GHRH are synergistic, inducing a substantial GH response when given in combination (76–79). Several studies comparing GHRH and Ghrelin demonstrate that 1 ug/kg GHRH results in a GH peak of approximately 25 ng/ml, 1 ug/kg Ghrelin results in a GH peak of approximately 80 ng/ml GH, but when given together, 1ug/kg of GHRH + 1 ug/kg Ghrelin results in a GH peak of approximately 120 ng/ml (79,80). When short normal children were compared to children with neurosecretory GH deficiency, Ghrelin secretion was similar in both groups during daytime, but higher Ghrelin levels were detected during the night in short children with neurosecretory GH deficiency. The authors therefore suggest that Ghrelin is not involved in nighttime GH secretion (81), although these findings are also consistent with a relative Ghrelin insensitivity at night. In a group of boys with constitutional delay of puberty, testosterone administration caused the expected increase in GH concentrations but did not affect the 24-hour Ghrelin profile, suggesting that the testosterone-induced GH secretion was not mediated by Ghrelin (82). Another study demonstrated a decrease in Ghrelin concentrations following glucagon administration in a group of non-GH-deficient short children, suggesting that Ghrelin does not mediate glucagon-induced GH secretion (83).
A second hormone, Obestatin is also known to be produced from preproghrelin. Obestatin has anorexigenic effects, opposite those of Ghrelin (84). Several nucleotide changes have been identified in the preproghrelin locus, and some are associated with body mass index, BMI (85). It is not clear, however, whether these are polymorphisms, or distinct mutations. It is also not clear whether these nucleotide variants exert their effects solely via an altered Ghrelin, a corrupted Obestatin, or a combination of the two. A knockout mouse lacking the preproghrelin locus had no statural or weight phenotype, but this may well be the result of the simultaneous loss of both ghrelin and Obestatin. To this point, a transgenic mouse with abnormal ghrelin but normal Obestatin did indeed have poor weight gain, explained by either ghrelin deficiency, unopposed Obestatin, or both. There are no reports of (spontaneous) mutations in Ghrelin associated with short stature, either in humans or in any animal model, although polymorphisms have been associated with weight/metabolic syndrome. The theoretical phenotype of such an individual would presumably be that of isolated GH deficiency, most likely of post-natal onset and possibly with an abnormally low appetite.
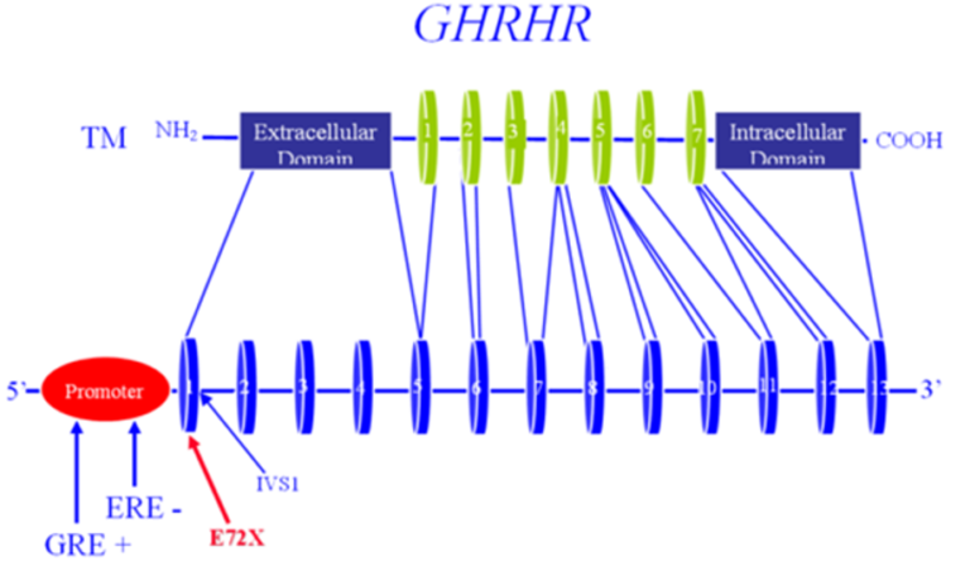
Figure 11. The growth hormone releasing hormone receptor (GHRHR) gene.
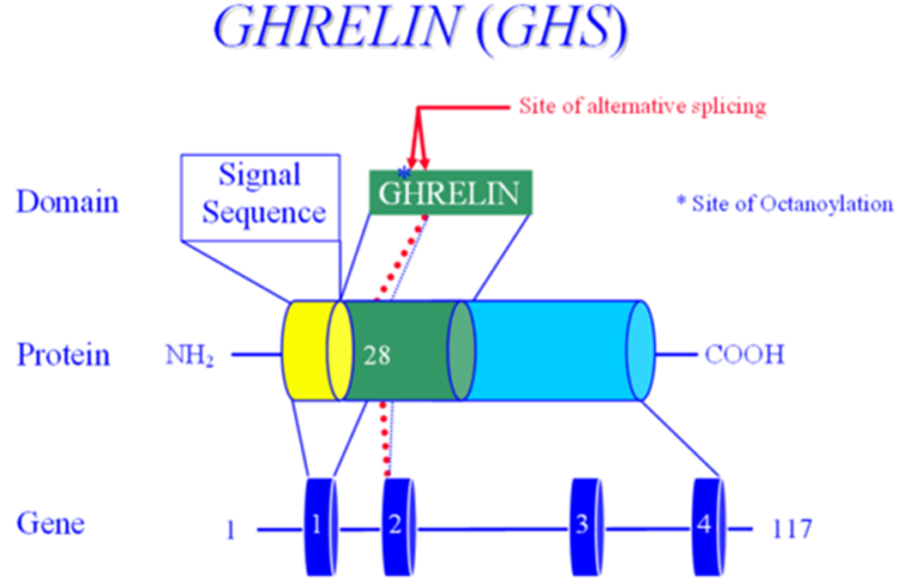
Figure 12. The ghrelin (GHS) gene structure.
Ghrelin Receptor
The receptor for Ghrelin (GHSR) was identified in 1996 by Howard et al (74), prior to the identification of the ligand, and maps to 3q26-27 (Figure 13). Mutations of the GHSR gene have been reported in individuals with isolated GH deficiency (86).
Combining data from numerous investigators, there appear to be differences in the specific roles of these parallel but independent pathways for GH secretion. Given that:
- Ghrelin induces a larger release of GH than GHRH,
- Both bolus and continuous GHRH infusion results in a chronic release of GH (87),
- A bolus of Ghrelin results in GH secretion, but continuous Ghrelin infusion does not; and
- Ghrelin administration (bolus or continuous) does not cause an increase in GH mRNA.
It is therefore likely that the GHRH/GHRHR arm of the somatotropin pathway serves primarily in the production of de novo GH, and secondarily in the release of (pre-made) GH while Ghrelin/GHSR may serve primarily in the release of stored GH, and only secondarily-if at all-in the production of de novo GH (80,88).
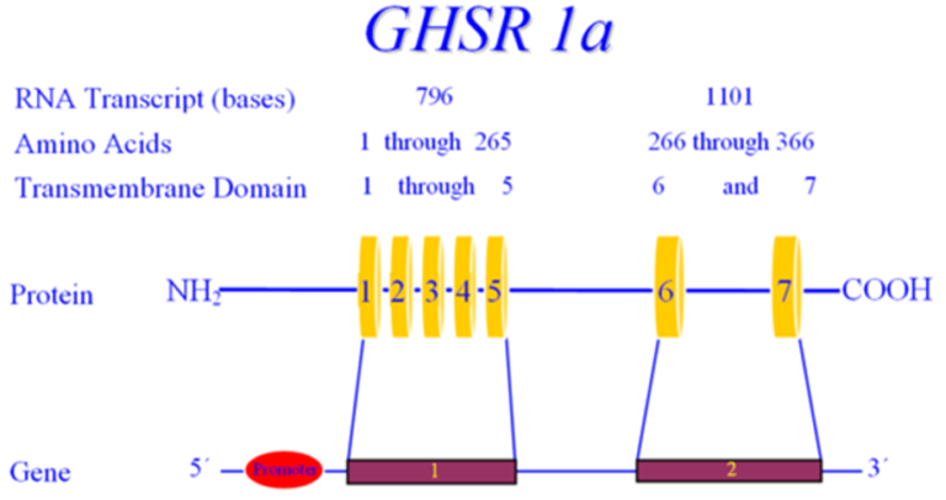
Figure 13. The Ghrelin receptor (GHSR) gene.
Somatostatin
The somatostatin gene (SST) is located on 3q28, and contains two exons, encoding a 116 amino acid pre-prosomatostatin molecule that is refined down to a 14 amino acid cyclic peptide (as well as a 28 amino acid precursor/isoform) (89) (see figure 14). Pancreatic somatostatin inhibits the release of both insulin and glucagon, while in the CNS, somatostatin inhibits the actions of several hypothalamic hormones, including GHRH. For this reason, somatostatin is also known as Growth Hormone Release Inhibiting Hormone. Somatostatin's widespread effects are mediated by five different receptors, all encoded by different genes (rather than through alternative splicing of a single gene). The anti-GHRH actions on the pituitary are primarily mediated by somatostatin receptors (SSTR) 2 and 5, which act by inhibiting cAMP as well as other pathways (90) (see figures 15 and 16). There is a single case report of a nucleotide variant in SSTR5, occurring in a subject with acromegaly. (This individual, however, was also reported as having a mutation in the GSP oncogene, placing the pathological nature of the SSTR5 variant in question). Whereas GHRH induces release of growth hormone stored in secretory vesicles by depolarization of the somatotrope, somatostatin inhibits GH release by hyperpolarizing the somatotrope, rendering it unresponsive to GHRH. There are no reports of mutations in the somatostatin gene, or in SSTR2.
All three of these hypothalamic modifiers of GH secretion act through cell-membrane receptors of the G-protein coupled receptor (GCPR) class. These receptors are characterized by seven membrane-spanning helical domains, an extracellular region that binds (but does not internalize) the ligand hormone, and an intracellular domain that interacts with a G-protein, which contains a catalytic subunit that generates a second messenger (e.g. cyclic AMP or inositol triphosphate).
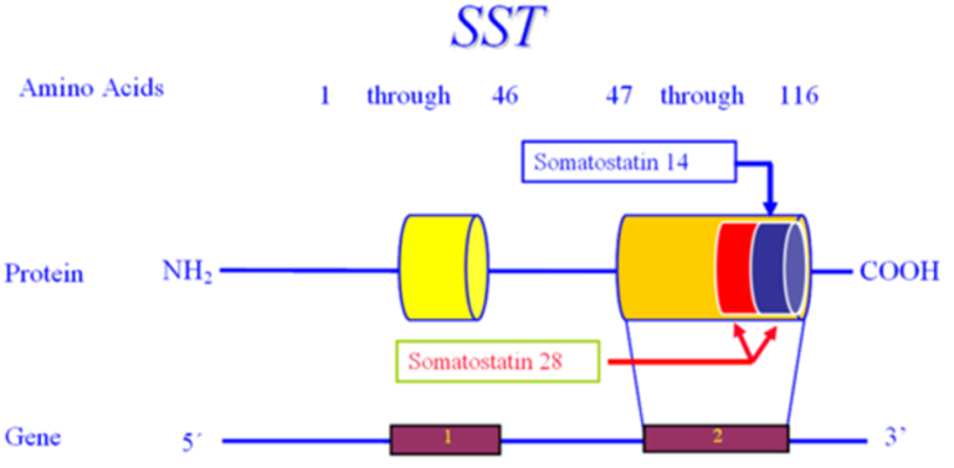
Figure 14. The somatostatin (SST) gene.
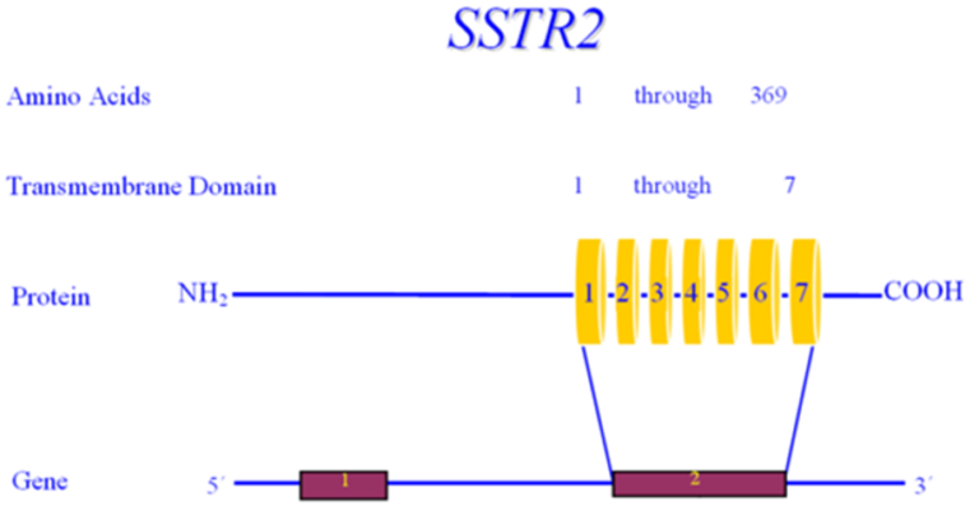
Figure 15. The somatostatin receptor 2 (SSTR2) gene.
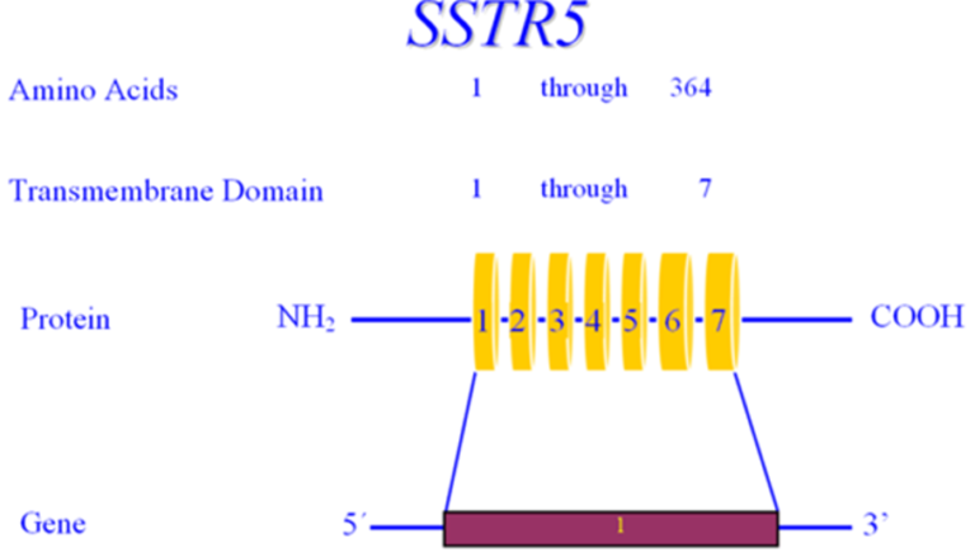
Figure 16. The somatostatin receptor 5 (SSTR5) gene.
PITUITARY GH DEFICIENCY
Human Growth Hormone
GH is critical for growth through (most of) childhood as well as for optimal metabolic, neurocognitive, cardiac, musculoskeletal and adipose function throughout life. GH acts through GH receptors on cells of a variety of target tissues. Many, but not all, actions of GH are mediated by insulin-like growth factor 1 (IGF1), also known as Somatomedin-C. IGF1 is released in response to GH and acts as both a hormone and an autocrine/paracrine factor. GH, directly and indirectly through the actions of IGF1, stimulates tissue growth and proliferation, most notably in the epiphyseal growth plates of children, increases lean muscle mass, decreases fat mass, and increases bone mineral density.
Growth hormone is a single-chain polypeptide that contains 191 amino acids with two intramolecular disulfide bonds and the molecular weight of 22,128 Daltons. The GH protein (GHN) is encoded by the GH1 gene located on chromosome 17q22-q24 (Figure 17) in a complex of five genes: two for the growth hormone/growth hormone variant (GH1, GH2), two for chorionic somatomammotropin (CS1, CS2), and one for the somatomammotropin pseudogene (CSL). GH2 encodes the GHV protein that is secreted by the placenta into maternal circulation. GHV has greater lactogenic properties than does GHN and may function to maintain the maternal blood sugar in a desirable range, thus ensuring sufficient nutrition for the fetus.
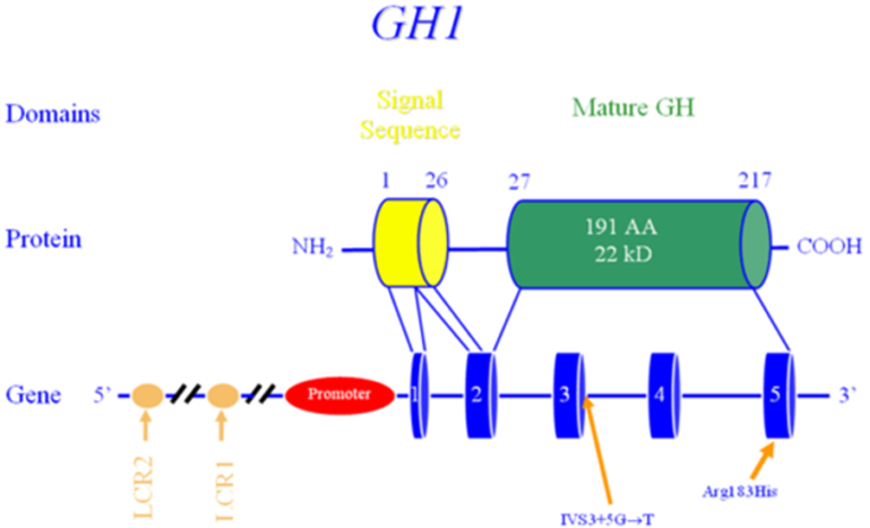
Figure 17. Growth hormone (GH1) gene.
PERIPHERAL GH RESISTANCE
Growth Hormone Receptor
Growth failure with normal serum GH levels is well known, both at the genetic and clinical level. Although such cases may be due to defects of GH1 (e.g. bio inactive GH), many such subjects have been shown to have mutations in the GH Receptor (GHR), i.e. Growth Hormone Insensitivity, known as Laron Syndrome. Biochemical hallmarks of this syndrome are increased or normal GH levels with low IGF1 and with absent or decreased response to GH treatment (91).
The growth hormone receptor gene (GHR) is located on 5p13-12 and contains 10 exons which span a physical distance of almost 300 kb of genomic DNA (Figure 18). The GHR consists of a ligand-binding extracellular domain, an 'anchoring' transmembrane domain and an intracellular domain with intrinsic tyrosine kinase activity. A monomeric GHR binds a single GH molecule, which then dimerizes a second GHR, and activates the JAK/STAT and MAPK pathways and is internalized. The internalization leads to extinguishing of the GH signal, and the GHR is recycled for further rounds of activity. Two naturally occurring isoforms of the GHR arise from alternative splicing-one with an alternate exon 3, and the other with an alternate exon 9. The alternative exon 9 isoform yields a protein with only amino acids 1-279, and virtually none of the intracellular domain. This isoform cannot transduce the GH signal and yields higher molar quantities of GHBP (than wild-type GHR) and therefore acts as a GH "sink" (92). The GHR isoform lacking exon 3 has a high prevalence, and may be associated with altered GH signaling, although the direction of the alteration is not clear (93–96).
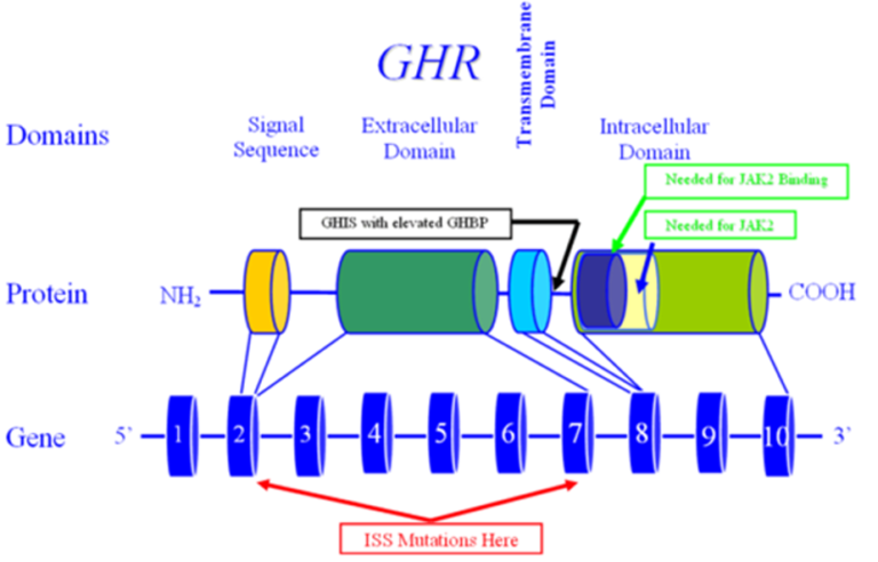
Figure 18. The growth hormone receptor (GHR) gene.
Defects in the GH signaling pathway have been demonstrated to be associated with postnatal growth failure. Mutations of Stat5b were reported in patients with severe growth failure. Several mutations of Stat5b gene have been reported. Although patients had a phenotype similar to that of congenital GH deficiency or GHR dysfunction, clinical and biochemical features (including normal serum GHBP concentrations) and immune deficiency (97) distinguish patients with STAT5b defects from patients with GHR defects. It also appears that STAT5b mutations are associated with hyperprolactinemia. It remains unclear whether the hyperprolactinemia is a direct or indirect effect of STAT5b mutations (98).
In humans, the extracellular portion of GHR is enzymatically cleaved and functions as the GH-Binding Protein (GHBP)(99). GHBP presumably serves to maintain GH in an inactive form in the circulation and to prolong the half-life of GH. Serum levels of GHBP are therefore used as a surrogate marker for the presence of GHR, and abnormal levels-both elevated and decreased-may indicate abnormality in the GHR (100,101). Of note is that mutations have also been described in individuals with 'normal' GHBP levels. GHI secondary to GHR mutations are mostly autosomal recessive mutations, but dominant negative mutations have also been described. Individuals with heterozygote mutations in GHRmay present with significant short stature (102). Mutations in GHR have also been associated with idiopathic short stature (ISS) (103–105). The original reports of GHR mutation described limited elbow extension and blue sclera, but these findings are not universal.
Many genetic abnormalities have been described in GHR, including nonsense mutations, missense mutations, macrodeletions, microdeletions and splice site changes. Of the latter, one of the most interesting is the "E180E" mutation, wherein an exonic adenosine is converted to a guanine, converting GAA to GAG, which would be predicted to not change the amino acid structure of GHR (both GAA and GAG encode glutamic acid). On this basis, this "silent polymorphism" would be expected to have no phenotype, but in reality, causes GH resistance and extreme short stature by activation of a cryptic splice site. This mutation was noted in Loja and El Oro, Ecuador in two large cohorts. This identical mutation has also been identified in Jews of Moroccan descent, suggesting that this mutation dates to at least the 1400’s and that the Ecuadorian cohorts, therefore, likely represent Sephardic Jews who left Spain around the time of the Inquisition at the end of the fifteenth century, CE (106). Another splice site mutation at position 785-3 (C>A in the intron 7) was recently described in a patient and mother with short stature and extremely elevated GHBP (107). The consequence of this novel mutation is a truncated GHR which lacks the transmembrane domain (encoded by exon 8) and the cytoplasmic domain. It was hypothesized by the authors that this GHR variant cannot attach to the cell membrane, and the continual secretion into the circulation results in the elevated levels of serum GHBP detected in the patient and his mother. The presence of the wild-type GHR allele presumably permits some level of normal GH-induced action.
Insulin-Like Growth Factor 1 (IGF1)
Many of growth hormone's physiological actions are mediated through the insulin-like growth factor, IGF1 (formerly referred to as somatomedin C). Serum IGF1 levels are commonly measured as a surrogate marker of GH status since IGF1 displays minimal circadian fluctuation in serum concentration. IGF1 plays a critical role in both prenatal and postnatal growth, signaling through the IGF1 as well as the insulin receptors (Table 5). IGF1 circulates as a ternary complex consisting of IGF1, IGFBP3, and ALS. IGF bioavailability is regulated by a metalloprotease termed ‘pregnancy-associated plasma protein-A2’ (PAPPA2) (108). PAPPA2 cleaves IGFBP3 or IGFBP5 to free IGF1 from its ternary complex, allowing it to bind to its target tissues. In addition to PAPPA2, stanniocalcin-2 (STC2) also plays a critical role in IGF1 bioavailability by inhibiting the proteolytic activity of PAPPA2 (109,110).
The IGF1 gene is located on 12q22-24.1, consists of six exons and spans over 45 kb of genomic DNA (Figure 19). Alternative splicing produces two distinct IGF1 transcripts, IGF1-A and IGF1-B. Woods et al described a male of a consanguineous union with prenatal (intrauterine) and postnatal growth retardation, sensorineural deafness and mental retardation (111). DNA analysis showed a homozygous partial deletion of the IGF1 gene (111,112). Subsequently, additional cases have been described (113,114).
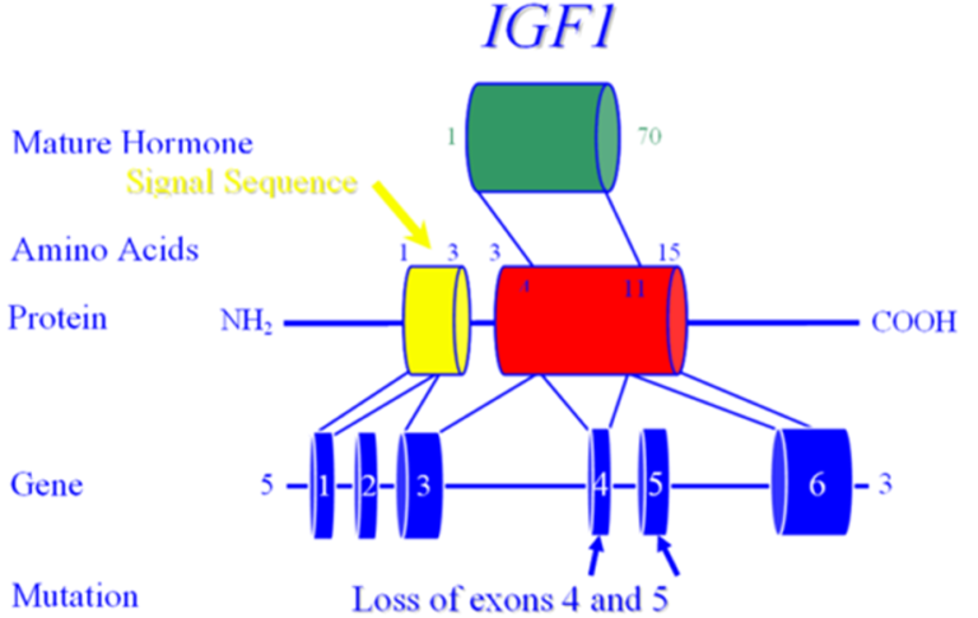
Figure 19. The IGF1 gene.
Mice engineered to completely lack Igf1 (Igf1 knockouts) are born 40% smaller than their normal littermates (115,116). Recent studies of a hepatic-only Igf1 knockout (KO) mouse, however, demonstrate that IGF1 functions primarily in a paracrine or autocrine role, rather than in an endocrine role (117). Liver specific Igf1 knock-out mice, were found to have a 75% reduction in serum Igf1 levels but were able to grow and develop (nearly) normally (117,118) with a mild phenotype developing only late in life (117). A further decrease in serum IGF1 levels of 85% was observed when double gene KO mice were generated lacking both the acid labile subunit (ALS) and hepatic IGF1. Unlike the single hepatic-only IGF1-KO's, these mice showed significant reduction in linear growth as well as 10% decrease in bone mineral density (119). Thus, as illustrated by the combination liver specific IGF1+ALS knock-out mouse model, there likely exists a threshold concentration of circulating IGF1 that is necessary for normal bone growth and suggests that IGF1, IGFBP3, and ALS may play an important role in bone physiology and the pathophysiology of osteoporosis.
In humans, homozygous mutations in ALS result in mild postnatal growth retardation, insulin resistance, pubertal delay, unresponsiveness to GH stimulation tests, elevated basal GH levels, low IGF1 and IGFBP3 levels and undetectable ALS (120–122). Although it is not clear why postnatal growth is mildly affected, it may be due to increased GH secretion due to loss of negative feedback regulation by the low circulating IGF1. Increased GH secretion could then up-regulate the functional GH receptor increasing local IGF1 production, thus partially protecting linear growth (97) (Figure 20). Over a dozen inactivating mutations of the IGFALS gene have been described in patients with ALS deficiency (123).
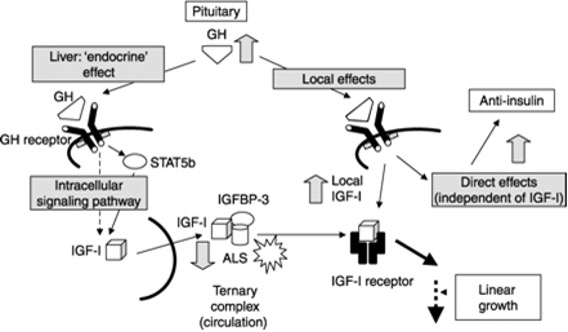
Figure 20. The effect of ALS mutations on the GH-IGF1 axis. Savage MO Camacho-Hubner C, David A, et al. 2007” Idiopathic short stature: will genetics influence the choice between GH and IGF1 therapy?” Eu J of Endocrine 157:S33 Society of European Journal of Endocrinology (2007). Reproduced by permission. Reprinted with permission (124).
Elevated IGF1 levels has recently been associated with colon, prostate and breast cancer (125–127) and the association was strongest when an elevated IGF1 was combined with a decreased IGFBP3 level. This combination-expected to yield more bioactive IGF1 may merely reflect the tumorigenic process, rather than demonstrate causality. Importantly, GH treatment induces a rise in both IGF1 as well as IGFBP3 (128) and therefore would not be expected to increase cancer risk in normal individuals.
Table 5. Summary of IGF1 Function in Different Systems and its Effects (129)
|
IGF1 Function
|
IGF1 Deficiency
|
Intrauterine Growth
|
IUGR
|
Postnatal Growth
|
Short Stature
|
CNS
|
Neurodegenerative disease
|
Insulin sensitization/improvement of glucose disposal/beta cell proliferation
|
Type 1 and Type 2 Diabetes
|
|
IGF1 Excess
|
Mitosis/Inhibition of apoptosis
|
Malignancy
|
IGF1 Deficiency
IGF1 deficiency can be classified based on decreased IGF1 synthesis (primary) or decreased IGF1 as a result of decreased or inactive GH (secondary) (130) (see Table 6).
Table 6. IGF1 Deficiency
|
I. Primary IGF1 Deficiency (normal or elevated GH levels)
II.
1. Defects in IGF1 Production:
1. Mutation in IGF1 gene or bio inactive IGF1
2. GHR receptor signaling defects (JAK/STAT)
3. Mutations in ALS gene
4. Factors affecting IGF1 production (malnutrition, liver, inflammatory bowel
disorders, celiac disease)
Defects in IGF1 Action:
1. IGF1 resistance due to receptor or post-receptor defects
2. Factors inhibiting IGF1 binding to IGF1R (increased IGFBPs and presence of IGF1 antibodies)
3.
Defects in GH Action:
1. Factors inhibiting (increased GHBPs and presence of GH antibodies)
2. GH receptor defects (decreased GH receptors, GHR antibodies, GHR gene defects)
|
III. Secondary IGF1 Deficiency (decreased GH levels)
Decreased GH production
1. Defects in GH gene
2. Defects in GHRH or GHRH receptor
3. Psychological disorders
Defects in hypothalamus and pituitary
|
Recombinant Human IGF1 (rhIGF1)
rhIGF1 is useful in the treatment of primary IGF1 deficiency resulting from abnormalities of the GH molecule (resulting in a bio inactive GH), the GH receptor (known as Laron syndrome) or GH signaling cascade (131). Studies have shown that rhIGF1 significantly improves height in children unresponsive to rhGH (132,133), and clinical trials clearly demonstrated better response to IGF1 therapy when initiated at an early age (134).
FDA approved conditions for rhIGF1 treatment for children with (135):
- Severe primary IGF1 deficiency
- GH gene deletions who have developed neutralizing antibodies to GH
Severe primary IGF1 deficiency is defined by:
- Height SD score is less than -3SD
- Basal IGF1 level is below -3SD
- Normal or elevated GH
The recommended starting dose of rhIGF1 is 40-80 microgram/kg twice daily by subcutaneous injection. If it is tolerated well for at least one week, the dose may be increased by 40 microgram/kg per dose, to the maximum dose of 120 microgram/kg per dose (136).
The most common side effects of IGF1 treatment are pain at injection site and headaches which mostly diminish after the first month of treatment (131). Other less common side effects are lipohypertrophy at the injection site, pseudotumor cerebri, facial nerve palsy and hypoglycemia (134). Another effect of IGF1 treatment is a significant increase in fat mass and BMI (137) — in contradistinction to the lipolytic effect of rhGH treatment. Coarsening of facial features, increased hair growth, slipped capital femoral epiphysis, scoliosis, hypersensitivity, and allergic reactions including anaphylaxis are other prominent adverse effects and are most commonly seen during puberty. Growth of lymphoid tissue is a concern which may require tonsillectomy (131).
Insulin-Like Growth Factor 1 Receptor (IGF1R)
The receptor for IGF1 is structurally related to the insulin receptor and similarly has tyrosine kinase activity (Figure 21). IGF1R is located on 15q25-26. The mature (human) IGF1 receptor contains 1337 amino acids and has potent anti-apoptotic activity (137). The IGF1 receptor transduces signals from IGF1, IGF2 and insulin. However, murine data suggest that initially (in the embryo) only the IGF2 signal is operational, while later on in development (i.e. the fetus), both IGF1 and IGF2 (and probably insulin) signal through the IGF1R (112). Hemizygosity for IGF1R has been reported in a single patient (and appears likely in others) with IUGR, microcephaly, micrognathia, renal anomalies, lung hypoplasia and delayed growth and development (138). Murine and human studies have shown that mutations in IGF1R result in combined intrauterine and postnatal growth failure (104), confirming the critical role of the IGF system on embryonic, fetal and postnatal growth. A novel heterozygous mutation in the tyrosine kinase domain of the IGF1R gene was recently identified in a family with short stature. The mutation, a heterozygous 19-nucleotide duplication within exon 18 of the IGF1R gene, results in haploinsufficiency of the IGF1R protein due to nonsense mediated mRNA decay (139).
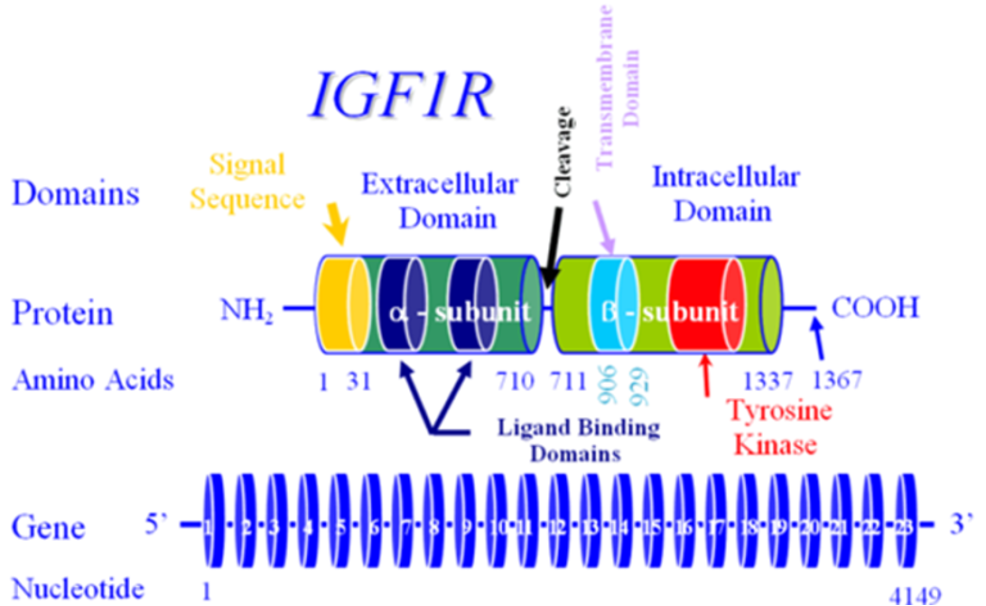
Figure 21. The Insulin-Like Growth Factor 1 Receptor (IGF1R) gene.
In summary, IGF1 and IGF1R mutations should be considered if a child presents with the following:
- Intrauterine and postnatal growth retardation
- Microcephaly
- Mental retardation
- Developmental delay
- Sensorineural deafness
- Micrognathia
- Very low or very high levels of serum IGF1
High serum (total) IGF1 levels are observed in patients with loss-of-function mutations in pregnancy-associated plasma protein A2 (PAPPA2) (140). As noted earlier, PAPPA2 is responsible for cleaving IGFBP3 or IGFBP5 to free bioactive IGF1 from its ternary complex so that IGF1 binds to its target sites (108). Loss-of-function mutations in PAPPA2 decrease biologically active (i.e. ‘free’) IGF1 levels and cause short stature (140,141). To date, a total of seven patients with PAPPA2 mutations have been reported from three unrelated families (141,142). Patients generally had similar clinical phenotypes with growth failure, microcephaly, micrognathia, delayed dental eruption, thin long bones, and decreased bone mineral density (141). Their total serum IGF1, IGF2, IGFBP3, IGFBP5, and ALS levels were elevated but the affected individuals had decreased free IGFI levels and IGF1 bioactivity (141). Serum PAPPA2 levels were either undetectable or low (143).
Both short-term and long-term treatment with rhIGF1 has been shown to be effective improving linear growth in children with PAPPA2 deficiency with variable height gain results and positive effects on bone mineral density and bone structure (142,144–148). Although the majority of subjects tolerated rhIGF1 treatment with no adverse effects, pseudotumor cerebri was noted to be the most common adverse event (147).
A single patient with PAPPA2 deficiency was administered fresh frozen plasma with the goal to restore PAPPA2 activity. Interestingly, the patient responded with significantly increased free IGF1 levels, but her serum PAPPA2 levels did not measurably increase.in vitro addition of rhPAPPA2 to the patients serum similarly demonstrated an increase in free IGF1, as did the serum of patients with ISS, although the increase in ISS patients was less robust (149). However, authors caution first establishing the normal ranges for PAPPA2 and free IGF1 before developing PAPPA2 as a potential novel treatment for growth.
Insulin-Like Growth Factor 2 (IGF2)
IGF2 is thought to be a major prenatal growth hormone and to be less critical for statural growth in post-natal life.
The human gene, IGF2, is located on 11p15.5 (Figure 22). Chromosome 11p15.5 carries a group of maternally (IGF2) and paternally (H19) imprinted genes that are crucial for embryonic and/or fetal growth. Genetic or epigenetic changes in the 11p15.5 region alter this growth (150). IGF2 is maternally imprinted, meaning that the maternal allele is unexpressed. The close proximity of INS to IGF2-in addition to nearly 50% amino acid identity-suggest that these genes arose through gene duplication events from a common ancestor gene. IGF2 acts via the IGF1 receptor (as well as the insulin receptor). Over-expression of IGF2 results in overgrowth, similar to that seen in Beckwith-Wiedemann Syndrome (which can be due to loss of imprinting, effectively doubling IGF2 expression). A mouse model overexpressing Igf2 demonstrates increased body size, organomegaly, omphalocele, cardiac, adrenal and skeletal abnormalities, suggestive of Beckwith-Wiedemann and Simpson-Golabi-Behmel syndromes (151). Interestingly, IGF2 expression is normally extinguished by the Wilm's Tumor protein (WT1), providing an explanation for the overgrowth (e.g. hemi-hypertrophy) typically seen in subjects with Wilm's Tumor (152). In contrast, mice without a functional Igf2 (Igf2 knockouts) are born 40% smaller than their normal littermates (identical to Igf1 knockouts).
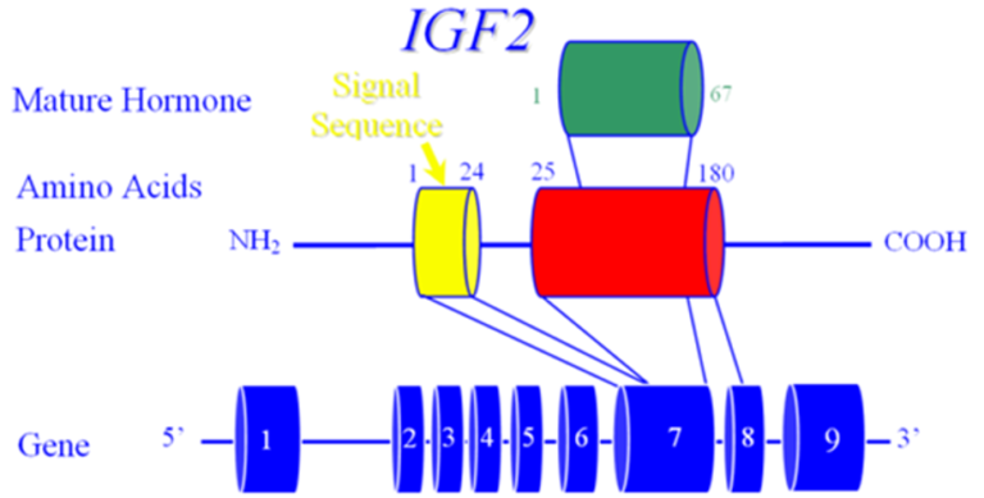
Figure 22.The Insulin-Like Growth Factor 2 (IGF2) gene.
Recent reports on individuals with severe intrauterine growth retardation showed maternal duplication of 11p15 (153). Furthermore, individuals with Silver-Russell-syndrome (SRS, also known as Russell-Silver syndrome) have been found to have an epimutation (demethylation) associated with biallelic expression of H19 and down regulation of IGF2(154,155). Russell-Silver syndrome is a congenital disorder characterized by intrauterine and postnatal growth retardation, typical facial features (triangular face, micrognathia, frontal bossing, downward slanting of corners of the mouth), asymmetry, and clinodactyly. Other chromosomal abnormalities such as maternal uniparental disomy on chromosome 7 also have been shown in 10% of individuals with SRS (156).
A paternally derived balanced chromosomal translocation that disrupted the regulatory regions of the predominantly paternally expressed IGF2 gene was described in a woman with short stature, history of severe intrauterine growth retardation (-5.4 SDS), atypical diabetes and lactation failure (157).
Insulin-Like Growth Factor 2 Receptor (IGF2R)
A receptor for IGF2, the IGF2R, has been identified, but does not appear to be the mediator of IGF2's growth promoting action. IGF2R is located on 6q26 and encodes a receptor unrelated to the IGF1 or insulin receptor (Figure 23). IGF2R is also the mannose-6-phosphate receptor and serves as a negative modulator of growth (for all IGF's and insulin). Its main role in vivo is probably as a tumor suppressor gene. While IGF2 is maternally imprinted, mouse Igf2R is paternally imprinted. There is some evidence that (in a temporally limited fashion) IGF2R is also paternally imprinted in humans. Somatic mutations have been found in hepatocellular carcinoma tissue (heterozygous mutations associated with loss of the other allele), but no germ-line mutations have been identified in individuals with growth abnormalities.
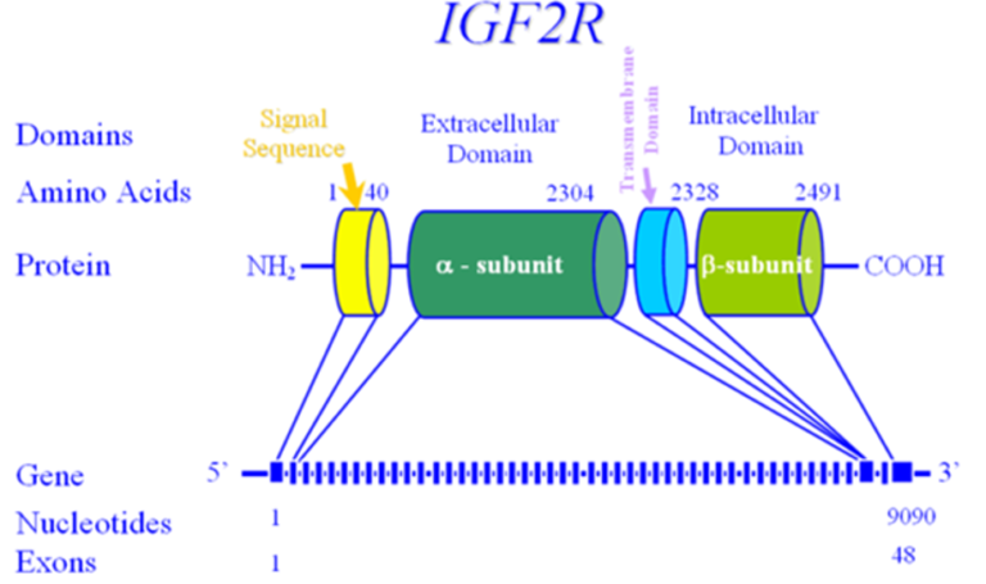
Figure 23. The Insulin-Like Growth Factor 2 Receptor (IGF2R) gene.
Insulin
In addition to its glycemic and metabolic roles, insulin functions as a significant growth promoting/anabolic agent. The insulin gene (INS) is located on Chr 11p15.5 and comprises 3 exons (Figure 24). Insulin's role in fetal growth is quite significant, as demonstrated by hyper insulinemic babies (e.g. infants of diabetic mothers (IDM)). Insulin's growth promoting activity is mediated through a combination of insulin and the IGF1 receptors. Mutations in the INS gene have been described in subjects with hyperinsulinemia (and/or hyperproinsulinemia) and diabetes mellitus.
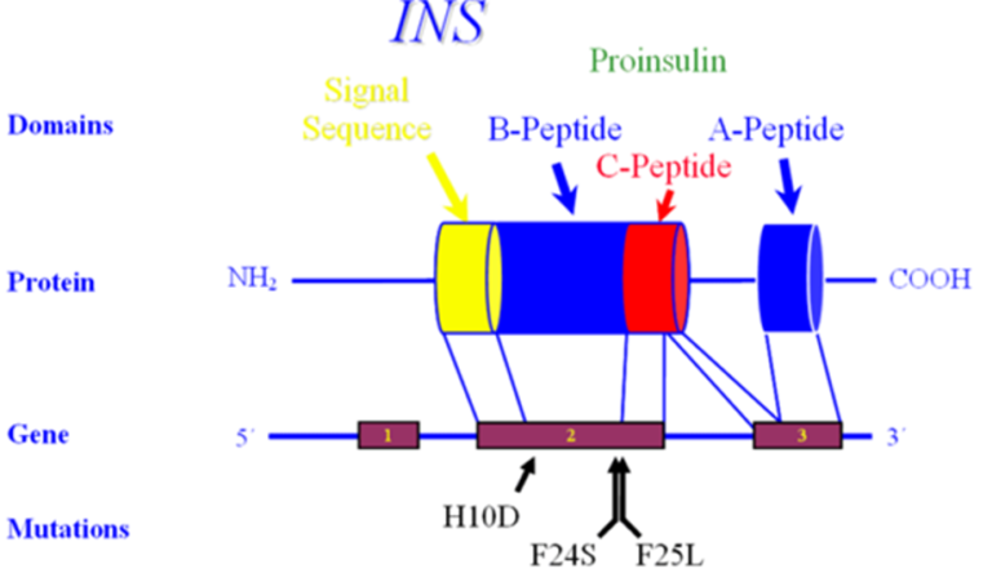
Figure 24. The insulin gene (INS).
Insulin Receptor
The insulin receptor is structurally related to the IGF1 receptor. The gene, INSR, is located on Chr 19p13.2 and contains 22 exons that span over 120 kilobases of genomic DNA (Figure 25). INSR encodes a transmembrane protein with tyrosine kinase activity which can transduce the signals of insulin, IGF1 and IGF2.
Individuals with a mutation in the insulin receptor have been identified and may be the basis for the mythological 'Leprechauns'. They typically have intrauterine growth retardation; small elfin facies with protuberant ears; distended abdomen; relatively large hands, feet, and genitalia; and abnormal skin with hypertrichosis, acanthosis nigricans, and decreased subcutaneous fat. At autopsy, several subjects have been found to have cystic changes in the membranes of gonads and hyperplasia of pancreatic islet cells. Severe mutations generally lead to death within months, but more mild mutations have been found in individuals with insulin resistance, hypoglycemia, acanthosis nigricans, normal subcutaneous tissue and may even be associated with a normal growth pattern! Individuals with even 'mild mutations' have been shown to have a thickened myocardium, enlarged kidneys and ovarian enlargement.
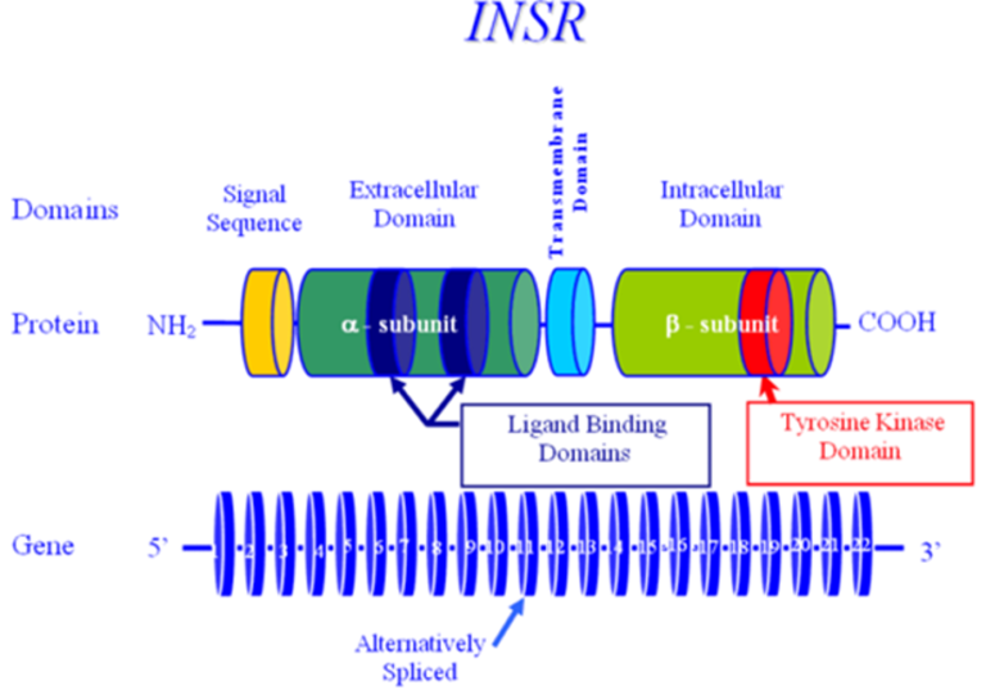
Figure 25. The insulin receptor gene (INSR).
SHORT STATURE WITH AN ADVANCED BONE AGE
Aggrecan
Aggrecan has also been shown to be involved in human height and the growth process. The aggrecan protein is a major constituent of the extracellular matrix of articular cartilage, where it forms large multimeric aggregates. The gene, ACAN, is located on Chr 15q26.1, comprising 19 exons spread over nearly 72 kilobases of genomic DNA. Exon 1 is approximately 13 kilobases upstream of exons 2-19, which comprise the coding portion of ACAN (158). ACANundergoes alternative splicing yielding several isoforms; the predominant isoform being 2132 amino acids long, with three globular domains (G1-3), an ‘interglobular’ (IG) domain, a keratan sulfate (KS) domain and a chondroitin sulfate (CS) domain, largely encoded in a modular fashion (Figure 26).
Domains G1 and G2 contain tandem repeat units rich in cysteine, which are necessary for disulfide bridging, the binding of hyaluronic acid and structural integrity, and are separated by the IGD, which provides a level of rigidity. The KS domain contains 11 copies of a six amino acid motif, while the chondroitin sulfate (CS) domain contains over 100 (non-tandem repeated) copies of the dipeptide Serine-Glycine). The G3 domain appears to function in maintaining proper protein folding and subsequent aggrecan secretion. The attachment of hyaluronic acid, keratan and chondroitin sulfate lead to significant water retention, which is largely responsible for the shock-absorbing character of articular cartilage. Aggrecan is also necessary for proper “chondroskeletal morphogenesis” (159), ensuring the proper organization and sequential maturation of the epiphysis.
In 1999, Kawaguchi reported a mutation in ACAN in subjects with lumbar disc herniation (160), then in 2005, both an autosomal dominant form of spondyloephiphyseal dysplasia (SED-Kimberly type) (161) and an autosomal recessive form (SED-Aggrecan type) (162) were shown to arise from mutations in ACAN.
In 2010, cases of autosomal dominant short stature with an advanced bone age were found to have mutations in ACAN, either with or without osteochondritis dissecans and/or (early-onset) osteoarthritis (163–167).
Dateki identified a family of four affected where three members had short stature with an advanced bone age, midface hypoplasia, joint problems and brachydactyly, while the fourth had lumbar disc herniation without other findings (168), attesting to phenotypic heterogeneity, even within a family.
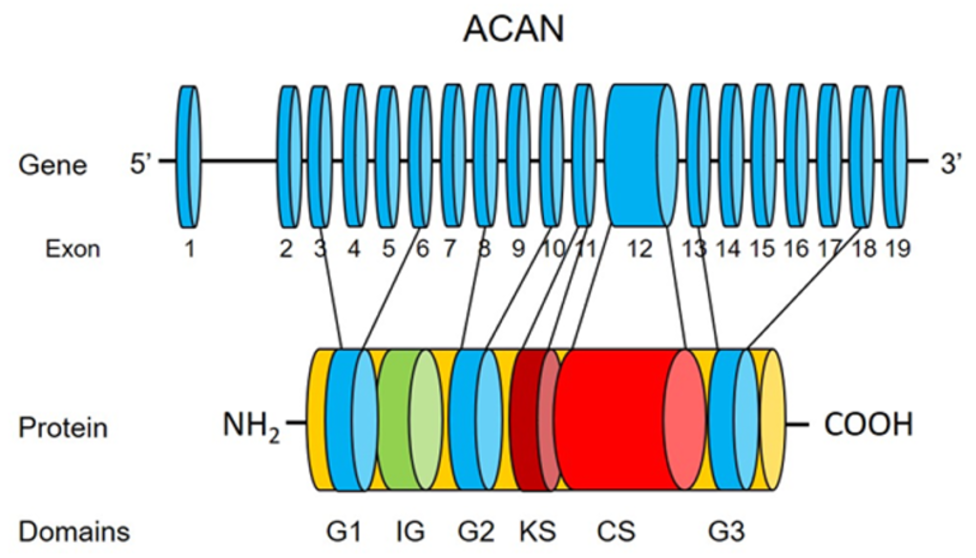
Figure 26. The aggrecan gene (ACAN).
The Short Stature Homeobox-Containing Gene (SHOX) Haploinsufficiency
The Short Stature Homeobox-containing gene (SHOX) was identified in the pseudo-autosomal region 1 on the distal end of the X and Y chromosomes at Xp22.3 and Yp11.3 (Figure 27) (169). Mutations in SHOX were observed in 60-100% of Léri-Weill dyschondrosteosis and Langer mesomelic dysplasia (170,171). Turner syndrome is almost always associated with the loss of SHOX gene because of numerical or structural aberration of X chromosome (172).The most common genetic defects leading to growth failure in humans are due to SHOX mutations with the incidence of 1:1,000 (173).
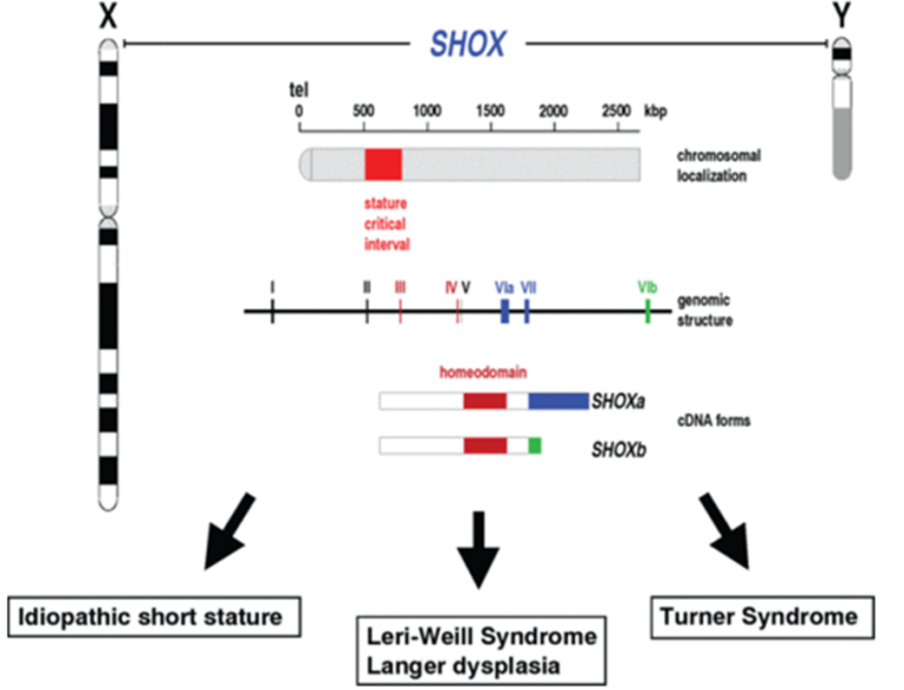
Figure 27. The Short Stature Homeobox-containing gene (SHOX). Reprinted with Permission. www.shox.uni.hd.de.
Genes in pseudoautosomal region 1 do not undergo X inactivation, therefore, healthy individuals express two copies of the SHOX gene, one from each of the sex chromosomes in both 46,XX and 46,XY individuals. The SHOX gene plays an important role in linear growth and is involved in the following:
- Intrauterine linear skeletal growth
- Fetal and childhood growth plate in a developmentally specificpattern and responsible for chondrocytedifferentiation and proliferation (174).
- A dose effect: SHOX haploinsufficiency is associated with short stature. In contrast, SHOX overdose as seen in sex chromosome polyploidy is associated with tall stature.
A large number of unique mutations (mostly deletions and point mutations) of SHOX have been described (170,172,175). SHOX abnormalities are associated with a broad phenotypic spectrum, ranging from short stature without dysmorphic signs as seen in idiopathic short stature (ISS) to profound Langer’s mesomelic skeletal dysplasia, a form of short stature characterized by disproportionate shortening of the middle segments of the upper arms (ulna) and lower legs (fibula) (176). In contrast to many other growth disorders such as growth hormone deficiency, SHOX deficiency is more common in girls.
Rappold et al developed a scoring system to determine the phenotypic spectrum of SHOX deficiency in children with short stature and identify patients for SHOX molecular testing (175). The authors recommend a careful examination including measurement of body proportions and X-ray of the lower legs and forearm before making the diagnosis of ISS. The scoring system consists of three anthropometric variables (arm span/height ratio, sitting height/height ratio and BMI), and five clinical variables (cubitus valgus, short forearm, bowing of forearm, muscular hypertrophy and dislocation of the ulna at the elbow). Based on the scoring system, authors recommend testing for SHOX deficiency for the individuals with a score greater than four or seven out of a total score of 24 (Table 7).
The recent data show that GH treatment is effective in improving linear growth of patients with SHOX mutations (176).
Noonan syndrome (NS) is a relatively common genetic disorder with the incidence of between 1:1000 and 1:4000 (177). NS is inherited in an autosomal dominant manner, and sporadic cases are not uncommon (50-60%) (178). NS is characterized by short stature, cardiac defects (most commonly pulmonary stenosis and hypertrophic cardiomyopathy), facial dysmorphism (down-slanting, antimongoloid palpebral fissures, ptosis, and low-set posteriorly rotated ears), webbed neck, mild mental retardation, cryptorchidism, feeding difficulties in infancy. The phenotype is variable between affected members of the same family and becomes milder with age (179).
Nearly 50% of patients with NS have gain-of-function mutations in protein tyrosine phosphatase nonreceptor type 11 (PTPN11), the gene encoding the cytoplasmic tyrosine phosphatase SHP-2, which regulates GH signaling by dephosphorylating STAT5b, resulting in down-regulation of GH activity (180). Mutations in four other genes (KRAS, SOS1, NF1 and RAF1) involved in RAS/MAPK signaling systems have been identified in patients with the NS phenotype and related disorders including LEOPORD, Costello, and cardio-facial-cutaneous syndromes (Figure 28) (181).
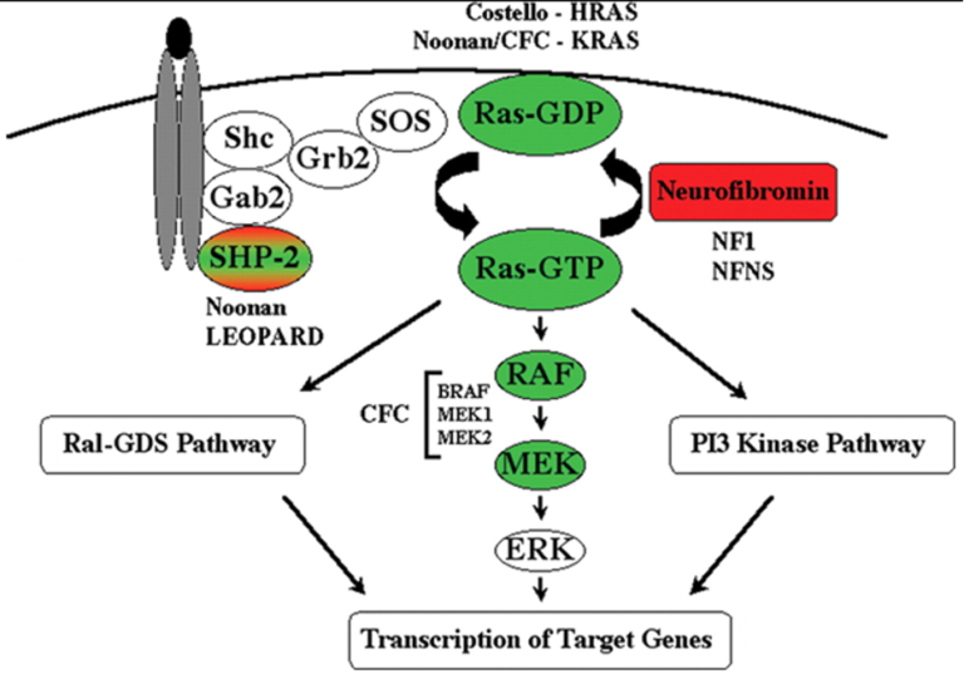
Figure 28. The RAS/MAPK signaling pathway. Reprinted with permission (181).
Although identifying these mutations has contributed to better understanding of the pathogenesis of NS, it appears that the genotype does not completely correlate with the phenotype, e.g. short stature in patients with NS. Several studies have shown that the subjects carrying gain of function mutations of PTPN11 had lower IGF1 levels, poor growth response, and resistance to GH therapy compared to subjects without PTPN11 mutations (182,183). However, data from one large study of individuals with NS did not demonstrate the same correlation between PTPN11 mutations and short stature (177). However, more recent studies showed significant improvement in final adult height in individuals with NS regardless of their mutation type (184,185).
DIAGNOSIS
Diagnosis of GH deficiency during childhood and adolescence is frequently challenging. Children whose height are below the 3rd percentile or -2 SD and have decreased growth velocity require clinical evaluation. Evaluation should begin with a detailed past medical history, family history, diet history, detailed review of prior growth data (including the initial post-natal period) and a thorough physical examination (186). Together, these should help the clinician identify the pattern and cause of growth failure, such as fetal growth restriction (e.g. SGA and IUGR), chronic illness, malnutrition/malabsorption, hypothyroidism, skeletal abnormalities or other identifiable syndromes, such as Turner syndrome. Once growth hormone deficiency is suspected, further testing of the hypothalamic-pituitary axes (including but not limited to the GH-IGF axis) along with radiological evaluation, should be performed (Table 8). It is important to note that the tests cannot be performed simultaneously, or in random order. Certain conditions (e.g. Hypothyroidism and Celiac disease) may mask the presence of others (e.g. GH deficiency), therefore requiring a stepwise approach with screening tests preceding specific examinations. Since growth failure generally occurs outside of GHD, only those children with signs or symptoms undergo expensive, invasive and non-physiologic GH provocative testing.
Table 8. Guidelines for Initial Clinical Evaluation of a Child with Growth Failure
|
Evaluation
|
Key elements
|
Birth history
|
Gestational age, birth weight and length, head circumference, delivery type, birth trauma, hypoglycemia, prolonged jaundice.
|
Past medical and surgical history
|
Head trauma, surgery, cranial radiation, CNS infection.
|
Review of systems
|
Appetite, eating habits, bowel movements, headache, vision change.
|
Chronic illness
|
Anemia, Inflammatory Bowel Disease, cardiovascular disease, renal insufficiency, etc.
|
Family history
|
Consanguinity, parents and siblings’ heights, family history of short stature, delayed puberty.
|
Physical examination
|
Body proportions (upper/lower segment ratios, arm span), head circumference, microphallus, dysmorphism, and midline craniofacial abnormalities.
|
Growth pattern
|
Crossing of percentiles, failure to catch-up.
|
Screening Tests
|
CBC, CMP, ESR/c-reactive protein, Celiac screening, TSH and Free T4, UA, IGF1, IGFBP3, Bone age (and a Karyotype for females)
|
Growth Charts
The growth pattern is a key element of growth assessment and is best studied by plotting growth data on an appropriate growth chart. US growth charts were developed from cross-sectional data provided by the National Center for Health Statistics and updated in 2000 (187), with body mass index included in this newest set. The supine length should be plotted for children from birth through age 3 years and standing height plotted when the child is old enough to stand, generally after 2 years of age. Ideally, growth data is determined by evaluating subjects at regular (optimally at 3 month) intervals, with the same stadiometer, and with the same individual obtaining the measurements, whenever possible. Three months is the minimal time interval needed between measurements to calculate a reliable growth velocity, and a six-to-twelve-month interval is optimal. Age and pubertal staging must be considered when evaluating the growth velocity, with the understanding that there is great individual variation in the onset and rate of puberty (188).
Deviations across height percentiles should be noted and evaluated further when confirmed, with the understanding that during the first two years of life, the crossing of length and/or weight percentiles may reflect catch-up or catch-down growth. Crossing percentiles during this period is not always physiological, and must be examined in the context of family, prenatal, birth and medical histories. Additionally, between two and three years of age, statural growth measurement changes from supine to erect, and may also introduce variation. Growth below the normal range (e.g.>-2SD) even without further deviation is consistent with (but not pathognomonic of) GH deficiency. Short stature with a low BMI suggests an abnormality of nutrition/GI tract (e.g. malnutrition, Celiac Disease, etc.), while short stature with an elevated BMI suggests hypothyroidism, Cushing’s syndrome or a central eating disorder, such as Prader-Willi syndrome, etc.
Figures 29-31 represent growth charts of children studied by the authors who have genetic defects leading to isolated growth hormone deficiency.
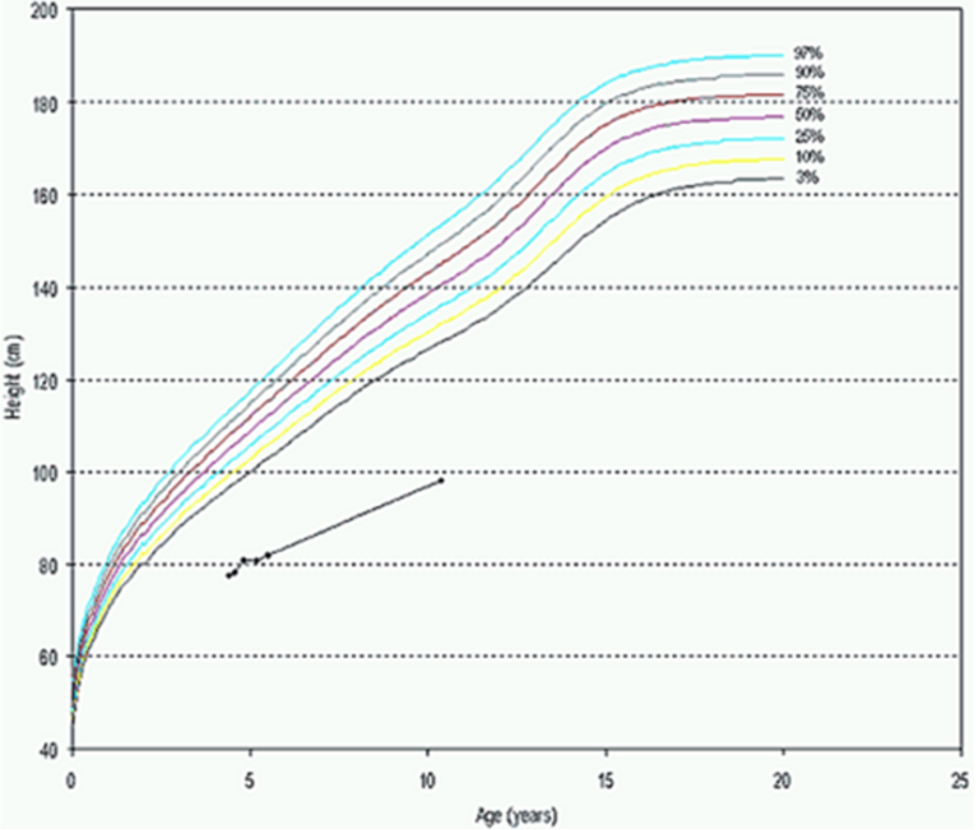
Figure 29. Growth pattern in children with isolated GH deficiency (Type 1A).
Growth failure can manifest as severe growth delay (Figure 29), gradual deceleration (“falling off the curve”) (Figure 30), or alternatively, maintaining a growth pattern parallel to the 3rd percentile, but without catch-up growth (Figure 31).
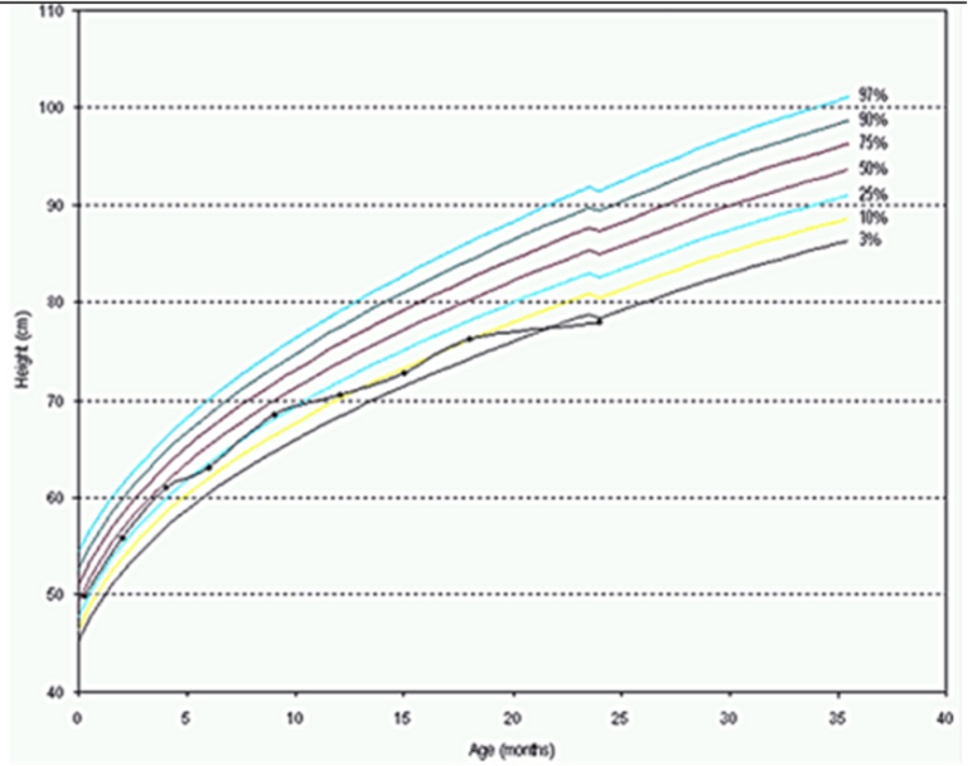
Figure 30. Growth pattern in children with isolated GH deficiency (Type 1B).
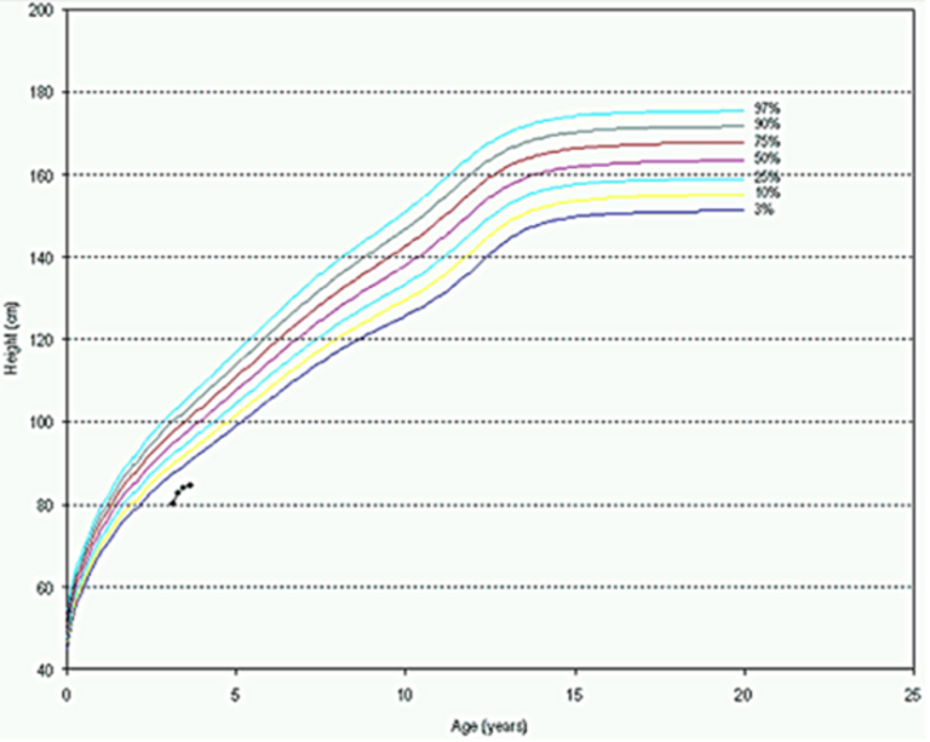
Figure 31. Growth pattern in children with isolated GH deficiency (Type 2).
Most children with GH deficiency have normal birth weight and length. However, in most cases, postnatal growth becomes severely compromised. This can be seen even in the first months of life. Although such children may show a normal growth pattern during the first 6 months, growth failure will eventually occur as GH takes on a more physiologically dominant role, and a child’s growth falls below the normal range.
Radiological Evaluation
The most commonly used system to assess skeletal maturity is to determine the ‘bone age’ of the left hand and wrist, using the method of Greulich and Pyle (189). Children younger than 2 years of age should have their bone age estimated from x-rays of the knee. Tanner and Whitehouse and their colleagues developed a scoring system for each of the hand bones as an alternative method to the method of Greulich and Pyle (190).
Adult height prediction methods estimate adult height by evaluating height at presentation relative to normative values for chronological or bone age. Such methods have been utilized for approximately 60 years (191) and are generally considered accurate in evaluating healthy children with a ‘normal’ growth potential (192,193). Several different methods have been produced and are currently in widespread use, including those of Bayley-Pinneau, the Tanner-Whitehouse-Marshall-Carter and Roche-Wainer-Thissen.
In 1946, Bayley initially described how final height could be estimated from the present height and the bone age, revising the method in 1952 to use the bone age assessment method of Greulich and Pyle (189). They developed what is commonly known as the predicted adult height (PAH) method of Bayley-Pinneau (BP). Tables have been developed for the BP method, listing the proportion of adult height attained at different bone ages, using longitudinal growth data on 192 healthy white children predominantly from North European ancestry in the US. Three tables – average, advanced and retarded – correct for possible differences between CA and BA of more than one year (194). The Bayley-Pinneau PAH method is applicable from age 8 years onwards.
Tanner, Whitehouse, Marshall and Carter developed an adult height prediction model based on current height, the mid-parental height, the age of menarche in girls and the ‘Tanner’ bone age (190). This PAH method (TW2) was developed on the longitudinal data of 211 healthy, British children. TW2 differs from the BP method in that the TW2 lowers the minimal age of prediction to 4 years, and allows for a quantitative effect of BA, while BP gives a semi-quantitative effect of bone age (i.e. delayed, normal or advanced).
The PAH method of Roche-Wainer-Thissen (RWT) was derived from longitudinal data on approximately 200 “normal” Caucasian American children in southwestern Ohio, at the Fels Research Institute (195). The RWT PAH method assesses the subject’s height, weight, BA and mid-parental height (MPH) and then applies regression techniques to determine the mathematical weighting to be applied to the four variables. The RWT method was designed to allow final height prediction from a single visit but is only applicable when greater than half of the bones are not fully mature.
Since both the bone age assessments and height prediction methods are created from healthy children (and often children from a single ethnic group and region), their use in ‘other’ populations is potentially inappropriate. In fact, Tanner et al state that their method is applicable to both boys and girls with short stature, but caution that “In clearly pathological children, such as those with endocrinopathies, they do not apply”. Similarly, Roche et al suggest caution when applying the RWT PAH method in ‘non-white and pathological populations’ (195). Zachmann et al reported that the RWT and TW2 methods (which are more BA-reliant) are better when growth potential is normal relative to the BA, however, in conditions with “…abnormal and incorrigible growth patterns…”, the BP method was more accurate, stating that with a “non-normal bone maturation to growth potential relationship, the ‘coefficient and regression equations’ (RWT and Tanner) cause an over-prediction of adult height” (196).
As stated above, these methods are based on healthy children and assume that the growth potential is directly proportional to the amount of time left prior to epiphyseal fusion as measured by the bone age. While this is correct for some of the children seen by the pediatric endocrinologist (e.g. healthy children, children with GH deficiency), it is not correct for many others with abnormal growth (e.g. children born SGA, children with idiopathic short stature, Turner syndrome and chronic renal failure). It is likely also inappropriate for children with an abnormal tempo of maturation (e.g.children with Russell-Silver syndrome, precocious puberty and congenital adrenal hyperplasia). In such children, standard growth prediction methods should be used only as ‘general guides’, if at all. Table 9 summarizes these 4 methods.
Table 9. Summary of Methods Used for PAH
|
Methods
|
Parameters
|
Bayley-Pinneau (BP)
|
Height, BA, CA
|
Tanner-Whitehouse- Marshall and Carter (TW2)
|
Height, BA, CA, MPH, the age of menarche in girls
|
Roche-Wainer-Thissen (RWT)
|
Height, weight, BA, MPH
|
Khamis-Roche
|
Height, weight, MPH
|
Biochemical Evaluation of GH Deficiency
As growth hormone is secreted in a pulsatile manner (usually 6 pulses in 24 hours and mainly during the night) with little serum GH at any given time, several methods have been developed to assess the adequacy of GH secretion:
- Stimulation testing: GH provocation utilizing arginine, clonidine, glucagon, L-Dopa, insulin, etc. This practice generally measures pituitary reserve-or GH secretory ability-rather than endogenous secretory status. It is labor intensive and trained individuals should perform the GH stimulation test according to a standardized protocol, with special care taken with younger children/infants (186).
- GH-dependent biochemical markers: IGF1 and IGFBP3: Values below a cut-off less than -2 SD for IGF1 and/or IGFBP3 strongly suggest an abnormality in the GH axis if other causes of low IGF have been excluded. Age and gender appropriate reference ranges for IGF1 and IGFBP3 are mandatory.
- 24-hour or Overnight GH sampling: Blood sampling at frequent intervals designed to quantify physiologic bursts of GH secretion.
- IGF generation test: This test is used to assess GH action and for the confirmation of suspected GH insensitivity. GH is given for several days (3-5 days) with serum IGF1 and IGFBP3 levels measured at the start and end of the test. A sufficient rise in IGF1 and IGFBP3 levels would exclude severe forms of GH insensitivity (103,188).
Failure to raise the serum GH level to the threshold level in response to provocation suggests the diagnosis of GH deficiency, while a low IGF1 and/or IGFBP3 level is supportive evidence. Although pharmacological GH stimulation tests have known deficiencies such as poor reproducibility, arbitrary cut-off limits, varying GH assays, side effects, and labor intensivity requiring trained staff, they remain the most easily available and accepted tools to evaluate pituitary GH secretory capacity. GH stimulation test results should be interpreted carefully in conjunction with pubertal status and body weight. Puberty and administration of sex steroids increase GH response to stimulation tests (197). To prevent false positive results, some centers use sex steroid priming in prepubertal children prior to GH stimulation testing (198). In obese children, the normal regulation of the GH/IGF1 axis is disturbed and GH secretion is decreased. IGF1 levels are very sensitive to nutritional status, while IGFBP3 are less so. Additionally, the normative range for IGF1 and IGFBP3 values are extremely wide, often with poor discrimination between normal and pathological. Age/pubertal stage and gender-specific threshold values must be utilized for both IGF1 and IGFBP3.
Due to the above limitations of current agents, the quest for new, more reliable agents that with fewer side effects and are less labor intensive has been ongoing. One of them is macimorelin acetate, a potent, orally administered growth hormone (GH) secretagogue approved by the FDA and European Medical Agency (EMA) for diagnosing adult growth hormone deficiency (199,200). It functions by increasing GH levels acutely via the ghrelin receptor GHSR1-a (199). Csákváry et al investigated the use of macimorelin as a diagnostic test in children with suspected GH deficiency, finding it to be safe and well-tolerated across different dosing cohorts (200). However, more research is needed to fully understand its efficacy in diagnosing pediatric GH deficiency and its potential therapeutic applications.
Growth hormone–releasing peptide-2 (GHRP2) is a potent stimulator of growth hormone secretion (201) and has been investigated its effectiveness diagnosing GH deficiency in children and adolescents (202). It is widely used in Japan for diagnosis of adult GH deficiency (203). GHRP2 also stimulates corticotropes along with somatotropes and maybe useful diagnosis of adrenal insufficiency which is concomitant with GHD in many hypothalamic-pituitary disorders (204).
Summary of Diagnosis of GH Deficiency
Children with severe GH deficiency can usually be diagnosed easily on clinical grounds and failed GH stimulation tests. Studies have shown that despite clinical evidence of GH deficiency, some children may pass GH stimulation tests (188). In the case of unexplained short stature, if the child meets most of the following criteria, a trial of GH treatment should be initiated (8):
- Height >2.25 SD below the mean for age or >2 SD below the mid-parental height percentile,
- Growth velocity <25th percentile for bone age,
- Bone age >2 SD below the mean for age,
- Height prediction is significantly below the mid-parental height,
- Low serum insulin-like growth factor 1 (IGF1) and/or insulin-like growth factor binding protein 3 (IGFBP3) for bone age and gender
- Other clinical features suggestive of GH deficiency.
Key elements that may indicate GH deficiency are:
- Height more than 2 SD below the mean.
- Neonatal hypoglycemia, microphallus, prolonged jaundice, or traumatic delivery.
- Although not required, a peak GH concentration after provocative GH testing of less than 10 ng/ml.
- Consanguinity and/or a family member with GH deficiency.
- Midline CNS defects, pituitary hypo- or aplasia, pituitary stalk agenesis, empty sella, ectopic posterior pituitary (bright spot’) on MRI.
- Deficiency of other pituitary hormones: TSH, PRL, LH/FSH and/or ACTH deficiency.
Many practitioners consider GH stimulation tests to be optional in the case of clinical evidence of GH deficiency, in patients with a history of surgery or irradiation of the hypothalamus/pituitary region and growth failure accompanied by additional pituitary hormone deficiencies. Similarly, children born SGA, with Turner syndrome, PWS and chronic renal insufficiency do not require GH stimulation testing before initiating GH treatment (8).
TREATMENT
The principal objective of GH treatment in children with GH deficiency is to improve final adult height. Human pituitary-derived GH was first used in children with hypopituitarism over 60 years ago, and abruptly ceased in 1985, after the first cases of Creutzfeld-Jacob disease were recognized. Since 1986, recombinant human GH (rhGH) has been the exclusive form of growth hormone used to treat GH deficiency in the United States and most of the world.
Short stature without overt growth hormone deficiency is very well described, and occurs in Turner Syndrome, renal failure, malnutrition, cardiovascular disease, Prader-Willi syndrome, small for gestational age, inflammatory bowel disease, and osteodystrophies- clearly representing the majority of short/poorly growing children in the world. Although not the focus of this discussion, it is important to realize that - in clinical terms - GH therapy is used to treat growth failure, rather than a biochemical GH deficiency. GH therapy in this setting, in combination with disease-specific treatments, generally improves statural growth and final adult height.
The primary goals of the treatment of a child with GH deficiency are to achieve normal height during childhood and to attain normal adult height. Children should be treated with an adequate dose of rhGH, with the dose tailored to that child’s specific condition. FDA guidelines for daily rhGH dose vary according to the indication and are given in Table 10(8).
Administration of daily rhGH in the evening is designed to mimic physiologic hGH secretion. Treatment is continued until adult height or epiphyseal closure (or both) has been recorded. GH therapy, however, should be continued throughout adulthood in the case of GHD, to optimize the metabolic effects of GH and to achieve normal peak bone mass-albeit at significantly lower “adult” doses. Adult GH replacement should only be started after retesting the individual and again demonstrating a failure to reach the new age-appropriate GH threshold, if appropriate.
Table 10. GH Dosage for Daily rhGH
|
Indication
|
Dose (mg/kg/wk)
|
GH Deficiency
Children Pre-pubertal
Pubertal
Adults
|
0.16 – 0.35
0.16 – 0.70
0.04 – 0.175
|
Turner Syndrome
|
0.375
|
Chronic renal insufficiency
|
0.35
|
Prader-Willi Syndrome
|
0.24
|
SGA
|
0.48
|
Idiopathic short stature (ISS)
|
0.3 – 0.37
|
SHOX Deficiency
|
0.35
|
Noonan Syndrome
|
0.23 – 0.46
|
The growth response to daily GH treatment is typically maximal in the first year of treatment and then gradually decreases over the subsequent years of treatment. First year growth response to rhGH is generally 200% of the pre-treatment velocity, and after several years, averages 150% of the baseline. Height improvements of 1 SD are typically achieved in children with GHD after two years of treatment, and between 2 and 2.5 SD after five or seven years.
GH doses are often increased if catch-up growth is inadequate and/or to compensate for the waning effect of rhGH with time. Cohen et al reported a significant improvement in HV when GH dose was adjusted based on IGF1 levels (205). However, GH dose was almost 3 times higher than mean conventional GH dose when IGF1 levels were titrated to the upper limit of normal. The lack of long-term safety data on high doses of GH and high circulating levels of IGF1 levels should be considered. Therefore, weight-based GH dosing is still recommended by many as the standard of care (206).
It is critically important to maximize height with GH therapy before the onset of puberty. Several investigators have advocated modifying puberty or the production of estrogens by the use of GnRH analogues (207–209) and aromatase inhibitors (210–213), respectively, in order to expand the therapeutic window for GH treatment, especially in older males.
The response to daily rhGH, however, may vary in children (214). Factors may affect the response to GH therapy including
- The etiology of short stature
- Age at the start of treatment
- Height deficit at the start of treatment
- GH dose and frequency
- Duration of treatment
- Genetic factors
Several studies have reported the association between response to GH therapy and a GHR gene polymorphism, the deletion of exon 3 (GHRd3). Although some reports showed better response to GH therapy in GHRd3 carriers with different clinical conditions including GHD, Turner syndrome, SGA, and ISS (93–95,215,216), many others failed to confirm positive effects of GHRd3 on response to GH treatment (96,217,218).
Daily GH injections can be inconvenient and lead to poor adherence over time in children, with a negative impact on their final adult height. To overcome these barriers, long-acting growth hormone (LAGH) formulations have been developed using various technologies including depot formulations, Pegylated formulations, pro-drug formulations, non-covalent albumin binding GH, and GH fusion proteins (219). It was noted that LAGH does not suppress endogenous GH secretion and can be used for treating non-GH deficient short stature, e.g., idiopathic short stature, with similar efficacy and safety compared to daily GH (220). In addition, the timing of LAGH administration does not appear to be a crucial factor in treatment efficacy, as no differences in growth rate or IGF1 concentrations were observed between morning and evening treatment schedules (221), this further improves compliance and ultimately adult height. In contrast, the typical bedtime administration of daily GH formulations may cause conflict during vacation, trip, or physical and social activities.
Currently, several long-acting growth hormones are available worldwide, utilizing pegylation, prodrug formulations, noncovalent transient albumin binding, and GH fusion proteins and only last three of them are FDA approved in the United States (222,223). The goal of these new formulations is to improve patient compliance while maintaining the efficacy and safety of traditional daily GH therapy.
Long-acting prodrug growth hormone formulations, such as lonapegsomatropin (Skytrofaâ), are designed to release unmodified growth hormone over an extended period, typically one week, while maintaining similar efficacy and safety profiles as daily injections (224,225). It received FDA approval in August 2021 for treating pediatric patients at least 1 year old with GH deficiency (226,227).
Lonapegsomatropin, also known as TransCon GH, consists of unmodified rhGH transiently attached to an inert carrier molecule, methoxypolyethylene glycol (mPEG), via a transient, low-molecular weight TransCon Linker (227). While the carrier molecule, mPEG, is responsible for decreasing GH receptor binding and renal excretion until its release, the linker determines when to release unmodified rhGH from its carrier prodrug, acts as a timer to allow controlled release of GH at body temperature and pH over one week (224,225,227). Lonapegsomatropin is administered subcutaneously as daily GH. Because it releases unmodified rhGH over one week under physiologic conditions, it has the same actions as endogenous GH. . In a phase 2 trial comparing TransCon GH to daily growth hormone in children with GHD, the mean annualized height velocity for TransCon GH was 12.9 cm/y compared to 11.6 cm/y for daily growth hormone at an equivalent dose (228). The subsequent phase 3 heiGHt Trial investigated the safety, tolerability, and efficacy of weekly lonapegsomatropin versus daily GH over 52 weeks in treatment-naive prepubertal children with GHD (225,229,230).This trial enrolled 161 treatment-naïve, prepubertal children with GHD. Subjects were randomized 2:1 to receive lonapegsomatropin 0.24 mg/kg/week or daily somatropin (0.34 mg/kg/week). At 52 weeks, annualized height velocity was again better in lonapegsomatropin group (11.2 cm/year) compared to daily GH group 10.3 cm/year (p=0.009). This study confirmed noninferiority and further showed statistical superiority of lonapegsomatropin in annualized height velocity compared to daily GH, with similar safety, in treatment-naïve children with GHD (230).
Lonapegsomatropin injections were well tolerated and were not associated with increased adverse effects compared to daily GH. None of study subjects had pseudotumor cerebri, slipped capital femoral epiphysis or hyperglycemia; in contrast, these complications have been previously reported in daily GH therapy (231). However, transient hyperglycemia was recently reported in a child with obesity who was on Lonepegsomatropin suggesting the need for careful glucose monitoring while receiving lonepegsomatropin (232). In the lonapegsomatropin group, although study subjects had higher IGF1 SD and reached target IGF1 SD earlier compared to daily GH-treated group, IGF1/IGFBP3 ratios were similar between the lonapegsomatropin- and daily GH-treated groups. There were two children that required dose reduction due to IGF1 SD >2. In both groups, there were no neutralizing antibodies and low-affinity antibodies were observed.
While the phase 3 heiGHt Trial in children established non-inferiority and statistical superiority of height velocity compared to daily growth hormone therapy, with no concerning side effects (230), the fliGHt Trial showed that switching from daily somatropin to lonapegsomatropin resulted in a similar adverse event profile and maintained growth outcomes (233). Long-term data from the enliGHten trial showed continued improvement in height standard deviation scores through the third year of therapy (234).
Somapacitan (Sogroyaâ) was developed using a well-established protraction method which previously has been used to extend half-lives of insulin detemir and glucagon like peptide 1 agonists (liraglutide and semaglutide). It is a novel albumin-binding GH derivative, with a small albumin-binding property attached to the GH molecule (235). Somapacitan consists of a human GH molecule and a single amino acid substitution at position 101 (leucine to cysteine) where a side chain has been attached (235). Amino acid substitution, Leu101Cys does not affect the binding to the GH receptor and the side chain consists of a hydrophilic spacer and an albumin-binding moiety (236). The reversible binding to endogenous albumin delays the elimination of somapacitan, extends its half-life and, therefore, duration of action, allowing once-weekly administration (237). Somapacitan was first approved for adult growth hormone deficiency in August 2020, and later for pediatric growth hormone deficiency in April 2023 by the US Food and Drug Administration.
Clinical trials have demonstrated somapacitan’s efficacy and safety compared to daily GH injections (238,239). In children with GHD, somapacitan showed similar height velocity and IGF1 increases as daily GH, with similar safety profiles including neutralizing antibodies, bone maturation, and metabolic profile over 1 year-2 years-and 4 years of treatment (238,240,241). In addition, authors assessed disease burden and treatment burden on both patients and parents/guardians, at years 2, 3, 4 which showed both groups strongly preferred weekly somapacitan over daily GH injections (241).
For adults with GHD, somapacitan improved body composition and IGF1 levels while being well-tolerated (242).Interestingly, somapacitan may have some advantages over daily GH. It showed neutral effects on glucose metabolism in adults with GHD, with no new cases of diabetes reported in long-term studies (243). In some patients switching from daily GH, somapacitan led to improvements in lipid metabolism and glucose tolerance, suggesting a potentially higher GH replacement effect (244). However, weekly injections may be easier to forget than daily ones for some patients (244).
Somatrogon (NGENLA®) consists of rhGH combined with three copies of the carboxy terminal peptide (CTP) from human chorionic gonadotropin resulting in a fusion protein of approximately 41 kDa (245). The addition of the CTP moieties extends the half-life of the attached rhGH, allowing for once-weekly subcutaneous administration (246). Somatrogon is the last LAGH that received FDA approval in June 2023 for the treatment of pediatric GH deficiency.
Clinical trials have demonstrated the efficacy and safety of somatrogon. In a phase 3 randomized trial, once-weekly somatrogon was non-inferior to once-daily human GH in increasing height velocity in pediatric patients with GHD (247).The mean height velocity at 12 months was 10.12 cm/yr for somatrogon compared to 9.78 cm/yr for daily GH (247).Interestingly, a comparison with published literature and the KIGS database indicated that children treated with once-weekly somatrogon (0.66 mg/kg/week) showed good growth compared to children treated with once-daily human growth hormone (248) and sustained improvement in height SDS and delta height SDS up to 5 years of treatment (249).
Similar to other LAGH formulations, somatrogon is generally well-tolerated, with injection site pain being the most frequent treatment-emergent adverse event (246). Furthermore, somatrogon had a lower treatment burden with favorable treatment experience than daily GH (233).
SUMMARY
In summary, long-acting growth hormone formulations represent a significant advancement in the treatment of growth hormone deficiency. While long-acting growth hormone formulations offer a promising alternative to daily growth hormone injections as well as improving treatment adherence and quality of life due to less frequent injections, there are some concerns regarding their unphysiological profile. Ongoing research and long-term studies are necessary to fully understand their efficacy, safety, and optimal use in clinical practice (250,251). Additionally, cost-effectiveness and the identification of patient groups that may benefit most from weekly injections are areas that require further investigation (250,252). Table 11 summarizes the approved dosage for long-acting growth hormone formulations.
Table 11. GH dosage for long-acting growth hormone
|
Medication
|
Dose (mg/kg/wk)
|
Somapacitan (Sogroyaâ)
|
0.16
|
Somatrogon (NGENLA®)
|
0.66
|
Lonapegsomatropin (Skytrofaâ)
|
0.24
|
Monitoring GH Treatment
Children receiving GH therapy require periodic monitoring. Three-month intervals are commonly chosen to allow for sufficient growth for a meaningful measurement, while minimizing time between dose adjustments/intervention. During follow up visits, height, weight, pubertal status, inspection of injection sites, and a comprehensive clinical exam should be initiated. In clinical practice, there are several parameters to monitor the response to GH treatment; the determination of the growth response (i.e. change in height velocity, ∆HV) being the most important parameter. These points are summarized in Table 12.
Table 12. Summary of Follow-Up Evaluation
|
Parameters
|
Assessment
|
Bone age
|
12-month intervals to assess the predicted height.
|
Thyroid Function Test
|
6-month intervals, or immediately, if growth velocity decreases.
|
Serum IGF1
|
Most useful in maintaining GH dose in ‘safe’ region. It does not necessarily correlate with growth velocity.
12-month intervals for daily rhGH.
However, timing to assess IGF-1 varies for each long-acting GH formulations. Monitoring of IGF-1 should be based on the mean IGF-1 over the week, which is on day 4 for somatrogon and somapacitan, and day 4.5 for lonapegsomatropin.
|
Metabolic panel, HbA1C
|
12-month intervals.
|
Dose adjustment
|
Should be based on weight-change, growth response, pubertal stage, comparison to predicted height at each visit, and IGF1/IGFBP3 levels.
|
Adverse Events
|
Every visit.
|
The Safety of GH Treatment
To date, multiple studies have demonstrated the safety of GH therapy (6,63,186,187,253–256). While rhGH treatment is generally considered safe, patients, however, should be monitored closely during treatment. Some of the common side effects seen during GH therapy are scoliosis, slipped capital femoral epiphysis (SCFE), pancreatitis, and pseudotumor cerebri (intracranial hypertension). An analysis of Genentech’s National Cooperative Growth Study (NCGS) identified eleven cases of adrenal insufficiency (AI) resulting in four deaths. All eleven cases of AI occurred in patients with organic GH deficiency (n=8,351), yielding an incidence of 132 per 100,000 in this subgroup, and an overall incidence of AI in NCGS was 20 per 100,000 (257).
Another concern is the use of GH in patients with Prader-Willi syndrome. Early recognition of the syndrome allows earlier intervention to prevent morbidity. Previous studies and data from KIGS showed that earlier initiation of GH treatment in children with PWS significantly improved body composition, muscle tone, growth, and cognition (258). Fatalities have been reported in patients with Prader-Willi syndrome during or after rhGH therapy (259). Data for children aged 3 years and older showed no statistically significant differences between the GH-treated and untreated groups with respect to cause of death, including respiratory infection or insufficiency (259,260). Although there is no clear evidence that those deaths are related to GH therapy, it was postulated that GH/IGF1 may worsen sleep apnea or hypoventilation via increasing tonsillar/adenoid tissue or worsen pre-existing impaired respiration by increasing volume load (261). However, studies on respiratory function of subjects with Prader-Willi syndrome during rhGH therapy have only demonstrated improved respiratory drive and function (262). In fact, a recent study showed that all subjects tested had abnormal sleep studies/parameters prior to initiating GH treatment, and that GH treatment resulted in an improvement in sleep apnea in the majority of patients with PWS. Importantly, however, a subset had worsening of sleep disturbance shortly after (6 wk) starting GH when also developing a respiratory infection (263). Because it is difficult to predict who will worsen with GH treatment, these authors recommend that patients with Prader-Willi syndrome have polysomnography before and 6 wk after starting rhGH and should be monitored for sleep apnea during upper respiratory tract infections. IGF1 levels should also be monitored.
The data on the efficacy and safety of GH treatment in 5220 Turner Syndrome (TS) children during the last 20 years has been reported by NCGS. The incidence of various side effects known to be associated with GH including pseudotumor cerebri, slipped capital femoral epiphysis, and scoliosis was increased in TS patients treated with GH compared with non-TS patients, however, children with TS are known to have a higher incidence of these side effects independent of rhGH treatment (264). Interestingly, type 1 diabetes was increased in GH treated group, most likely unrelated to GH treatment since the predisposition to autoimmune disorders is one of the characteristics of TS. In addition, NCGS data demonstrate a slightly increased incidence of a variety of malignancies in TS, however, this may again be related to the underlying condition, (i.e. not necessarily the rhGH treatment) as girls with TS have been shown to have an increased risk for cancer compared to general population (265). In summary, twenty years of experience in 5220 patients seems reassuring and does not indicate any new rhGH-related safety signals in the TS population (264).
There has been ongoing concern about tumorigenicity of chronically elevated IGF1 levels. It would therefore seem prudent to maintain IGF1 levels in the mid-normal range for age/pubertal stage and gender. Although the long-term consequences of elevated IGF1 levels during childhood are not known, some investigators recommend that dose reductions be considered if IGF1 levels are above the normal range (8). The report from the Safety and Appropriateness of Growth Hormone Treatments in Europe (SAGhE) in 2012, raised many concerns about the long-term safety of rhGH therapy in children. SAGhE is a large database established by eight European countries to evaluate the long-term safety of childhood GH treatment between 1980s and 1990s in 30,000 patients. Preliminary analysis of the patients in France revealed that among patients treated with rhGH, there was a 33% increased relative risk of mortality compared with French general population. They also noted an increased incidence of bone malignancies and cardiovascular disease (266). However, the data from the Belgian, Swedish the Dutch portions of SAGhE did not support or corroborate the findings that were reported from France (267).
Real and Theoretical adverse events of GH therapy are summarized in Table13.
Table 13. Real and Theoretical Adverse Events of GH Treatment
|
Side effects
|
Comment
|
Slipped capital femoral epiphysis (SCFE)
|
Unclear whether GH causes SCFE or if it is a result of diathesis and rapid growth induced by the GH. In addition, obesity, trauma, and previous radiation exposure increase the risk for SCFE. At each visit, patients should be evaluated for knee or hip pain/limp.
|
Pseudotumor cerebri
|
The mechanism is unclear, but it may be a result of GH induced salt and water retention within the CNS. Mostly occurs within the first months of treatment. It is more common in patients with organic GH deficiency, chronic renal insufficiency, and Turner Syndrome (257). Complaints of headache, nausea, dizziness, ataxia, or visual changes should be evaluated immediately.
|
Leukemia
|
Numerous large studies have not shown any association between rhGH and leukemia in children without predisposing conditions (254,257,268).
|
Recurrence risk of CNS tumors
|
Extensive studies did not support this possible side effect without risk factors (253,257,269–272)
|
Risk of primary malignancy
|
Studies have not shown a higher risk of all-site primary malignancy without a history of previous malignancy (273,274)
|
Insulin resistance
|
Insulin resistance is associated with GH therapy, though it is generally transient and/or reversible and rarely leads to overt diabetes. Patients with a limited insulin reserve may develop glucose intolerance. HbA1C should be monitored.
|
Pancreatitis
|
It may occur in patients with Turner syndrome, and associated risk factors (257).
|
Hypothyroidism
|
Almost 25 % of children may develop declines in serum T4 levels, generally reflecting enhanced conversion of T4 to T3, rather than outright hypothyroidism.
|
Transient gynecomastia
|
These are attributed to anabolic and metabolic effects of GH.
|
Scoliosis
|
It is more common in Turner syndrome and PWS. Patients should be evaluated for scoliosis at each visit and referred as appropriate
|
Adrenal Insufficiency
|
GH decreases the conversion of corticosterone to cortisol by a modulating effect on hepatic 11-beta hydroxysteroid dehydrogenase 1. Thus, endogenous cortisol levels can decrease in GHD patients after initiation of GH treatment. Furthermore, GH therapy may unmask previously unsuspected central ACTH deficiency. Whether the patients with hypopituitarism are on GH or not, they have a lifelong risk for adrenal insufficiency. Therefore, they should be monitored closely for adrenal insufficiency and their cortisol dose should be adjusted when GH therapy is started (257).
|
Sleep apnea/sleep disturbance
|
GH treatment might worsen sleep apnea/sleep disturbance in patients with Prader-Willi Syndrome, especially during a concomitant respiratory infection.
|
Transitioning GH Treatment from Childhood to Adulthood
Growing data support the need for continuation of GH treatment in individuals with childhood GH deficiency. GH treatment provides significant benefits in body composition, bone mineralization, lean body mass, lipid metabolism, and quality of life in adults with GH deficiency (275,276). However, identifying appropriate patients for transitioning from childhood to adult GH therapy remains challenging. The majority of children with a diagnosis of GHD and who are treated with GH do not have permanent GHD and will not require treatment during adulthood. Re-evaluation of GH secretory capacity is recommended after completion of linear growth in adolescents with history of childhood GHD (277). However, such re-evaluation requires cessation of GH treatment for at least one month. Furthermore, there is no established optimal GH stimulation test identified and validated during this transition period. The stimulation test results vary by protocol, and only a few secretagogues (insulin, arginine, and glucagon) are available to confirm GHD. The cut-off values are also stricter; the peak GH level to establish GHD is <6 mcg/L for the insulin tolerance test and ≤ 3 mcg/L for the glucagon test in young adults (278,279). It is generally agreed that patients with severe GHD secondary to organic defects (hypothalamic-pituitary abnormalities, tumors involving pituitary or hypothalamic area, infiltrative diseases, and cranial irradiation), genetic causes of GHD involving one or more additional pituitary hormone deficiencies and serum IGF-1 level below the normal range at least one month off therapy, are more likely to have permanent GHD and retesting to confirm GHD is unnecessary (275,279). However, children with idiopathic GHD are less likely to have permanent GHD. In a US study, only one third of patients with idiopathic GHD retested as GHD (280). In that cohort, authors found age <4 at diagnosis and IGFBP3 below -2.0 SDS were the strongest predictive factors (100% PPV) for permanent GHD. In contrast to previous studies (277), low IGF-1 (< -2.0 SDS) did not have significant power to identify permanent GHD unless IGF-1 level was extremely low (-5.3 SDS) (280).
In summary, current guidelines recommend the measurement of serum IGF-1 levels and a GH stimulation test after cessation of treatment for at least one month to determine whether the adolescents with childhood-onset GHD will need ongoing treatment unless they have known organic or genetic defects in the hypothalamic-pituitary region (275,276,279).
CONCLUSION
The control of human growth is becoming increasingly clear and involves both genetic and environmental factors. Many genes have been identified as being involved in normal growth and in the common phenotype of short stature. Genetic analyses, however, should follow a detailed clinical, biochemical and radiological assessment in order to refine the phenotype and point to the relevant pathophysiology, e.g. genes involved in the development and function of the pituitary gland (e.g. POU1F1), those involved in transducing the GH signal (e.g. STAT5B) or the IGF1 signal (e.g. PAPPA2) and everything further “downstream”, e.g. the growth plate, (e.g. SHOX). Clinical identification of patterns and unique findings can direct the genetic evaluation and narrow its focus – e.g. short stature with an advanced bone age may suggest mutations in the ACAN gene.
Identifying causes of short stature is challenging due to many factors, including the lack of ‘gold standard’ diagnostic criteria and limitations in available diagnostic tests (281). Clinical, biochemical and radiological evaluation continue to be the foundation for diagnosis. IGF1 and IGFBP3 measurements and neuroimaging play especially critical roles in defining the clinical phenotype, and in directing genetic testing to establish the diagnosis in children with abnormal growth and initiating appropriate treatment (281,282).
Treatment with rhGH has been shown to be effective and safe, improving linear growth and achieving adult heights in the normal range, as well as improving body composition, bone mineral density, cardiovascular outcomes, and quality of life (283–285). rhIGF1 may be considered for select children, especially those with primary severe IGF1 deficiency. The timing of treatment initiation and dosage are crucial factors in determining treatment outcomes (286). Additionally, continuing GH administration during the transition period between childhood and adulthood may be necessary for proper body composition, bone and muscle health, and metabolic parameters (287). Weekly GH preparations are now approved for the treatment of children with GH deficiency and offer the potential for reduced treatment burden, increased compliance and improved clinical outcomes (219,288). However, long-term safety concerns and non-physiological GH profiles, need to be carefully monitored and addressed in future studies; a growth hormone registry for daily and weekly GH preparations may help resolve these questions (250,289).
The understanding of growth remains complex, despite remarkable scientific advances in the last decades. Elucidation of new genetic factors, diagnostic tests and treatment options will provide us with a better understanding of the physiology of growth and should lead to improved diagnosis and treatment options, individualized to each patients’ unique situation.
REFERENCES
- Knobil E, Greep R. The physiology of growth hormone with particular reference to its action in the rhesus monkey and the’species specificity’problem. Recent Progr Horm Res. 1959;15(1).
- Ranke MB, Wit JM. Growth hormone - past, present and future. Nat Rev Endocrinol. 2018 May;14(5):285–300.
- Slonim AE, Bulone L, Damore MB, Goldberg T, Wingertzahn MA, McKinley MJ. A preliminary study of growth hormone therapy for Crohn’s disease. N Engl J Med. 2000;342(22):1633–7.
- Touati G, Prieur AM, Ruiz JC, Noel M, Czernichow P. Beneficial effects of one-year growth hormone administration to children with juvenile chronic arthritis on chronic steroid therapy. I. Effects on growth velocity and body composition. J Clin Endocrinol Metab. 1998;83(2):403–9.
- Hardin DS, Stratton R, Kramer JC, de la Rocha SR, Govaerts K, Wilson DP. Growth hormone improves weight velocity and height velocity in prepubertal children with cystic fibrosis. Horm Metab Res. 1998;30(10):636–41.
- Hardin DS, Ellis KJ, Dyson M, Rice J, McConnell R, Seilheimer DK. Growth hormone improves clinical status in prepubertal children with cystic fibrosis: results of a randomized controlled trial. J Pediatr. 2001;139(5):636–42.
- Gullett NP, Hebbar G, Ziegler TR. Update on clinical trials of growth factors and anabolic steroids in cachexia andwasting. Am J Clin Nutr. 2010 Apr;91(4):1143S-1147S.
- Wilson TA, Rose SR, Cohen P, Rogol AD, Backeljauw P, Brown R, et al. Update of guidelines for the use of growth hormone in children: the Lawson Wilkins Pediatric Endocrinology Society Drug and Therapeutics Committee. J Pediatr. 2003;143(4):415–21.
- Savage MO, Simon D, Czernichow PC. Growth hormone treatment in children on chronic glucorticoid therapy. Endocr Dev. 2011;20:194–201.
- Mauras N, George D, Evans J, Milov D, Abrams S, Rini A, et al. Growth hormone has anabolic effects in glucocorticosteroid-dependent children with inflammatory bowel disease: A pilot study. Metabolism. 2002;51(1):127–35.
- Lin-Su K, Vogiatzi MG, Marshall I, Harbison MD, Macapagal MC, Betensky B, et al. Treatment with growth hormone and LHRH analogue improves final adult height in children with congenital adrenal hyperplasia. J Clin Endocrinol Metab. 2005;jc.2004-2128.
- Rosenbloom AL. Mecasermin (recombinant human insulin-like growth factor I). Adv Ther. 2009;26(1):40.
- Larsen WJ. Human Embryology. Second. Churchhill Livingstone, Inc; 1997.
- De Rienzo F, Mellone S, Bellone S, Babu D, Fusco I, Prodam F, et al. Frequency of genetic defects in combined pituitary hormone deficiency: a systematic review and analysis of a multicentre Italian cohort. Clin Endocrinol. 2015;83(6):849–60.
- Phillips JA, Cogan JD. Genetic basis of endocrine disease. 6. Molecular basis of familial human growth hormone deficiency. J Clin Endocrinol Metab. 1994;78(1):11–6.
- Roessler E, Du Y-Z, Mullor JL, Casas E, Allen WP, Gillessen-Kaesbach G, et al. Loss-of-function mutations in the human GLI2 gene are associated with pituitary anomalies and holoprosencephaly-like features. Proc Natl Acad Sci U S A. 2003;100(23):13424–9.
- Roessler E, Ermilov AN, Grange DK, Wang A, Grachtchouk M, Dlugosz AA, et al. A previously unidentified amino-terminal domain regulates transcriptional activity of wild-type and disease-associated human GLI2. Hum Mol Genet. 2005;14(15):2181–8.
- França MM, Jorge AAL, Carvalho LRS, Costalonga EF, Vasques GA, Leite CC, et al. Novel heterozygous nonsense GLI2 mutations in patients with hypopituitarism and ectopic posterior pituitary lobe without holoprosencephaly. J Clin Endocrinol Metab. 2010;95(11):E384–91.
- Demiral M, Demirbilek H, Unal E, Durmaz CD, Ceylaner S, Ozbek MN. Ectopic posterior pituitary, polydactyly, midfacial hypoplasia and multiple pituitary hormone deficiency due to a novel heterozygous IVS11-2A>C(C.1957-2A>C) mutation in GLI2 gene. J Clin Res Pediatr Endocrinol. 2020;12(3):319-28.
- Netchine I, Sobrier ML, Krude H, Schnabel D, Maghnie M, Marcos E, et al. Mutations in LHX3 result in a new syndrome revealed by combined pituitary hormone deficiency. Nat Genet. 2000;25(2):182–6.
- Bhangoo APS, Hunter CS, Savage JJ, Anhalt H, Pavlakis S, Walvoord EC, et al. Clinical case seminar: a novel LHX3 mutation presenting as combined pituitary hormonal deficiency. J Clin Endocrinol Metab. 2006;91(3):747–53.
- Raetzman LT, Ward R, Camper SA. Lhx4 and Prop1 are required for cell survival and expansion of the pituitary primordia. Development. 2002;129(18):4229–39.
- Mullen RD, Colvin SC, Hunter CS, Savage JJ, Walvoord EC, Bhangoo APS, et al. Roles of the LHX3 and LHX4 LIM-homeodomain factors in pituitary development. Mol Cell Endocrinol. 2007 Feb;265–266:190–5.
- Sobrier ML, Attié-Bitach T, Netchine I, Encha-Razavi F, Vekemans M, Amselem S. Pathophysiology of syndromic combined pituitary hormone deficiency due to a LHX3 defect in light of LHX3 and LHX4 expression during early human development. Gene Expr Patterns. 2004;5(2):279–84.
- Machinis K, Pantel J, Netchine I, Leger J, Camand OJ, Sobrier ML, et al. Syndromic short stature in patients with a germline mutation in the LIM homeobox LHX4. Am J Hum Genet. 2001;69(5):961–8.
- Dattani MT, Martinez-Barbera JP, Thomas PQ, Brickman JM, Gupta R, Martensson IL, et al. Mutations in the homeobox gene HESX1/Hesx1 associated with septo-optic dysplasia in human and mouse. Nat Genet. 1998;19(2):125–33.
- Carvalho LR, Woods KS, Mendonca BB, Marcal N, Zamparini AL, Stifani S, et al. A homozygous mutation in HESX1 is associated with evolving hypopituitarism due to impaired repressor-corepressor interaction. J Clin Invest. 2003;112(8):1192–201.
- Parks JS, Brown MR, Hurley DL, Phelps CJ, Wajnrajch MP. Heritable disorders of pituitary development. J Clin Endocrinol Metab. 1999;84(12):4362–70.
- Thomas PQ, Dattani MT, Brickman JM, McNay D, Warne G, Zacharin M, et al. Heterozygous HESX1 mutations associated with isolated congenital pituitary hypoplasia and septo-optic dysplasia. Hum Mol Genet. 2001;10(1):39–45.
- Rosenbloom A l., Almonte AS, Brown MR, Fisher DA, Baumbach L, Parks JS. Clinical and biochemical phenotype of familial anterior hypopituitarism from mutation of the PROP1 gene. J Clin Endocrinol Metab. 1999;84(1):50–7.
- Turton JPG, Mehta A, Raza J, Woods KS, Tiulpakov A, Cassar J, et al. Mutations within the transcription factor PROP1 are rare in a cohort of patients with sporadic combined pituitary hormone deficiency (CPHD). Clin Endocrinol (Oxf). 2005;63(1):10–8.
- Pfäffle RW, DiMattia GE, Parks JS, Brown MR, Wit JM, Jansen M, et al. Mutation of the POU-specific domain of Pit-1 and hypopituitarism without pituitary hypoplasia. Science. 1992;257(5073):1118–21.
- Turton JPG, Reynaud R, Mehta A, Torpiano J, Saveanu A, Woods KS, et al. Novel mutations within the POU1F1 gene associated with variable combined pituitary hormone deficiency. J Clin Endocrinol Metab. 2005;90(8):4762–70.
- Dattani MT. Growth hormone deficiency and combined pituitary hormone deficiency: does the genotype matter? Clin Endocrinol (Oxf). 2005;63(2):121–30.
- Rizzoti K, Brunelli S, Carmignac D, Thomas PQ, Robinson IC, Lovell-Badge R. SOX3 is required during the formation of the hypothalamo-pituitary axis. Nat Genet. 2004;36(3):247–55.
- Foster JW, Graves JA. An SRY-related sequence on the marsupial X chromosome: implications for the evolution of the mammalian testis-determining gene. Proc Natl Acad Sci U S A. 1994;91(5):1927–31.
- Stevanović M, Lovell-Badge R, Collignon J, Goodfellow PN. SOX3 is an X-linked gene related to SRY. Hum Mol Genet. 1993;2(12):2013–8.
- Collignon J, Sockanathan S, Hacker A, Cohen-Tannoudji M, Norris D, Rastan S, et al. A comparison of the properties of Sox-3 with Sry and two related genes, Sox-1 and Sox-2. Development. 1996;122(2):509–20.
- Laumonnier F, Ronce N, Hamel BCJ, Thomas P, Lespinasse J, Raynaud M, et al. Transcription factor SOX3 is involved in X-Linked mental retardation with growth hormone deficiency. Am J Hum Genet. 2002;71(6):1450–5.
- Woods KS, Cundall M, Turton J, Rizotti K, Mehta A, Palmer R, et al. Over- and underdosage of SOX3 is associated with infundibular hypoplasia and hypopituitarism. Am J Hum Genet. 2005;76(5):833–49.
- Goosens M, Brauner R, Czernichow P, Duquesnoy P, Rapaport R. Isolated growth hormone (GH) deficiency type 1A associated with a double deletion in the human GH gene cluster. J Clin Endocrinol Metab. 1986;62(4):712–6.
- Wagner JK, Eblé A, Hindmarsh PC, Mullis PE. Prevalence of human GH-1 gene alterations in patients with isolated growth hormone deficiency. Pediatr Res. 1998;43(1):105–10.
- Wajnrajch MP, Gertner JM, Harbison MD, Chua SC, Leibel RL. Nonsense mutation in the human growth hormone releasing hormone receptor causes growth failure analogous to the little (lit) mouse. Nat Genet. 1996;12:88–90.
- Binder G, Nagel BH, Ranke MB, Mullis PE. Isolated GH deficiency (IGHD) type II: imaging of the pituitary gland by magnetic resonance reveals characteristic differences in comparison with severe IGHD of unknown origin. Eur J Endocrinol. 2002;147(6):755–60.
- Mullis PE, Robinson ICAF, Salemi S, Eble A, Besson A, Vuissoz J-M, et al. Isolated autosomal dominant growth hormone deficiency: An evolving pituitary deficit? A multicenter follow-up study. J Clin Endocrinol Metab. 2005;90(4):2089–96.
- Cogan JD, Ramel B, Lehto M, Phillips J, Prince M, Blizzard RM, et al. A recurring dominant negative mutation causes autosomal dominant growth hormone deficiency--a clinical research center study. J Clin Endocrinol Metab. 1995;80(12):3591–5.
- Takahashi Y, Shirono H, Arisaka O, Takahashi K, Yagi T, Koga J, et al. Biologically inactive growth hormone caused by an amino acid substitution. J Clin Invest. 1997;100(5):1159–65.
- McCarthy EM, Phillips JA. Characterization of an intron splice enhancer that regulates alternative splicing of human GH pre-mRNA. Hum Mol Genet. 1998;7(9):1491–6.
- Cogan JD, Prince MA, Lekhakula S, Bundey S, Futrakul A, McCarthy EM, et al. A novel mechanism of aberrant pre-mRNA splicing in humans. Hum Mol Genet. 1997;6(6):909–12.
- Hayashi Y, Yamamoto M, Ohmori S, Kamijo T, Ogawa M, Seo H. Inhibition of growth hormone (GH) secretion by a mutant GH-I gene product in neuroendocrine cells containing secretory granules: An implication for isolated GH deficiency inherited in an autosomal dominant manner. J Clin Endocrinol Metab. 1999;84(6):2134–9.
- Kamijo T, Hayashi Y, Seo H, Ogawa M. Hereditary isolated growth hormone deficiency caused by GH1 gene mutations in Japanese patients. Growth Horm IGF Res. 1999;9(Suppl B):31–4.
- Lee MS, Wajnrajch MP, Kim SS, Plotnick LP, Wang J, Gertner JM, et al. Autosomal dominant growth hormone (GH) deficiency type II: the Del32-71-GH deletion mutant suppresses secretion of wild-type GH. Endocrinology. 2000;141(3):883–90.
- Vivenza D, Guazzarotti L, Godi M, Frasca D, di Natale B, Momigliano-Richiardi P, et al. A novel deletion in the GH1 gene including the IVS3 branch site responsible for autosomal dominant isolated growth hormone deficiency. J Clin Endocrinol Metab. 2006;91(3):980–6.
- Fintini D, Salvatori R, Salemi S, Otten B, Ubertini G, Cambiaso P, et al. Autosomal-dominant isolated growth hormone deficiency (IGHD type II) with normal GH-1 gene. Horm Res. 2006;65(2):76–82.
- Veldhuis JD, Roemmich JN, Rogol AD. Gender and sexual maturation-dependent contrasts in the neuroregulation of growth hormone secretion in prepubertal and late adolescent males and females--a general clinical research center-based study. J Clin Endocrinol Metab. 2000;85(7):2385–94.
- van den Berg G, Veldhuis JD, Frölich M, Roelfsema F. An amplitude-specific divergence in the pulsatile mode of growth hormone (GH) secretion underlies, the gender difference in mean GH concentrations in men and premenapausal women. J Clin Endocrinol Metab. 1996;81(7):2460–7.
- Roemmich JN, Clark PA, Weltman A, Veldhuis JD, Rogol AD. Pubertal alterations in growth and body composition: IX. Altered spontaneous secretion and metabolic clearance of growth hormone in overweight youth. Metabolism. 2005;54(10):1374–83.
- Rivier J, Spiess J, Thorner M, Vale W. Characterization of a growth hormone-releasing factor from a human pancreatic islet tumour. Nature. 1982;300(5889):276–8.
- Gonzalez-Crespo S, Boronat A. Expression of the rat growth hormone-releasing hormone gene in placenta is directed by an alternative promoter. Proc Natl Acad Sci U S A. 1991;88(19):8749-53.
- Rogol AD, Blizzard RM, Foley TPJ, Furlanetto R, Selden R, Mayo K, et al. Growth hormone releasing hormone and growth hormone: Genetic studies in familial growth hormone deficiency. Pediatr Res. 1985;19:489–92.
- Mayo KE. Molecular cloning and expression of a pituitary-specific receptor for growth hormone-releasing hormone. Mol Endocrinol. 1992;6:1734–44.
- Godfrey P, Rahal JO, Beamer WG, Copeland NG, Jenkins NA, Mayo KE. GHRH receptor of little mice contains a missense mutation in the extracellular domain that disrupts receptor function. Nat Genet. 1993;4(3):227-32.
- Lin SC, Lin CR, Gukovsky I, Lusis AJ, Sawchenko PE, Rosenfeld MG. Molecular basis of the little mouse phenotype and implications for cell type-specific growth. Nature. 1993;364(6434):208–13.
- Wajnrajch MP, Chua SC, Green ED, Leibel RL. Human growth hormone-releasing hormone receptor (GHRHR) maps to a YAC at Chromosome 7p15. Mamm Genome. 1994;5(9):595.
- Baumann G, Maheshwari H. The dwarfs of Sindh:severe growth hormone (GH) deficiency caused by a mutation in the GH-releasing hormone receptor gene. Acta Pediatr Suppl. 1997;423:33–8.
- Netchine I, Talon P, Dastot F, Vitaux F, Goosens M, Amselem S. Extensive phenotypic analysis of a family with growth hormone (GH) deficiency caused by a mutation in the GH-releasing hormone receptor gene. J Clin Endocrinol Metab. 1998;83(2):432–6.
- Salvatori R, Hayashida CY, Aguiar-Oliveira MH, Phillips JA, Souza AH, Gondo RG, et al. Familial dwarfism due to a novel mutation of the growth hormone-releasing hormone receptor gene. J Clin Endocrinol Metab. 1999;84(3):917–23.
- Wajnrajch MP, Gertner JM, Harbison MD, Netchine I, Maheshwari HG, Baumann G, et al. Haplotype analysis of the growth hormone releasing hormone receptor locus in three apparently unrelated kindreds from the Indian subcontinent with the identical mutation in the GHRH receptor. Am J Med Genet. 2003;120A:77–83.
- Desai MP, Upadhye PS, Kamijo T, Yamamoto M, Ogawa M, Hayashi Y, et al. Growth hormone releasing hormone receptor (GHRH-r) gene mutation in Indian children with familial isolated growth hormone deficiency: a study from western India. J Pediatr Endocrinol Metab. 2005;18(10):955–73.
- Roelfsema F, Biermasz NR, Veldman RG, Veldhuis JD, Frolich M, Stovkis-Brantsma WH, et al. Growth hormone (GH) secretion in patients with an inactivating defect of the GH-releasing hormone (GHRH) receptor is pulsatile: evidence for a role for non-GHRH inputs into the generation of GH pulses. J Clin Endocrinol Metab. 2001;86(6):2459–64.
- MacIntyre I, Szelke M, Royal Postgraduate Medical S, Bowers CY, Chang J, Momany FA, et al. Effects of the enkephalins and enkephalin-analog on release of pituitary hormones in vitro. In: Molecular Endocrinology: Proceedings of Endocrinology ’77 held at the Royal College of Physicians, London, England on 11-15 July, 1977. New York: Elsevier/North-Holland Biomedical Press; 1977.
- Kojima M, Hosoda H, Date Y, Nakazato M, Matsuo H, Kangawa K. Ghrelin is a growth-hormone-releasing acylated peptide from stomach. Nature. 1999;402(6762):656–60.
- Wajnrajch MP, Gertner JM, Mullis PE, Cogan JD, Dannies PS, Kim S, et al. Arg183His, a new mutational hot-spot in the growth hormone gene causing isolated GH deficiency type II. J Endocr Genet. 2000;1(3):124–34.
- Howard AD, Feighner SD, Cully DF, Arena JP, Liberator PA, Rosenblum CI, et al. A receptor in pituitary and hypothalamus that functions in growth hormone release. Science. 1996;273:974–7.
- Takaya K, Ariyasu H, Kanamoto N, Iwakura H, Yoshimoto A, Harada M, et al. Ghrelin strongly stimulates growth hormone release in humans. J Clin Endocrinol Metab. 2000;85(12):4908–11.
- Bercu B, Walker RF. Novel growth hormone secretagogues: Clinical applications. Endocrinologist. 1997;7(1):51-64.
- Giustina A, Bonfanti C, Licini M, Ragni G, Stefana B. Hexarelin, a novel GHRP-6 analog, stimulates growth hormone (GH) release in a GH-secreting rat cell line (GH1) insensitive to GH-releasing hormone. Rugul Pept. 1997;70(1):49–54.
- Tolis G, Karydis I, Markousis V, Karagiorga M, Mesimeris T, Lenaerts V, et al. Growth hormone release by the novel GH releasing peptide Hexarelin in patients with homozygous b-Thalassemia. J Pediatr Endocrinol Metab. 1997;10(1):35–40.
- Hataya Y, Akamizu T, Takaya K, Kanamoto N, Ariyasu H, Saijo M, et al. A low dose of ghrelin stimulates growth hormone (gh) release synergistically with gh-releasing hormone in humans. J Clin Endocrinol Metab. 2001;86(9):45524555.
- Arvat E, Di Vito L, Broglio F, Papotti M, Muccioli G, Dieguez C, et al. Preliminary evidence that Ghrelin, the natural GH secretagogue (GHS)-receptor ligand, strongly stimulates GH secretion in humans. J Endocrinol Invest. 2000;23(8):493–5.
- Ghizzoni L, Mastorakos G, Vottero A, Ziveri M, Ilias I, Bernasconi S. Spontaneous growth hormone (GH) secretion is not directly affected by ghrelin in either short normal prepubertal children or children with GH neurosecretory dysfunction. J Clin Endocrinol Metab. 2004;89(11):5488–95.
- Racine MS, Symons K V, Foster CM, Barkan AL. Augmentation of growth hormone secretion after testosterone treatment in boys with constitutional delay of growth and adolescence: Evidence against an increase in hypothalamic secretion of growth hormone-releasing hormone. J Clin Endocrinol Metab. 2004;89(7):3326–31.
- Hirsh D, Heinrichs C, Leenders B, Wong ACK, Cummings DE, Chanoine J-P. Ghrelin is suppressed by glucagon and does not mediate glucagon-related growth hormone release. Horm Res. 2005;63(3):111–8.
- Zhang J V, Ren P-G, Avsian-Kretchmer O, Luo C-W, Rauch R, Klein C, et al. Obestatin, a peptide encoded by the ghrelin gene, opposes ghrelin’s effects on food intake. Science. 2005;310(5750):996–9.
- Ukkola O, Ravussin E, Jacobson P, Snyder EE, Chagnon M, Sjöström L, et al. Mutations in the preproghrelin/ghrelin gene associated with obesity in humans. J Clin Endocrinol Metab. 2001;86(8):3996–9.
- Pantel J, Legendre M, Cabrol S, Hilal L, Hajaji Y, Morisset S, et al. Loss of constitutive activity of the growth hormone secretagogue receptor in familial short stature. J Clin Invest. 2006;116:760–8.
- Grossman A, Savage MO, Lytras N, Preece MA, Sueiras-Diaz J, Coy DH, et al. Responses to analogues of growth hormone-releasing hormone in normal subjects, and in growth-hormone deficient children and young adults. Clin Endocrinol. 1984;21(3):321–30.
- Date Y, Murakami N, Kojima M, Kuroiwa T, Matsukura S, Kangawa K, et al. Central effects of a novel acylated peptide, ghrelin, on growth hormone release in rats. Biochem Biophys Res Commun. 2000;275(2):477–80.
- Shen LP, Pictet RL, Rutter WJ. Human somatostatin I: Sequence of the cDNA. Proc Natl Acad Sci USA. 1982;79(15):4575–9.
- Rosskopf D, Schurks M, Manthey I, Joisten M, Busch S, Siffert W. Signal transduction of somatostatin in human B lymphoblasts. Am J Physiol Cell Physiol. 2003;284(1):C179-190.
- Laron Z. Prismatic cases: Laron syndrome (primary growth hormone resistance) from patient to laboratory to patient. J Clin Endocrinol Metab. 1995;80(5):1526–31.
- Dastot F, Sobrier ML, Duquesnoy P, Duriez B, Goossens M, Amselem S. Alternatively spliced forms in the cytoplasmic domain of the human growth hormone (GH) receptor regulate its ability to generate a soluble GH-binding protein. Proc Natl Acad Sci U S A. 1996;93(20):10723–8.
- Dos Santos C, Essioux L, Teinturier C, Tauber M, Goffin V, Bougneres P. A common polymorphism of the growth hormone receptor is associated with increased responsiveness to growth hormone. Nat Genet. 2004;36(7):720–4.
- Binder G, Baur F, Schweizer R, Ranke MB. The d3-growth hormone (GH) receptor polymorphism is associated with increased responsiveness to GH in Turner syndrome and short small-for-gestational-age children. J Clin Endocrinol Metab. 2006;91(2):659–64.
- Jorge AAL, Marchisotti FG, Montenegro LR, Carvalho LR, Mendonca BB, Arnhold IJP. Growth hormone (GH) pharmacogenetics: Influence of GH receptor exon 3 retention or deletion on first-year growth response and final height in patients with severe GH deficiency. J Clin Endocrinol Metab. 2006;91(3):1076–80.
- Pilotta A, Mella P, Filisetti M, Felappi B, Prandi E, Parrinello G, et al. Common polymorphisms of the Growth Hormone (GH) Receptor do not correlate with the growth response to exogenous recombinant human GH in GH deficient children. J Clin Endocrinol Metab. 2006;91(3):jc.2005-1308.
- Rosenfeld RG, Belgorosky A, Camacho-Hubner C, Savage MO, Wit JM, Hwa V. Defects in growth hormone receptor signaling. Trends Endocrinol Metab. 2007;18(4):134–41.
- Savage MO, Hwa V, David A, Rosenfeld RG, Metherell LA. Genetic defects in the growth hormone-IGF-I axis causing growth hormone insensitivity and impaired linear growth. Front Endocrinol (Lausanne). 2011;2:95.
- Ayling RM, Ross R, Towner P, von Laue S, Finidori J, Moutoussamy S, et al. A dominant-negative mutation of the growth hormone receptor causes familial short stature. Nat Genet. 1997;16:13–4.
- Amselem S, Duquesnoy P, Attree O, Novelli G, Bousnina S, Postel-Vinay MC, et al. Laron dwarfism and mutations of the growth hormone-receptor gene. N Engl J Med. 1989;321(15):989–95.
- Woods KA, Fraser NC, Postel-Vinay MC, Savage MO, Clark AJ. A homozygous splice site mutation affecting the intracellular domain of the growth hormone (GH) receptor resulting in Laron syndrome with elevated GH-binding protein. J Clin Endocrinol Metab. 1996;81(5):1686–90.
- Woods KA, Dastot F, Preece MA, Clark AJ, Postel-Vinay MC, Chatelain PG, et al. Phenotype: genotype relationships in growth hormone insensitivity syndrome. J Clin Endocrinol Metab. 1997;82(11):3529–35.
- Salerno M, Balestrieri B, Matrecano E, Officioso A, Rosenfeld RG, Di Maio S, et al. Abnormal GH receptor signaling in children with idiopathic short stature. J Clin Endocrinol Metab. 2001;86(8):3882–8.
- Abuzzahab MJ, Schneider A, Goddard A, Grigorescu F, Lautier C, Keller E, et al. IGF-I receptor mutations resulting in intrauterine and postnatal growth retardation. N Engl J Med. 2003;349(23):2211–22.
- Bonioli E, Tarò M, Rosa C La, Citana A, Bertorelli R, Morcaldi G, et al. Heterozygous mutations of growth hormone receptor gene in children with idiopathic short stature. Growth Horm IGF Res. 2005;15(6):405–10.
- Rosenbloom AL, Guevara Aguirre J, Rosenfeld RG. The little women of Loja--growth hormone-receptor deficiency in an inbred population of southern Ecuador. N Engl J Med. 1990;323(20):1367–74.
- Aalbers AM, Chin D, Pratt KL, Little BM, Frank SJ, Hwa V, et al. Extreme elevation of serum growth hormone-binding protein concentrations resulting from a novel heterozygous splice site mutation of the growth hormone receptor gene. Horm Res Paediatr. 2009;71(5):276–84.
- Overgaard MT, Boldt HB, Laursen LS, Sottrup-Jensen L, Conover CA, Oxvig C. Pregnancy-associated plasma protein-A2 (PAPP-A2), a novel insulin-like growth factor-binding protein-5 proteinase. J Biol Chem. 2001;276(24):21849–53.
- Jepsen MR, Kløverpris S, Mikkelsen JH, Pedersen JH, Füchtbauer E-M, Laursen LS, et al. Stanniocalcin-2 inhibits mammalian growth by proteolytic inhibition of the insulin-like growth factor axis. J Biol Chem. 2015;290(6):3430–9.
- Fujimoto M, Hwa V, Dauber A. Novel modulators of the growth hormone - insulin-like growth factor axis: Pregnancy-associated plasma protein-A2 and stanniocalcin-2. J Clin Res Pediatr Endocrinol. 2017;9(Suppl 2):1–8.
- Woods KA, Camacho-Hübner C, Savage MO, Clark AJ. Intrauterine growth retardation and postnatal growth failure associated with deletion of the insulin-like growth factor I gene. N Engl J Med. 1996;335(18):1363–7.
- Baker J, Liu JP, Robertson EJ, Efstratiadis A. Role of insulin-like growth factors in embryonic and postnatal growth. Cell. 1993;75(1):73–82.
- Bonapace G, Concolino D, Formicola S, Strisciuglio P. A novel mutation in a patient with insulin-like growth factor 1 (IGF1) deficiency. J Med Genet. 2003;40(12):913–7.
- Walenkamp MJE, Karperien M, Pereira AM, Hilhorst-Hofstee Y, van Doorn J, Chen JW, et al. Homozygous and heterozygous expression of a novel insulin-like growth factor-I mutation. J Clin Endocrinol Metab. 2005;90(5):2855–64.
- Liu JP, Baker J, Perkins AS, Robertson EJ, Efstratiadis A. Mice carrying null mutations of the genes encoding insulin-like growth factor I (Igf-1) and type 1 IGF receptor (Igf1r). Cell. 1993;75(1):59–72.
- Morison IM, Reeve AE. Insulin-like growth factor 2 and overgrowth: molecular biology and clinical implications. Mol Med Today. 1998;4(3):110–5.
- Yakar S, Liu JL, Stannard B, Butler A, Accili D, Sauer B, et al. Normal growth and development in the absence of hepatic insulin-like growth factor I. Proc Natl Acad Sci U S A. 1999;96(13):7324–9.
- Sjögren K, Liu JL, Blad K, Skrtic S, Vidal O, Wallenius V, et al. Liver-derived insulin-like growth factor I (IGF-I) is the principal source of IGF-I in blood but is not required for postnatal body growth in mice. Proc Natl Acad Sci U S A. 1999;96(12):7088–92.
- Yakar S, Rosen CJ, Beamer WG, Ackert-Bicknell CL, Wu Y, Liu JL, et al. Circulating levels of IGF-1 directly regulate bone growth and density. J Clin Invest. 2002;110(6):771–81.
- Domené HM, Bengolea S V, Martínez AS, Ropelato MG, Pennisi P, Scaglia P, et al. Deficiency of the circulating insulin-like growth factor system associated with inactivation of the acid-labile subunit gene. N Engl J Med. 2004;350(6):570–7.
- Hwa V, Haeusler G, Pratt KL, Little BM, Frisch H, Koller D, et al. Total absence of functional acid labile subunit, resulting in severe insulin-like growth factor deficiency and moderate growth failure. J Clin Endocrinol Metab. 2006;91(5):1826–31.
- Domené HM, Scaglia PA, Lteif A, Mahmud FH, Kirmani S, Frystyk J, et al. Phenotypic effects of null and haploinsufficiency of acid-labile subunit in a family with two novel IGFALS gene mutations. J Clin Endocrinol Metab. 2007;92(11):4444–50.
- Domené HM, Hwa V, Jasper HG, Rosenfeld RG. Acid-labile subunit (ALS) deficiency. Best Pract Res Clin Endocrinol Metab. 2011;25(1):101–13.
- Savage MO, Camacho-Hübner C, David A, Metherell LA, Hwa V, Rosenfeld RG, et al. Idiopathic short stature: will genetics influence the choice between GH and IGF-I therapy? Eur J Endocrinol. 2007;157 Suppl:S33-37.
- Chan JM, Stampfer MJ, Giovannucci E, Gann PH, Ma J, Wilkinson P, et al. Plasma insulin-like growth factor-I and prostate cancer risk: A prospective study. Science. 1998;279(5350):563–6.
- Pollak M. Insulin-like growth factor physiology and cancer risk. Eur J Cancer. 2000;36(10):1224–8.
- Jernstrom H, Chu W, Vesprini D, Tao Y, Majeed N, Deal C, et al. Genetic factors related to racial variation in plasma levels of insulin-like growth factor-1: Implications for premenopausal breast cancer risk. Mol Genet Metab. 2001;72(2):144–54.
- Cohen P, Clemmons DR, Rosenfeld RG. Does the GH-IGF axis play a role in cancer pathogenesis? Growth Horm IGF Res. 2000;10(6):297–305.
- Cohen P. Overview of the IGF-I system. Horm Res. 2006;65(Suppl 1):3–8.
- Ranke MB. Defining insulin-like growth factor-I deficiency. Horm Res. 2006;65(Suppl 1):9–14.
- Savage MO, Camacho-Hübner C, Dunger DB. Therapeutic applications of the insulin-like growth factors. Growth Horm IGF Res. 2004;14(4):301–8.
- Laron Z, Anin S, Klipper-Aurbach Y, Klinger B. Effects of insulin-like growth factor on linear growth, head circumference, and body fat in patients with Laron-type dwarfism. Lancet. 1992;339(8804):1258–61.
- Ranke MB, Savage MO, Chatelain PG, Preece MA, Rosenfeld RG, Blum WF, et al. Insulin-like growth factor I improves height in growth hormone insensitivity: two years’ results. Horm Res. 1995;44(6):253–64.
- Ranke MB, Savage MO, Chatelain PG, Preece MA, Rosenfeld RG, Wilton P. Long-term treatment of growth hormone insensitivity syndrome with IGF-I. Results of the European Multicentre Study. The Working Group on Growth Hormone Insensitivity Syndromes. Horm Res. 1999;51(3):128–34.
- Collett-Solberg PF, Misra M, Drug and Therapeutics Committee of the Lawson Wilkins Pediatric Endocrine Society. The role of recombinant human insulin-like growth factor-I in treating children with short stature. J Clin Endocrinol Metab. 2008;93(1):10–8.
- Laron Z. Insulin-like growth factor-I (lGF-l): safety and efficacy. Pediatr Endocrinol Rev. 2004;2(Suppl 1):78–85.
- Backeljauw PF, Underwood LE, GHIS Collaborative Group. Therapy for 6.5-7.5 years with recombinant insulin-like growth factor I in children with growth hormone insensitivity syndrome: A clinical research center study. J Clin Endocrinol Metab. 2001;86(4):1504–10.
- Roback EW, Barakat AJ, Dev VG, Mbikay M, Chretien M, Butler MG. An infant with deletion of the distal long arm of chromosome 15 (q26.1----qter) and loss of insulin-like growth factor 1 receptor gene. Am J Med Genet. 1991;38(1):74–9.
- Fang P, Schwartz ID, Johnson BD, Derr MA, Roberts CT, Hwa V, et al. Familial short stature caused by haploinsufficiency of the insulin-like growth factor i receptor due to nonsense-mediated messenger ribonucleic acid decay. J Clin Endocrinol Metab. 2009;94(5):1740–7.
- Fujimoto M, Andrew M, Dauber A. Disorders caused by genetic defects associated with GH-dependent genes: PAPPA2 defects. Mol Cell Endocrinol. 2020 Dec;518:110967.
- Dauber A, Muñoz-Calvo MT, Barrios V, Domené HM, Kloverpris S, Serra-Juhé C, et al. Mutations in pregnancy-associated plasma protein A2 cause short stature due to low IGF-I availability. EMBO Mol Med. 2016 Apr;8(4):363–74.
- Babiker A, Al Noaim K, Al Swaid A, Alfadhel M, Deeb A, Martín-Rivada Á, et al. Short stature with low insulin-like growth factor 1 availability due to pregnancy-associated plasma protein A2 deficiency in a Saudi family. Clin Genet. 2021 Nov;100(5):601–6.
- Barrios V, Chowen JA, Martín-Rivada Á, Guerra-Cantera S, Pozo J, Yakar S, et al. Pregnancy-associated plasma protein (PAPP)-A2 in physiology and disease. Cells. 2021;10(12):3576.
- Muñoz-Calvo MT, Barrios V, Pozo J, Chowen JA, Martos-Moreno GÁ, Hawkins F, et al. Treatment With recombinant human insulin-like growth factor-1 improves growth inpatients with PAPP-A2 deficiency. J Clin Endocrinol Metab. 2016;101(11):3879–83.
- Martín-Rivada Á, Barrios V, Martínez Díaz-Guerra G, Pozo J, Martos-Moreno GÁ, Argente J. Adult height and long-term outcomes after rhIGF-1 therapy in two patients withPAPP-A2 deficiency. Growth Horm IGF Res. 2021;60–61:101419.
- Muthuvel G, Dauber A, Alexandrou E, Tyzinski L, Andrew M, Hwa V, et al. Five-year therapy with recombinant human insulin-like growth factor-1 in a patient with PAPP-A2 deficiency. Horm Res Paediatr. 2023;96(5):449–57.
- Cabrera-Salcedo C, Mizuno T, Tyzinski L, Andrew M, Vinks AA, Frystyk J, et al. Pharmacokinetics of IGF-1 in PAPP-A2-deficient patients, growth response, and effects on glucose and bone density. J Clin Endocrinol Metab. 2017;102(12):4568–77.
- Hawkins-Carranza FG, Muñoz-Calvo MT, Martos-Moreno GÁ, Allo-Miguel G, Del Río L, Pozo J, et al. rhIGF-1 treatment increases bone mineral density and trabecular bone structure in children with PAPP-A2 deficiency. Horm Res Paediatr. 2018;89(3):200–4.
- Andrew M, Liao L, Fujimoto M, Khoury J, Hwa V, Dauber A. PAPPA2 as a therapeutic modulator of IGF-I bioavailability: in vivo and in vitro evidence. J Endocr Soc. 2018;2(7):646–56.
- Fitzpatrick GV, Soloway PD, Higgins MJ. Regional loss of imprinting and growth deficiency in mice with a targeted deletion of KvDMR1. Nat Genet. 2002;32(3):426–31.
- Eggenschwiler J, Ludwig T, Fisher P, Leighton PA, Tilghman SM, Efstratiadis A. Mouse mutant embryos overexpressing IGF-II exhibit phenotypic features of the Beckwith-Wiedemann and Simpson-Golabi-Behmel syndromes. Genes Dev. 1997;11(23):3128–42.
- McElreavey K, Fellous M. Sex-determining genes. Trends Endocrinol Metab. 1997;8(9):342–5.
- Fisher AM, Thomas NS, Cockwell A, Stecko O, Kerr B, Temple IK, et al. Duplications of chromosome 11p15 of maternal origin result in a phenotype that includes growth retardation. Hum Genet. 2002;111(3):290–6.
- Gicquel C, Rossignol S, Cabrol S, Houang M, Steunou V, Barbu V, et al. Epimutation of the telomeric imprinting center region on chromosome 11p15 in Silver-Russell syndrome. Nat Genet. 2005;37(9):1003–7.
- Schönherr N, Meyer E, Eggermann K, Ranke MB, Wollmann HA, Eggermann T. (Epi)mutations in 11p15 significantly contribute to Silver-Russell syndrome: but are they generally involved in growth retardation? Eur J Med Genet. 2006;49(5):414–8.
- Hannula K, Lipsanen-Nyman M, Kontiokari T, Kere J. A narrow segment of maternal uniparental disomy of chromosome 7q31-qter in Silver-Russell syndrome delimits a candidate gene region. Am J Hum Genet. 2001;68(1):247–53.
- Murphy R, Baptista J, Holly J, Umpleby AM, Ellard S, Harries LW, et al. Severe intrauterine growth retardation and atypical diabetes associated with a translocation breakpoint disrupting regulation of the insulin-like growth factor 2 gene. J Clin Endocrinol Metab. 2008;93(11):4373–80.
- Valhmu WB, Palmer GD, Rivers PA, Ebara S, Cheng JF, Fischer S, et al. Structure of the human aggrecan gene: Exon-intron organization and association with the protein domains. Biochem J. 1995;309(2):535–42.
- Kiani C, Chen L, Wu YJ, Yee AJ, Yang BB. Structure and function of aggrecan. Cell Res. 2002;12:19.
- Kawaguchi Y, Osada R, Kanamori M, Ishihara H, Ohmori K, Matsui H, et al. Association between an aggrecan gene polymorphism and lumbar disc degeneration. Spine. 1999;24:2456–60.
- Gleghorn L, Ramesar R, Beighton P, Wallis G. A mutation in the variable repeat region of the aggrecan gene (AGC1) causes a form of spondyloepiphyseal dysplasia associated with severe, premature osteoarthritis. Am J Hum Genet. 2005;77:484–90.
- Tompson SW, Merriman B, Funari VA, Fresquet M, Lachman RS, Rimoin DL, et al. A recessive skeletal dysplasia, SEMD aggrecan type, results from a missense mutation affecting the C-type lectin domain of aggrecan. Am J Hum Genet. 2009;84:72–9.
- Stattin E-L, Wiklund F, Lindblom K, Önnerfjord P, Jonsson B-A, Tegner Y, et al. A missense mutation in the aggrecan c-type lectin domain disrupts extracellular matrix interactions and causes dominant familial osteochondritis dissecans. Am J Hum Genet. 2010;86(2):126–37.
- Nilsson O, Guo MH, Dunbar N, Popovic J, Flynn D, Jacobsen C, et al. Short stature, accelerated bone maturation, and early growth cessation due to heterozygous aggrecan mutations. J Clin Endocrinol Metab. 2014;99(8):E1510–8.
- Quintos JB, Guo. MH, Dauber A. Idiopathic short stature due to novel heterozygous mutation of the aggrecan gene. J Pediatr Endocrinol Metab. 2015;28(7-8):927–32.
- Gkourogianni A, Andrew M, Tyzinski L, Crocker M, Douglas J, Dunbar N, et al. Clinical characterization of patients with autosomal dominant short stature due to aggrecan mutations. J Clin Endocrinol Metab. 2017;102(2):460–9.
- van der Steen M, Pfundt R, Maas SJWH, Bakker-van Waarde WM, Odink RJ, Hokken-Koelega ACS. ACAN gene mutations in short children born SGA and response to growth hormone treatment. J Clin Endo Metab. 2017;102:1458–67.
- Dateki S, Nakatomi A, Watanabe S, Shimizu H, Inoue Y, Baba H, et al. Identification of a novel heterozygous mutation of the aggrecan gene in a family with idiopathic short stature and multiple intervertebral disc herniation. J Hum Genet. 2017;62:717–21.
- Rao E, Weiss B, Fukami M, Rump A, Niesler B, Mertz A, et al. Pseudoautosomal deletions encompassing a novel homeobox gene causes growth failure in idiopathic short stature and Turner syndrome. Nat Genet. 1997;16:54–62.
- Huber C, Rosilio M, Munnich A, Cormier-Daire V. High incidence of SHOX anomalies in individuals with short stature. J Med Genet. 2006;43(9):735–9.
- Jorge AAL, Nishi MY, Funari MFA, Souza SC, Arnhold IJP, Mendonça BB. [Short stature caused by SHOX gene haploinsufficiency: from diagnosis to treatment]. Arq Bras Endocrinol Metabol. 2008;52(5):765–73.
- Binder G. Short stature due to SHOX deficiency: genotype, phenotype, and therapy. Horm Res Paediatr. 2011;75(2):81–9.
- Marchini A, Rappold G, Schneider KU. SHOX at a glance: From gene to protein. Arch Physiol Biochem. 2007;113(3):116–23.
- Munns CJF, Haase HR, Crowther LM, Hayes MT, Blaschke R, Rappold G, et al. Expression of SHOX in human fetal and childhood growth plate. J Clin Endocrinol Metab. 2004;89(8):4130–5.
- Rappold G, Blum WF, Shavrikova EP, Crowe BJ, Roeth R, Quigley CA, et al. Genotypes and phenotypes in children with short stature: Clinical indicators of SHOX haploinsufficiency. J Med Genet. 2007;44(5):306–13.
- Blum WF, Crowe BJ, Quigley CA, Jung H, Cao D, Ross JL, et al. Growth hormone is effective in treatment of short stature associated with short stature homeobox-containing gene deficiency: Two-year results of a randomized, controlled, multicenter trial. J Clin Endocrinol Metab. 2007;92(1):219–28.
- Shaw AC, Kalidas K, Crosby AH, Jeffery S, Patton MA. The natural history of Noonan syndrome: A long-term follow-up study. Arch Dis Child. 2007;92(2):128–32.
- Allanson JE. Noonan syndrome. J Med Genet. 1987;24(1):9–13.
- Allanson JE, Hall JG, Hughes HE, Preus M, Witt RD. Noonan syndrome: The changing phenotype. Am J Med Genet. 1985;21(3):507–14.
- Tartaglia M, Mehler EL, Goldberg R, Zampino G, Brunner HG, Kremer H, et al. Mutations in PTPN11, encoding the protein tyrosine phosphatase SHP-2, cause Noonan syndrome. Nat Genet. 2001;29(4):465–8.
- Gelb BD, Tartaglia M. Noonan syndrome and related disorders: dysregulated RAS-mitogen activated protein kinase signal transduction. Hum Mol Genet. 2006;15 Spec No:R220-226.
- Ferreira LV, Souza SAL, Arnhold IJP, Mendonca BB, Jorge AAL. PTPN11 (protein tyrosine phosphatase, nonreceptor type 11) mutations and response to growth hormone therapy in children with Noonan syndrome. J Clin Endocrinol Metab. 2005;90(9):5156–60.
- Limal J-M, Parfait B, Cabrol S, Bonnet D, Leheup B, Lyonnet S, et al. Noonan syndrome: relationships between genotype, growth, and growth factors. J Clin Endocrinol Metab. 2006;91(1):300–6.
- Raaijmakers R, Noordam C, Karagiannis G, Gregory JW, Hertel NT, Sipilä I, et al. Response to growth hormone treatment and final height in Noonan syndrome in a large cohort of patients in the KIGS database. J Pediatr Endocrinol Metab. 2008;21(3):267–73.
- Noordam C, Peer PGM, Francois I, De Schepper J, van den Burgt I, Otten BJ. Long-term GH treatment improves adult height in children with Noonan syndrome with and without mutations in protein tyrosine phosphatase, non-receptor-type 11. Eur J Endocrinol. 2008;159(3):203–8.
- Growth Hormone Research Society. Consensus guidelines for the diagnosis and treatment of growth hormone (GH) deficiency in childhood and adolescence: summary statement of the GH Research Society. GH Research Society. J Clin Endocrinol Metab. 2000;85(11):3990–3.
- Kuczmarski RJ, Ogden C, Grummer-Strawn LM, Guo SS, Wei R, Mei Z, et al. CDC growth charts: United States. Hyattsville: U.S. Department of Health and Human Services. Centers for Disease Control and Prevention. National Center for Health Statistics; 2000.
- Sizonenko PC, Clayton PE, Cohen P, Hintz RL, Tanaka T, Laron Z. Diagnosis and management of growth hormone deficiency in childhood and adolescence. Part 1: Diagnosis of growth hormone deficiency. Growth Horm IGF Res. 2001;11(3):137–65.
- Gruelich WW, Pyle SI. Radiographic atlas of skeletal development of the hand and wrist. Stanford University Press; 1959.
- Tanner JM. Assessment of skeletal maturity and prediction of adult height (TW2 method). Tanner JM, editor. London ; New York: Academic Press ; 1983.
- Bayley N. Tables for predicting adult height from present height and skeletal age. J Pediat. 1946;28:49.
- Rosenfield R, Cutler L, Jameson JL, DeGroot L, Burger H. Somatic growth and maturation. In: Endocrinology. Philadelphia: W.B. Saunders Co.; 2001. p. 477-502.
- Underwood LE, Van Wyk JJ. Normal and aberrant growth. In: Williams Textbook Of Endocrinology. Philadelphia: W.B. Saunders Co.; 2003. p. 1079–138.
- Bayley N, Pinneau SR. Tables for predicting adult height from skeletal age: Revised for use with the Greulich-Pyle hand standards. J Pediatr. 1952;40(4):423–41.
- Roche AF, Wainer H, Thissen D. The RWT method for the prediction of adult stature. Pediatrics. 1975;56(6):1026–33.
- Zachmann M, Sobradillo B, Frank M, Frisch H, Prader A. Bayley-Pinneau, Roche-Wainer-Thissen, and Tanner height predictions in normal children and in patients with various pathologic conditions. J Pediatr. 1978;93(5):749–55.
- Marin G, Domené HM, Barnes KM, Blackwell BJ, Cassorla FG, Cutler GB. The effects of estrogen priming and puberty on the growth hormone response to standardized treadmill exercise and arginine-insulin in normal girls and boys. J Clin Endocrinol Metab. 1994;79(2):537–41.
- Molina S, Paoli M, Camacho N, Arata-Bellabarba G, Lanes R. Is testosterone and estrogen priming prior to clonidine useful in the evaluation of the growth hormone status of short peripubertal children? J Pediatr Endocrinol Metab. 2008;21(3):257–66.
- Agrawal V, Garcia JM. The macimorelin-stimulated growth hormone test for adult growth hormone deficiency diagnosis. Expert Rev Mol Diagn. 2014;14(6):647–54.
- Csákváry V, Muzsnai A, Raduk D, Chaychenko T, Damholt BB, Kelepouris N, et al. Pharmacokinetics and pharmacodynamics of macimorelin acetate (AEZS-130) in paediatric patients with suspected growth hormone deficiency (GHD). J Endocr Soc. 2021;5(Suppl 1):A682–A682.
- Roh S-G, Lee H-G, Phung LT, Hidari H. Characterization of growth hormone secretion to growth hormone-releasing peptide-2 in domestic animals - a review. Asian-Australasian J Anim Sci. 2002;15(5):757–66.
- Onuki T, Hiroaki T, Sawano K, Shibata N, Nyuzuki H, Ogawa Y, et al. Robust growth hormone responses to GH-releasing peptide 2 in adolescents. J Pediatr Endocrinol Metab. 2024;37(8):730–3.
- Chihara K, Shimatsu A, Hizuka N, Tanaka T, Seino Y, Katofor Y. A simple diagnostic test using GH-releasing peptide-2 in adult GH deficiency. Eur J Endocrinol. 2007;157(1):19–27.
- Suzuki S, Ruike Y, Ishiwata K, Naito K, Igarashi K, Ishida A, et al. LBODP006 clinical usefulness of the growth hormone-releasing peptide-2 test for hypothalamic-pituitary disorder. J Endocr Soc. 2022;6(Suppl 1):A39–40.
- Cohen P, Rogol AD, Howard CP, Bright GM, Kappelgaard A-M, Rosenfeld RG, et al. Insulin growth factor-based dosing of growth hormone therapy in children: A randomized, controlled study. J Clin Endocrinol Metab. 2007;92(7):2480–6.
- Baron J. Growth hormone therapy in childhood: Titration versus weight-based dosing? J Clin Endocrinol Metab. 2007;92(7):2436–8.
- Pasquino AM, Pucarelli I, Roggini M, Segni M. Adult height in short normal girls treated with gonadotropin-releasing hormone analogs and growth hormone. J Clin Endocrinol Metab. 2000;85(2):619–22.
- Reiter EO. A brief review of the addition of gonadotropin-releasing hormone agonists(GnRH-Ag) to growth hormone (GH) treatment of children with idiopathic growth hormone deficiency: Previously published studies from America. Mol Cell Endocrinol. 2006;254–255:221–5.
- Li S, Wang X, Zhao Y, Ji W, Mao J, Nie M, et al. Combined therapy with GnRH analogue and growth hormone increases adult height inchildren with short stature and normal pubertal onset. Endocrine. 2020;69(3):615–24.
- Faglia G, Arosio M, Porretti S. Delayed closure of epiphyseal cartilages induced by the aromatase inhibitor anastrozole. Would it help short children grow up? J Endocrinol Invest. 2000;23(11):721–3.
- Dunkel L, Wickman S. Novel treatment of delayed male puberty with aromatase inhibitors. Horm Res. 2002;57(Suppl 2):44–52.
- Zung A, Zadik Z. New approaches in the treatment of short stature. Harefuah. 2002;141(12):1059–65.
- Hero M, Norjavaara E, Dunkel L. Inhibition of estrogen biosynthesis with a potent aromatase inhibitor increases predicted adult height in boys with idiopathic short stature: A randomized controlled trial. J Clin Endocrinol Metab. 2005;90(12):6396–402.
- Wassenaar MJ, Dekkers OM, Pereira AM, Wit JM, Smit JW, Biermasz NR, et al. Impact of the exon 3-deleted growth hormone (GH) receptor polymorphism on baseline height and the growth response to recombinant human GH therapy in GH-deficient (GHD) and non-GHD children with short stature: A systematic review and meta-analysis. J Clin Endocrinol Metab. 2009;94(10):3721–30.
- Tauber M, Ester W, Auriol F, Molinas C, Fauvel J, Caliebe J, et al. GH responsiveness in a large multinational cohort of SGA children with short stature (NESTEGG) is related to the exon 3 GHR polymorphism. Clin Endocrinol. 2007;67(3):457–61.
- Braz AF, Costalonga EF, Montenegro LR, Trarbach EB, Antonini SR, Malaquias AC, et al. The interactive effect of GHR-exon 3 and -202 A/C IGFBP3 polymorphisms on rhGH responsiveness and treatment outcomes in patients with Turner syndrome. J Clin Endocrinol Metab. 2012;97(4):E671-7.
- Blum WF, Machinis K, Shavrikova EP, Keller A, Stobbe H, Pfaeffle RW, et al. The growth response to growth hormone (GH) treatment in children with isolated GH deficiency is independent of the presence of the exon 3-minus isoform of the GH receptor. J Clin Endocrinol Metab. 2006;91(10):4171–4.
- Carrascosa A, Esteban C, Espadero R, Fernández-Cancio M, Andaluz P, Clemente M, et al. The d3/fl-growth hormone (GH) receptor polymorphism does not influence the effect of GH treatment (66 microg/kg per day) or the spontaneous growth in short non-GH-deficient small-for-gestational-age children: Results from a two-year controlled prospective. J Clin Endocrinol Metab. 2006;91(9):3281–6.
- Galetaki DM, Merchant N, Dauber A. Novel therapies for growth disorders. Eur J Pediatr. 2024;183(3):1121–8.
- Choi HS, Kwon A, Suh J, Song K, Chae HW, Kim H-S. Effect of long-acting growth hormone treatment on endogenous growth hormone secretion in prepubertal patients with idiopathic short stature: A preliminary study. Growth Horm IGF Res. 2022;66:101486.
- Matustik MC, Furlanetto RW, Meyer WJ 3rd. Chronobiologic considerations in human growth hormone therapy. J Pediatr. 1983;103(4):543–6.
- Lal RA, Hoffman AR. Perspectives on long-acting growth hormone therapy in children and adults. Arch Endocrinol Metab. 2019;63(6):601–7.
- Grillo MS, Frank J, Saenger P. Long acting growth hormone (LAGH), an update. Front Pediatr. 2023;11:1254231.
- Höybye C, Pfeiffer AFH, Ferone D, Christiansen JS, Gilfoyle D, Christoffersen ED, et al. A phase 2 trial of long-acting TransCon growth hormone in adult GH deficiency. Endocr Connect. 2017;6(3):129–38.
- Thornton P, Hofman P, Maniatis A, Aghajanova E, Chertok E, Korpal-Szczyrska M, et al. OR17-4 Transcon growth hormone in the treatment of pediatric growth hormone deficiency: Results of the phase 3 height trial. J Endocr Soc. 2019;3(Suppl 1).
- Lamb YN. Lonapegsomatropin: Pediatric first approval. Paediatr Drugs. 2022 Jan;24(1):83–90.
- Miller BS, Yuen KCJ. Spotlight on lonapegsomatropin once-weekly injection and its potential in the treatment of growth hormone deficiency in pediatric patients. Drug Des Devel Ther. 2022;16:2055–66.
- Chatelain P, Malievskiy O, Radziuk K, Senatorova G, Abdou MO, Vlachopapadopoulou E, et al. A randomized phase 2 study of long-acting TransCon GH vs daily GH in childhood GH deficiency. J Clin Endocrinol Metab. 2017;102(5):1673–82.
- Campbell DL, Walsh KJ, Herz C, Karpf DB, Beckert M, Leff JA, et al. 016–TransCon GH as a long-acting growth hormone for the treatment of pediatric growth hormone deficiency. J Pediatr Nurs. 2019;46:133.
- Thornton PS, Maniatis AK, Aghajanova E, Chertok E, Vlachopapadopoulou E, Lin Z, et al. Weekly lonapegsomatropin in treatment-naïve children with growth hormonedeficiency: The Phase 3 heiGHt Trial. J Clin Endocrinol Metab. 2021;106(11):3184–95.
- Stochholm K, Kiess W. Long-term safety of growth hormone-A combined registry analysis. Clin Endocrinol (Oxf). 2018;88(4):515–28.
- Alkhatib EH, Dauber A, Estrada DE, Majidi S. Weekly growth hormone (lonapegsomatropin) causes severe transient hyperglycemia in a child with obesity. Vol. 96, Hormone Research in Paediatrics. Switzerland; 2023. p. 542–6.
- Maniatis AK, Carakushansky M, Galcheva S, Prakasam G, Fox LA, Dankovcikova A, et al. Treatment burden of weekly somatrogon vs daily somatropin in children with growth hormone deficiency: A randomized study. J Endocr Soc. 2022;6(10):bvac117.
- Maniatis AK, Casella SJ, Nadgir UM, Hofman PL, Saenger P, Chertock ED, et al. Safety and efficacy of lonapegsomatropin in children with growth hormone deficiency: enliGHten Trial 2-year results. J Clin Endocrinol Metab. 2022;107(7):e2680-e2689.
- Miller BS, Blair J, Horikawa R, Linglart A, Yuen KCJ. Developments in the management of growth hormone deficiency: Clinical utility of somapacitan. Drug Des Devel Ther. 2024;18:291–306.
- Helleberg H, Bjelke M, Damholt BB, Pedersen PJ, Rasmussen MH. Absorption, metabolism and excretion of once-weekly somapacitan, a long-acting growth hormone derivative, after single subcutaneous dosing in human subjects. Eur J Pharm Sci. 2021;167:106030.
- Ramírez-Andersen HS, Behrens C, Buchardt J, Fels JJ, Folkesson CG, Jianhe C, et al. Long-acting human growth hormone analogue by noncovalent albumin binding. Bioconjug Chem. 2018;29(9):3129–43.
- Savendahl LS, Rasmussen MH. SAT-LB12 Once-weekly somapacitan vs daily growth hormone in growth hormone deficiency: 2-year safety results from REAL 3, a randomized phase 2 trial. J Endocr Soc. 2020 8;4(Suppl 1).
- Savendahl L, Højby Rasmussen M, Horikawa R, Khadilkar V, Battelino T, Saenger P. SUN-247 Once-weekly somapacitan in childhood growth hormone deficiency: Efficacy and safety results of a randomized, open-label, controlled phase 2 trial (REAL 3). J Endocr Soc. 2019;3(Suppl 1).
- Sävendahl LS, Battelino T, Rasmussen MH, Horikawa R, Saenger P. Once-weekly somapacitan versus daily growth hormone in growth hormone deficiency: 2-year efficacy results from REAL 3, a Randomized Phase 2 Trial. J Endocr Soc. 2021;5(Suppl 1):A680–A680.
- Sävendahl L, Battelino T, Højby Rasmussen M, Brod M, Röhrich S, Saenger P, et al. Weekly somapacitan in GH deficiency: 4-year efficacy, safety, andtreatment/disease burden results from REAL 3. J Clin Endocrinol Metab. 2023;108(10):2569–78.
- Yuen KCJ. Utilizing somapacitan, a long-acting growth hormone formulation, for thetreatment of adult growth hormone deficiency: A guide for clinicians. Endocr Pract. 2024;30(10):1003–10.
- Takahashi Y, Biller BMK, Fukuoka H, Ho KKY, Rasmussen MH, Nedjatian N, et al. Weekly somapacitan had no adverse effects on glucose metabolism in adults withgrowth hormone deficiency. Pituitary. 2023;26(1):57–72.
- Fujio S, Makino R, Sugata J, Hanada T, Hanaya R. 6731 Initial therapeutic effects of weekly growth hormone replacement therapy: Somapacitan. J Endocr Soc. 2024;8(Suppl 1).
- Hershkovitz O, Bar-Ilan A, Guy R, Felikman Y, Moschcovich L, Hwa V, et al. In vitro and in vivo characterization of MOD-4023, a long-acting carboxy-terminal peptide (CTP)-modified human growth hormone. Mol Pharm. 2016;13(2):631–9.
- Velazquez EP, Miller BS, Yuen KCJ. Somatrogon injection for the treatment of pediatric growth hormone deficiency with comparison to other LAGH products. Expert Rev Endocrinol Metab. 2024;19(1):1–10.
- Valluri S, Pastrak A, Wajnrajch M, Silverman L, Cara J, Deal C. Somatrogon growth hormone in the treatment of pediatric growth hormone deficiency: Results of the Pivotal Pediatric Phase 3 Clinical Trial. 2022;
- Cara J, Carlsson M, Rosenfeld RG, Wajnrajch MP, Wang R, Zadik Z. RF26 | PSAT150 Growth outcomes from the Phase 2 and Phase 3 studies of once weekly somatrogon vs daily genotropin in pediatric patients with growth hormone deficiency: Comparisons with published literature and an international growth study database. J Endocr Soc. 2022;6(Suppl 1):A647–A647.
- Zadik Z, Zelinska N, Iotova V, Skorodok Y, Malievsky O, Mauras N, et al. An open-label extension of a phase 2 dose-finding study of once-weekly somatrogon vs. once-daily Genotropin in children with short stature due to growth hormone deficiency: Results following 5 years of treatment. J Pediatr Endocrinol Metab. 2023;36(3):261–9.
- Boguszewski MC da S, Boguszewski CL. Update on the use of long-acting growth hormone in children. Curr Opin Pediatr. 2024;36(4):437–41.
- Höybye C. Comparing treatment with daily and long-acting growth hormone formulations in adults with growth hormone deficiency: Challenging issues, benefits, and risks. Best Pract Res Clin Endocrinol Metab. 2023;37(6):101788.
- Maniatis A, Cutfield W, Dattani M, Deal C, Collett-Solberg PF, Horikawa R, et al. Long-acting growth hormone therapy in pediatric growth hormone deficiency: A consensus statement. J Clin Endocrinol Metab. 2024;1-9.
- Blethen SL, Allen DB, Graves D, August G, Moshang T, Rosenfeld R. Safety of recombinant deoxyribonucleic acid-derived growth hormone: The National Cooperative Growth Study Experience. J Clin Endocrinol Metab. 1996;81(5):1704–10.
- Tuffli GA, Johanson A, Rundle AC, Allen DB. Lack of increased risk for extracranial, nonleukemic neoplasms in recipients of recombinant deoxyribonucleic acid growth hormone. J Clin Endocrinol Metab. 1995;80(4):1416–22.
- Cowell CT, Dietsch S. Adverse events during growth hormone therapy. J Pediatr Endocrinol Metab. 1995;8:243–52.
- Clayton PE, Cowell CT. Safety issues in children and adolescents during growth hormone therapy-a review. Growth Horm & IGF Res. 2000;10:306–10.
- Bell J, Parker KL, Swinford RD, Hoffman AR, Maneatis T, Lippe B. Long-term safety of recombinant human growth hormone in children. J Clin Endocrinol Metab. 2010;95(1):167–77.
- Craig ME, Cowell CT, Larsson P, Zipf WB, Reiter EO, Albertsson Wikland K, et al. Growth hormone treatment and adverse events in Prader-Willi syndrome: Data from KIGS (the Pfizer International Growth Database). Clin Endocrinol (Oxf). 2006;65(2):178–85.
- Tauber M, Diene G, Molinas C, Hébert M. Review of 64 cases of death in children with Prader-Willi syndrome (PWS). Am J Med Genet A. 2008;146A(7):881–7.
- Eiholzer U. Deaths in children with Prader-Willi syndrome. A contribution to the debate aboutthe safety of growth hormone treatment in children with PWS. Horm Res. 2005;63(1):33–9.
- Gerard JM, Garibaldi L, Myers SE, Aceto TJ, Kotagal S, Gibbons VP, et al. Sleep apnea in patients receiving growth hormone. Clin Pediatr. 1997;36(6):321–6.
- Lindgren AC, Hellström LG, Ritzén EM, Milerad J. Growth hormone treatment increases CO(2) response, ventilation and centralinspiratory drive in children with Prader-Willi syndrome. Eur J Pediatr. 1999;158(11):936–40.
- Miller J, Silverstein J, Shuster J, Driscoll DJ, Wagner M. Short-term effects of growth hormone on sleep abnormalities in Prader-Willi syndrome. J Clin Endocrinol Metab. 2006;91(2):413–7.
- Bolar K, Hoffman AR, Maneatis T, Lippe B. Long-term safety of recombinant human growth hormone in Turner syndrome. J Clin Endocrinol Metab. 2008;93(2):344–51.
- Schoemaker MJ, Swerdlow AJ, Higgins CD, Wright AF, Jacobs PA, UK Clinical Cytogenetics Group. Cancer incidence in women with Turner syndrome in Great Britain: A national cohort study. Lancet Oncol. 2008;9(3):239–46.
- Carel J-C, Ecosse E, Landier F, Meguellati-Hakkas D, Kaguelidou F, Rey G, et al. Long-term mortality after recombinant growth hormone treatment for isolated growth hormone deficiency or childhood short stature: Preliminary report of the French SAGhE study. J Clin Endocrinol Metab. 2012;97(2):416–25.
- Sävendahl L, Maes M, Albertsson-Wikland K, Borgström B, Carel JC, Henrard S, et al. Long-term mortality and causes of death in isolated GHD, ISS, and SGA patients treated with recombinant growth hormone during childhood in Belgium, The Netherlands, and Sweden: Preliminary report of 3 countries participating in the EU SAGhE study. J Clin Endo Metab. 2012;97(2):213–7.
- Maneatis T, Baptista J, Connelly K, Blethen S. Growth hormone safety update from the National Cooperative Growth Study. J Pediatr Endocrinol Metab. 2000;13(Suppl 2):1035–44.
- Ergun-Longmire B, Mertens AC, Mitby P, Qin J, Heller G, Shi W, et al. Growth hormone treatment and risk of second neoplasms in the childhood cancer survivor. J Clin Endocrinol Metab. 2006;91(9):3494–8.
- Sklar CA, Mertens AC, Mitby P, Occhiogrosso G, Qin J, Heller G, et al. Risk of disease recurrence and second neoplasms in survivors of childhood cancer treated with growth hormone: A report from the Childhood Cancer Survivor Study. J Clin Endocrinol Metab. 2002;87(7):3136–41.
- Patterson BC, Chen Y, Sklar CA, Neglia J, Yasui Y, Mertens A, et al. Growth hormone exposure as a risk factor for the development of subsequent neoplasms of the central nervous system: A report from the Childhood Cancer Survivor Study. J Clin Endocrinol Metab. 2014;99(6):2030–7.
- Sklar CA, Antal Z, Chemaitilly W, Cohen LE, Follin C, Meacham LR, et al. Hypothalamic–pituitary and growth disorders in survivors of childhood cancer: An Endocrine Society Clinical Practice Guideline. J Clin Endocrinol Metab. 2018;103(8):2761-84.
- Child CJ, Zimmermann AG, Jia N, Robison LL, Brämswig JH, Blum WF. Assessment of primary cancer incidence in growth hormone-treated children: Comparison of a multinational prospective observational study with population databases. Horm Res Paediatr. 2016;85(3):198–206.
- Raman S, Grimberg A, Waguespack SG, Miller BS, Sklar CA, Meacham LR, et al. Risk of neoplasia in pediatric patients receiving growth hormone therapy--a report from the Pediatric Endocrine Society Drug and Therapeutics Committee. J Clin Endocrinol Metab. 2015;100(6):2192–203.
- Clayton PE, Cuneo RC, Juul A, Monson JP, Shalet SM, Tauber M. Consensus statement on the management of the GH-treated adolescent in the transition to adult care. Eur J Endocrinol. 2005;152(2):165–70.
- Molitch ME, Clemmons DR, Malozowski S, Merriam GR, Vance ML. Evaluation and treatment of adult growth hormone deficiency: An Endocrine Society Clinical Practice Guideline. J Clin Endocrinol Metab. 2011;96(6):1587–609.
- Tauber M, Moulin P, Pienkowski C, Jouret B, Rochiccioli P. Growth hormone (GH) retesting and auxological data in 131 GH-deficient patients after completion of treatment. J Clin Endocrinol Metab. 1997;82(2):352–6.
- Colao A, Di Somma C, Savastano S, Rota F, Savanelli MC, Aimaretti G, et al. A reappraisal of diagnosing GH deficiency in adults: Role of gender, age, waist circumference, and body mass index. J Clin Endocrinol Metab. 2009;94(11):4414–22.
- Cook DM, Rose SR. A review of guidelines for use of growth hormone in pediatric and transition patients. Pituitary. 2012;15(3):301–10.
- Quigley CA, Zagar AJ, Liu CC, Brown DM, Huseman C, Levitsky L, et al. United States multicenter study of factors predicting the persistence of GH deficiency during the transition period between childhood and adulthood. Int J Pediatr Endocrinol. 2013;2013(1):6.
- Stanley T. Diagnosis of growth hormone deficiency in childhood. Curr Opin Endocrinol Diabetes Obes. 2012;19(1):47–52.
- Hage C, Gan H-W, Ibba A, Patti G, Dattani M, Loche S, et al. Advances in differential diagnosis and management of growth hormone deficiency in children. Nat Rev Endocrinol. 2021;17(10):608–24.
- Ranke MB, Lindberg A, Albertsson-Wikland K, Wilton P, Price DA, Reiter EO. Increased response, but lower responsiveness, to growth hormone (GH) in very young children (aged 0-3 years) with idiopathic GH deficiency: Analysis of data from KIGS. J Clin Endocrinol Metab. 2005;90(4):1966–71.
- Stagi S, Scalini P, Farello G, Verrotti A. Possible effects of an early diagnosis and treatment in patients with growth hormone deficiency: The state of art. Ital J Pediatr. 2017;43(1):81.
- Boguszewski MCS. Growth hormone deficiency and replacement in children. Rev Endocr Metab Disord. 2021;22(1):101–8.
- Ross J, Fridman M, Kelepouris N, Murray K, Krone N, Polak M, et al. Factors associated with response to growth hormone in pediatric growth disorders: Results of a 5-year Registry Analysis. J Endocr Soc. 2023;7(5):bvad026.
- Yuen KCJ, Alter CA, Miller BS, Gannon AW, Tritos NA, Samson SL, et al. Adult growth hormone deficiency: Optimizing transition of care from pediatric to adult services. Growth Horm IGF Res. 2021;56:101375.
- Saenger PH, Mejia-Corletto J. Long-acting growth hormone: An update. Endocr Dev. 2016;30:79–97.
- Pampanini V, Deodati A, Inzaghi E, Cianfarani S. Long-acting growth hormone preparations and their use in children with growth hormone deficiency. Horm Res Paediatr. 2023;96(6):553–9.